We are looking for a curious and motivated new member of our team working on single-walled carbon nanotubes as one-dimensional semiconductors. The goal of this PhD project is to understand the many-body physics of excitons and trions in carbon nanotubes, i.e., the spectroscopic features (optical absorption/emission) of chemically, electrochemically and electrostatically doped carbon nanotubes at different carrier densities and temperatures.
The project is embedded in the Collaborative Research Centre “Isolated quantum systems and universality in extreme conditions” (SFB 1225, https://www.isoquant-heidelberg.de/) and the Institute for Physical Chemistry (https://www.pci.uni-heidelberg.de/en) in Heidelberg ensuring close collaboration between theorists and experimentalists.
More information can be found here.
DPG Press Release, November 14, 2024
“In recognition of his groundbreaking developments of Penning ion traps into spectroscopic precision measurement devices and their applications for testing the four fundamental forces, their symmetries, the fundamental constants, and thereby the Standard Model of particle physics.”
Klaus Blaum is being recognized for his groundbreaking advancements in Penning ion trap technology, which have significantly improved the precision of measurements of masses, charges, and magnetic moments of particles, antiparticles, and highly charged ions by up to two orders of magnitude. This enables the study of fundamental forces and interactions with an accuracy that rivals and complements the largest particle accelerators in the world.
Klaus Blaum studied physics at Johannes Gutenberg University Mainz, where he earned his PhD in 2000. After positions at GSI Darmstadt, CERN, and as a Helmholtz junior research group leader in Mainz, he was appointed Director of the Max Planck Institute for Nuclear Physics in Heidelberg in 2007. He has received numerous awards, including the Gustav Hertz and Otto Hahn Prizes, and has been awarded two ERC Advanced Grants.
The award will be presented in March 2025 during the 88th Annual Meeting of the DPG in Bonn.
The Stern-Gerlach Medal is the highest award of the DPG for outstanding achievements in experimental physics. It is given for work in any area of physics. The award consists of a gold medal featuring the portraits of Otto Stern and Walther Gerlach, as well as a certificate handwritten on parchment.
DPG Press Release, November 14, 2024
“In recognition of his groundbreaking developments of Penning ion traps into spectroscopic precision measurement devices and their applications for testing the four fundamental forces, their symmetries, the fundamental constants, and thereby the Standard Model of particle physics.”
Klaus Blaum is being recognized for his groundbreaking advancements in Penning ion trap technology, which have significantly improved the precision of measurements of masses, charges, and magnetic moments of particles, antiparticles, and highly charged ions by up to two orders of magnitude. This enables the study of fundamental forces and interactions with an accuracy that rivals and complements the largest particle accelerators in the world.
Klaus Blaum studied physics at Johannes Gutenberg University Mainz, where he earned his PhD in 2000. After positions at GSI Darmstadt, CERN, and as a Helmholtz junior research group leader in Mainz, he was appointed Director of the Max Planck Institute for Nuclear Physics in Heidelberg in 2007. He has received numerous awards, including the Gustav Hertz and Otto Hahn Prizes, and has been awarded two ERC Advanced Grants.
The award will be presented in March 2025 during the 88th Annual Meeting of the DPG in Bonn.
The Stern-Gerlach Medal is the highest award of the DPG for outstanding achievements in experimental physics. It is given for work in any area of physics. The award consists of a gold medal featuring the portraits of Otto Stern and Walther Gerlach, as well as a certificate handwritten on parchment.
DPG Pressemitteilung vom 14.11.2024
„In Würdigung seiner bahnbrechenden Entwicklungen der Penning-Ionenfallen zu spektroskopischen Präzisionsmessgeräten und deren Anwendungen für Tests der vier fundamentalen Wechselwirkungen, deren Symmetrien, der fundamentalen Konstanten und damit des Standardmodells der Teilchenphysik.“
Klaus Blaum wird für seine bahnbrechenden Weiterentwicklungen der Messtechnik an Penning-Ionenfallen gewürdigt, mit denen er die Genauigkeit der Messung von Massen, Ladungen und magnetischen Momenten von Teilchen, Antiteilchen und hochgeladenen Ionen um teilweise mehr als zwei Größenordnungen verbessert hat. Dies eröffnet die Möglichkeit, fundamentale Kräfte und Wechselwirkungen mit einer Genauigkeit zu untersuchen, die mit den größten Beschleunigeranlagen der Welt konkurriert und diese ergänzen kann.
Klaus Blaum studierte Physik an der Johannes Gutenberg-Universität Mainz und wurde dort im Jahr 2000 promoviert. Nach Stationen an der GSI-Darmstadt, am CERN und als Helmholtz-Nachwuchsgruppenleiter in Mainz wurde er 2007 als Direktor an das Max-Planck-Institut für Kernphysik in Heidelberg berufen. Er wurde u.a. mit dem Gustav-Hertz- und dem Otto-Hahn-Preis ausgezeichnet und erhielt zwei ERC Advanced Grants.
Die Auszeichnung wird im März 2025 während der 88. Jahrestagung der DPG in Bonn verliehen.
_______________________________________________________________________________
Die Stern-Gerlach-Medaille ist die höchste Auszeichnung der DPG für herausragende Leistungen auf dem Gebiet der experimentellen Physik. Sie wird für Arbeiten aus dem gesamten Bereich der Physik vergeben. Die Auszeichnung besteht aus einer goldenen Medaille mit den Porträts von Otto Stern und Walther Gerlach und einer auf Pergament handgeschriebenen Urkunde.
Pressemitteilung Universität Heidelberg Nr. 101/2024
10. September 2024
Fakultät für Physik und Astronomie lädt zu Aktions- und Vortragsprogramm ein – Angebote für Schulklassen und die interessierte Öffentlichkeit
Aus Anlass des 70-jährigen Bestehens des europäischen Forschungszentrums CERN – dem Sitz des weltweit leistungsfähigsten Teilchenbeschleunigers – lädt die Fakultät für Physik und Astronomie der Universität Heidelberg am 19. und 20. September 2024 zu einem umfangreichen Aktions- und Vortragsprogramm ein: Unter dem Motto „Kleine Teilchen, große Chancen: 70 Jahre CERN“ bietet es Einblicke in die Forschung zur Welt der kleinsten Teilchen und der Zusammensetzung unseres Universums. Dazu gehören ein Science Pub Quiz zur Teilchenphysik, eine Podiumsdiskussion sowie Vorträge von Heidelberger Physikerinnen und Physikern, die sich mit der Geschichte des CERN und den technologischen und physikalischen Highlights der CERN-Forschung beschäftigen. Eingebettet sind die für eine breite Öffentlichkeit konzipierten Veranstaltungen in ein Mitmach- und Besichtigungsprogramm, das sich an Schulklassen ab Jahrgangsstufe 7 sowie an interessierte Einzelpersonen wendet. Sämtliche Veranstaltungen finden im und am Kirchhoff-Institut für Physik, Im Neuenheimer Feld 227, statt.
Was ist das Universum und woraus besteht es? Zur Welt der kleinsten Teilchen und ihren Wechselwirkungen forschen Wissenschaftler aus der ganzen Welt seit sieben Jahrzehnten am CERN, der in der Nähe von Genf (Schweiz) gelegenen internationalen Forschungseinrichtung für Kern- und Teilchenphysik. Ihr Herz ist der Large Hadron Collider (LHC) – ein unterirdischer ringförmiger Teilchenbeschleuniger mit fast 27 Kilometern Umfang, in dem Protonen und Atomkerne gegenläufig auf nahezu Lichtgeschwindigkeit beschleunigt und zur Kollision gebracht werden. Dabei werden Zustände wie unmittelbar nach dem Urknall erreicht. Mithilfe spezieller Detektoren beobachten Forscherinnen und Forscher, welche Teilchen bei diesen Kollisionen entstehen. Maßgeblich an den Forschungsarbeiten beteiligt sind auch Physikerinnen und Physiker der Universität Heidelberg – so an den ALICE-, ATLAS- und LHCb-Experimenten. Ziel der Forschung ist es, aus den Teilchenkollisionen Informationen zu den bisher unbeantworteten physikalischen Fragen zur Zusammensetzung und der Entwicklung unseres Universums zu finden. Aus Anlass des 70-jährigen Bestehens der Forschungseinrichtung CERN – Conseil Européen pour la Recherche Nucléaire – beteiligt sich auch die Fakultät für Physik und Astronomie der Ruperto Carola an einem bundesweiten Aktionsprogramm. Initiiert hat die Heidelberger Veranstaltungen Prof. Dr. Stephanie Hansmann-Menzemer vom Physikalischen Institut, die Sprecherin des LHCb-Forschungsschwerpunktes ist.
Für ein Science Pub Quiz – ein Wissenschaftsquiz in „Kneipenatmosphäre“ – öffnet das Kirchhoff-Institut für Physik am Abend des 19. September seine Türen. Die interessierte Öffentlichkeit ist eingeladen, in Gruppen von bis zu sechs Personen in lockerer Runde an witzigen und interessanten Fragen rund um die Teilchenphysik und das CERN zu knobeln. Von ihren Erfahrungen in der CERN-Forschung berichten dabei mit Prof. Dr. Monica Dunford und Dr. Sara Celani zwei Heidelberger Physikerinnen, die an den LHC-Experimenten ATLAS und LHCb beteiligt sind. Prof. Dunford befasst sich mit Proton-Proton-Kollisionen bei sehr hohen Energien – mit der Suche nach neuen Phänomenen außerhalb des sogenannten Standardmodells der Elementarteilchenphysik; Dr. Celani erläutert die Unterschiede zwischen Materie und Antimaterie. Das Science Pub Quiz beginnt um 19 Uhr.
Drei Kurzvorträge mit anschließender Podiumsdiskussion stehen am Abend des 20. September auf dem Programm. Neben Prof. Dunford gewähren Prof. Dr. Hans-Christian Schultz-Coulon und Prof. Dr. Ulrich Uwer Einblicke in die Geschichte des CERN; sie sprechen über die Forschungseinrichtung als Technologieschmiede und berichten über Highlights der physikalischen Forschung. Die drei Wissenschaftler, die selbst an zentraler Stelle in den CERN-Kollaborationen eingebunden und damit hautnah bei den Experimenten mit dabei sind, beantworten im Anschluss Fragen aus dem Publikum. Durch den Abend führt als Moderatorin die Physikerin Dr. Angela Halfar. Die Vorträge mit anschließender Diskussionsrunde finden in Hörsaal 1 des Kirchhoff-Instituts für Physik statt, Beginn der Veranstaltung ist um 19 Uhr.
Eingebettet sind die beiden Abendveranstaltungen in ein Aktions- und Vortragsprogramm für Schulklassen ab Jahrgangsstufe 7, das am 19. und 20. September jeweils von 8 bis 14 Uhr stattfindet. Dazu gehören Kurzvorträge von Professorinnen und Professoren der Fakultät für Physik und Astronomie, die am CERN forschen. Sie nehmen die Schülerinnen und Schüler mit auf eine „Reise zum Urknall“, berichten über ein „kosmisches Antimaterierätsel“ oder erklären, worin sich Teilchen und Antiteilchen unterscheiden. Teil des Programms sind auch Führungen durch eine Ausstellung zur CERN-Forschung mit Experimenten zum Mitmachen sowie eine Augmented-Reality-Rallye, bei der computergestützt die großen LHC-Detektoren in Originalgröße dreidimensional zu bestaunen sind oder ein kosmischer Teilchenschauer sichtbar gemacht wird. Abgerundet wird das Aktionsprogramm durch die mobile Ausstellung „Urknall unterwegs“, die zu einer Zeitreise von den Anfängen unseres Universums bis in die Gegenwart einlädt.
Die Teilnahme an den Abendveranstaltungen ist kostenlos und ohne Anmeldung möglich. Das Programm für Schülerinnen und Schüler steht auch interessierten Einzelpersonen offen. Es besteht jeweils ganztägig die Möglichkeit, die CERN-Ausstellung im Foyer des Kirchhoff-Instituts für Physik und den „Urknall unterwegs“-Tunnel zu besuchen oder an der Augmented-Reality-Rallye über den Campus teilzunehmen. Für die Teilnahme von Schulklassen ist eine Anmeldung per Mail erforderlich an:
Press Release Heidelberg University No. 101/2024
September 10,2024
To celebrate the 70th anniversary of CERN – home to the world’s most powerful particle accelerator – the Faculty of Physics and Astronomy at Heidelberg University is hosting an extensive program of activities and lectures on September 19 and 20, 2024. Under the motto “Small Particles, Big Opportunities: 70 Years of CERN”, the program offers insights into research on the world of the smallest particles and the composition of our universe. Highlights include a science pub quiz on particle physics, a panel discussion, and lectures by Heidelberg physicists addressing CERN’s history and the technological and scientific milestones of its research. Designed for a broad audience, these events are embedded in a hands-on and tour program aimed at school classes from grade 7 upwards and interested individuals. All events will take place at and around the Kirchhoff Institute for Physics, located at Im Neuenheimer Feld 227.
What is the universe, and what is it made of?
For seven decades, scientists from around the globe have been investigating the smallest particles and their interactions at CERN, the international research center for nuclear and particle physics near Geneva, Switzerland. At its heart is the Large Hadron Collider (LHC) – a nearly 27-kilometer-long underground circular accelerator where protons and atomic nuclei are accelerated to nearly the speed of light and collided. These experiments recreate conditions similar to those immediately following the Big Bang. Special detectors allow researchers to observe the particles produced in these collisions. Physicists from Heidelberg University play a significant role in this research, contributing to experiments such as ALICE, ATLAS, and LHCb. The goal is to extract information from these collisions to address fundamental unanswered questions about the universe’s composition and evolution.
To mark CERN’s 70th anniversary (Conseil Européen pour la Recherche Nucléaire), the Faculty of Physics and Astronomy at Heidelberg University is participating in a nationwide program of activities. The Heidelberg events have been initiated by Prof. Dr. Stephanie Hansmann-Menzemer from the Physics Institute, who leads the LHCb research group.
Program Highlights:
- Science Pub Quiz:
On the evening of September 19, the Kirchhoff Institute for Physics will open its doors for a pub-style science quiz. The public is invited to form groups of up to six people to tackle fun and fascinating questions about particle physics and CERN in a relaxed setting.- Guest speakers: Prof. Dr. Monica Dunford and Dr. Sara Celani, Heidelberg physicists involved in the ATLAS and LHCb experiments, respectively. Prof. Dunford focuses on high-energy proton-proton collisions and the search for phenomena beyond the Standard Model of particle physics, while Dr. Celani explores the differences between matter and antimatter.
- Time: 7:00 PM.
- Lectures and Panel Discussion:
On the evening of September 20, three short lectures will be followed by a panel discussion featuring:- Prof. Dr. Monica Dunford, Prof. Dr. Hans-Christian Schultz-Coulon, and Prof. Dr. Ulrich Uwer, who will share insights into CERN’s history, its role as a technological powerhouse, and key achievements in physics research.
- The event will be moderated by Dr. Angela Halfar.
- Location: Lecture Hall 1, Kirchhoff Institute for Physics.
- Time: 7:00 PM.
- Daytime Program for Schools and Individuals:
From 8:00 AM to 2:00 PM on September 19 and 20, the program includes:- Short lectures by Heidelberg professors engaged in CERN research, offering a “journey to the Big Bang,” discussions on the “cosmic antimatter puzzle,” and explanations of particle-antiparticle differences.
- Guided tours of a CERN research exhibit featuring hands-on experiments.
- An Augmented Reality (AR) rally, showcasing full-scale 3D models of the LHC detectors and visualizations of cosmic particle showers.
- The mobile exhibition “Big Bang on the Move,” presenting a time travel from the universe’s origins to today.
General Information:
- Evening events are free and open to the public without prior registration.
- The daytime program is also open to interested individuals in addition to school classes. Visitors can explore the CERN exhibit in the Kirchhoff Institute foyer, the Big Bang on the Move tunnel, or participate in the AR rally throughout the day.
Press Release Heidelberg University No. 101/2024
September 10,2024
To celebrate the 70th anniversary of CERN – home to the world’s most powerful particle accelerator – the Faculty of Physics and Astronomy at Heidelberg University is hosting an extensive program of activities and lectures on September 19 and 20, 2024. Under the motto “Small Particles, Big Opportunities: 70 Years of CERN”, the program offers insights into research on the world of the smallest particles and the composition of our universe. Highlights include a science pub quiz on particle physics, a panel discussion, and lectures by Heidelberg physicists addressing CERN’s history and the technological and scientific milestones of its research. Designed for a broad audience, these events are embedded in a hands-on and tour program aimed at school classes from grade 7 upwards and interested individuals. All events will take place at and around the Kirchhoff Institute for Physics, located at Im Neuenheimer Feld 227.
What is the universe, and what is it made of?
For seven decades, scientists from around the globe have been investigating the smallest particles and their interactions at CERN, the international research center for nuclear and particle physics near Geneva, Switzerland. At its heart is the Large Hadron Collider (LHC) – a nearly 27-kilometer-long underground circular accelerator where protons and atomic nuclei are accelerated to nearly the speed of light and collided. These experiments recreate conditions similar to those immediately following the Big Bang. Special detectors allow researchers to observe the particles produced in these collisions. Physicists from Heidelberg University play a significant role in this research, contributing to experiments such as ALICE, ATLAS, and LHCb. The goal is to extract information from these collisions to address fundamental unanswered questions about the universe’s composition and evolution.
To mark CERN’s 70th anniversary (Conseil Européen pour la Recherche Nucléaire), the Faculty of Physics and Astronomy at Heidelberg University is participating in a nationwide program of activities. The Heidelberg events have been initiated by Prof. Dr. Stephanie Hansmann-Menzemer from the Physics Institute, who leads the LHCb research group.
Program Highlights:
- Science Pub Quiz:
On the evening of September 19, the Kirchhoff Institute for Physics will open its doors for a pub-style science quiz. The public is invited to form groups of up to six people to tackle fun and fascinating questions about particle physics and CERN in a relaxed setting.- Guest speakers: Prof. Dr. Monica Dunford and Dr. Sara Celani, Heidelberg physicists involved in the ATLAS and LHCb experiments, respectively. Prof. Dunford focuses on high-energy proton-proton collisions and the search for phenomena beyond the Standard Model of particle physics, while Dr. Celani explores the differences between matter and antimatter.
- Time: 7:00 PM.
- Lectures and Panel Discussion:
On the evening of September 20, three short lectures will be followed by a panel discussion featuring:- Prof. Dr. Monica Dunford, Prof. Dr. Hans-Christian Schultz-Coulon, and Prof. Dr. Ulrich Uwer, who will share insights into CERN’s history, its role as a technological powerhouse, and key achievements in physics research.
- The event will be moderated by Dr. Angela Halfar.
- Location: Lecture Hall 1, Kirchhoff Institute for Physics.
- Time: 7:00 PM.
- Daytime Program for Schools and Individuals:
From 8:00 AM to 2:00 PM on September 19 and 20, the program includes:- Short lectures by Heidelberg professors engaged in CERN research, offering a “journey to the Big Bang,” discussions on the “cosmic antimatter puzzle,” and explanations of particle-antiparticle differences.
- Guided tours of a CERN research exhibit featuring hands-on experiments.
- An Augmented Reality (AR) rally, showcasing full-scale 3D models of the LHC detectors and visualizations of cosmic particle showers.
- The mobile exhibition “Big Bang on the Move,” presenting a time travel from the universe’s origins to today.
General Information:
- Evening events are free and open to the public without prior registration.
- The daytime program is also open to interested individuals in addition to school classes. Visitors can explore the CERN exhibit in the Kirchhoff Institute foyer, the Big Bang on the Move tunnel, or participate in the AR rally throughout the day.
Abstract:
An important step towards a comprehensive understanding of far-from-equilibrium dynamics of quantum many-body systems is the identification of unifying features that are independent of microscopic details of the system. We experimentally observe such robust features in the magnetization relaxation dynamics of disordered Heisenberg XX, XXZ, and Ising Hamiltonians. We realize these Heisenberg spin models with tunable anisotropy parameter and power-law interactions in an ensemble of Rydberg atoms by encoding the spin in suitable Rydberg state combinations. We consistently observe stretched-exponential relaxation of magnetization for all considered spin models, collapsing onto a single curve after appropriate rescaling of time. This robust short-time relaxation behavior is explained by a perturbative treatment that exploits the strong disorder in pairwise couplings, which leads to a description in terms of approximately independent pairs of spins. In numerical simulations of small systems, we show that these pairs of spins constitute approximate local integrals of motion, which remain at least partially conserved on a timescale exceeding the duration of the relaxation dynamics of the magnetization.
T. Franz, S. Geier, C. Hainaut, A. Braemer, N. Thaicharoen, M. Hornung, E. Braun, M. Gärttner,
G. Zürn, M.Weidemüller, „Observation of anisotropy-independent magnetization dynamics
in spatially disordered Heisenberg spin systems“, Phys. Rev. Res. 6, 033131 (2024).
https://journals.aps.org/prresearch/abstract/10.1103/PhysRevResearch.6.033131
Related to Project A05
Abstract:
We apply a Local Discontinuous Galerkin discretisation to flow equations of the O(N)-model in the Local Potential Approximation. The improved stability is directly observed by solving the flow equation for various N and space-time dimensions d. A particular focus of this work is the numerical discretisation and its implementation. It is realised as a module within the high performance PDE framework DUNE. A preliminary version of the module is available on GitHub, but it is not submitted for publication as it is too early to be archived.
F. Ihssen, J. M. Pawlowski, F. R. Sattler, N. Wink, „Local discontinuous Galerkin for the functional
renormalisation group“, Comput. Phys. Commun. 300, 109182 (2024).
https://www.sciencedirect.com/science/article/pii/S001046552400105X
Related to Project A01, A02, C03
The CRC 1225 ISOQUANT is hiring! We’re looking for a proactive individual with a passion for science communication to join our team. This full-time position (39.5 hours/week) is available immediately and is limited until June 30, 2028.
For more details and to information on how to apply, see Academic staff member for science communication (m/f/d)
Press Release Max Planck Institute for Nuclear Physics Heidelberg
03 July 2024
More than 6000 interested visitors experienced exciting insights into the research at the institute
On 23 June, the Max Planck Institute for Nuclear Physics in Heidelberg opened its doors. More than 6000 interested visitors of all ages followed this invitation, including a gratifying number of children and young people. The almost 400 highly motivated employees from science and infrastructure offered the enthusiastic public extensive and diverse insights into the work of a modern research institute at more than 50 stations, in 30 lectures and on 500 metres of poster walls.
The hands-on experiments were very popular and some seminar rooms could barely hold the audience for the exciting presentations. Prof Dr Christoph H. Keitel, Managing Director of the MPIK, sums up the event very positively: “Our aim was to give the general public an insight into our fields of research. The staff prepared this in advance with great enthusiasm in such a way that it was not only informative, but above all exciting and easy to understand. ”
The CRC 1225 ISOQUANT participated with an interactive station on the theme “Welcome to the Quantum World.” Here, visitors could experience “Quantum Yoga” and put themselves into a state of superposition, being in two states simultaneously. At the game table, visitors could rediscover the classic game of Tic Tac Toe using quantum rules in “Quantum Tic Tac Toe.”.
Press Release Heidelberg University No. 61/2024
07.06.2024
Internationally acclaimed physicist honoured for her service to Ruperto Carola
Prof. Dr Johanna Stachel has left a lasting imprint on Heidelberg University with her work as an outstanding scientist, her commitment to university interests and the Faculty of Physics and Astronomy, and as a role model for many young researchers. Accordingly, she has been appointed Senior Professor distincta of Ruperto Carola. The university awards this life-long honorary title to excellent researchers and academic teachers who have also especially distinguished themselves through their all-round commitment to the university.
Johanna Stachel – an internationally acclaimed expert in the field of nuclear and particle physics – has “helped to shape both the development of her subject and that of the faculty and university with the same enthusiasm and equal engagement. She is, at all levels, an impressive scientist and university teacher,” underlined Prof. Dr Frauke Melchior, Rector of Heidelberg University. In 2021 Prof. Stachel was awarded the Cross of Merit 1st Class of the Order of Merit of the Federal Republic of Germany in recognition of her special service to German science.
For almost three decades, Prof. Stachel’s scientific research has taken place at the Institute for Physics of Heidelberg University. She studied physics and chemistry at ETH Zurich (Switzerland) and the University of Mainz, where she also obtained her doctorate in 1982. Then she spent thirteen years as professor at the State University of New York at Stony Brook (USA), before, in 1996, she was appointed to a professorship for experimental physics at Heidelberg University. The physicist’s special research interest is in quark-gluon plasma – a primeval state of matter which today can only be generated with the aid of particle accelerators such as the Large Hadron Collider (LHC).
Prof. Stachel has received numerous awards for her LHC research in the context of the ALICE collaboration at the European research centre CERN in Geneva (Switzerland) and her activities in the scientific arena. Examples are her various involvements in funding agencies for scientific research, membership of high-level scientific academies and societies, and contributions as a reviewer and consultant for national and international scientific research institutions. From 2012 to 2014 she was the first woman to head the German Physical Society (DPG), followed by a two-year term as vice-president.
Heidelberg University has so far awarded the honorary title of Senior Professor distinctus to Konrad Beyreuther, Hermann Bujard (1934–2020), Detlef Junker, Paul Kirchhof, Andreas Kruse, Peter Meusburger (1942–2017), Thomas Rausch, Jochen Tröger and Jürgen Wolfrum.
Pressemitteilung Nr. 61/2024
07.06.2024
International ausgewiesene Physikerin erhält Ehrenbezeichnung für ihre Verdienste um die Ruperto Carola
Mit ihrem Wirken als herausragende Wissenschaftlerin, ihrem Einsatz für universitäre Belange ebenso wie für die Fakultät für Physik und Astronomie und ihrer Vorbildrolle für viele junge Forscherinnen und Forscher hat Prof. Dr. Johanna Stachel die Universität Heidelberg nachhaltig geprägt. Dafür ist sie zur Seniorprofessorin distincta der Ruperto Carola ernannt worden. Die Universität vergibt diese Ehrenbezeichnung auf Lebenszeit an ausgezeichnete Forscher und akademische Lehrer, die sich auch durch ihr gesamtuniversitäres Engagement um die Hochschule in besonderer Weise verdient gemacht haben.
Johanna Stachel – international ausgewiesene Expertin auf dem Gebiet der Kern- und Teilchenphysik – hat mit „der gleichen Begeisterung und dem gleichen Engagement sowohl die Geschicke ihres Faches als auch die der Fakultät und der Universität mitgestaltet. Sie ist eine auf allen Ebenen beeindruckende Wissenschaftlerin und Universitätslehrerin“, betont die Rektorin der Universität Heidelberg, Prof. Dr. Frauke Melchior. In Anerkennung ihrer besonderen Verdienste um die deutsche Wissenschaft wurde Prof. Stachel im Jahr 2021 mit dem Verdienstkreuz 1. Klasse des Verdienstordens der Bundesrepublik Deutschland ausgezeichnet.
Prof. Stachel forscht seit annähernd drei Jahrzehnten als Wissenschaftlerin am Physikalischen Institut der Universität Heidelberg. Sie studierte Physik und Chemie an der Eidgenössischen Technischen Hochschule Zürich (Schweiz) und an der Universität Mainz, an der sie 1982 auch promoviert wurde. Im Anschluss daran war sie dreizehn Jahre als Professorin in den USA an der State University of New York at Stony Brook tätig, ehe sie 1996 auf eine Professur für Experimentalphysik an die Universität Heidelberg berufen wurde. Das spezielle Forschungsinteresse der Physikerin gilt dem sogenannten Quark-Gluon-Plasma – einem Urzustand von Materie, der heute nur mithilfe von Teilchenbeschleunigern wie dem Large Hadron Collider (LHC) erzeugt werden kann.
Für ihre LHC-Forschung im Rahmen der ALICE-Kollaboration am europäischen Forschungszentrum CERN in Genf (Schweiz) und ihren Einsatz in der Wissenschaftslandschaft wurde sie vielfach ausgezeichnet, so für ihr vielfältiges Engagement in Einrichtungen der Wissenschaftsförderung ebenso wie als Mitglied in hochrangigen wissenschaftlichen Akademien und Gesellschaften sowie als Gutachterin und Beraterin in nationalen und internationalen wissenschaftlichen Forschungseinrichtungen. Von 2012 bis 2014 stand sie – als erste Frau im Präsidentenamt – an der Spitze der Deutschen Physikalischen Gesellschaft (DPG), gefolgt von einer zweijährigen Amtszeit als Vizepräsidentin.
Die lebenslange Ehrenbezeichnung eines Seniorprofessors distinctus hat die Universität Heidelberg bislang vergeben an Konrad Beyreuther, Hermann Bujard (1934 bis 2020), Detlef Junker, Paul Kirchhof, Andreas Kruse, Peter Meusburger (1942 bis 2017), Thomas Rausch, Jochen Tröger und Jürgen Wolfrum.
Press Release Heidelberg University No. 61/2024
07.06.2024
Internationally acclaimed physicist honoured for her service to Ruperto Carola
Prof. Dr Johanna Stachel has left a lasting imprint on Heidelberg University with her work as an outstanding scientist, her commitment to university interests and the Faculty of Physics and Astronomy, and as a role model for many young researchers. Accordingly, she has been appointed Senior Professor distincta of Ruperto Carola. The university awards this life-long honorary title to excellent researchers and academic teachers who have also especially distinguished themselves through their all-round commitment to the university.
Johanna Stachel – an internationally acclaimed expert in the field of nuclear and particle physics – has “helped to shape both the development of her subject and that of the faculty and university with the same enthusiasm and equal engagement. She is, at all levels, an impressive scientist and university teacher,” underlined Prof. Dr Frauke Melchior, Rector of Heidelberg University. In 2021 Prof. Stachel was awarded the Cross of Merit 1st Class of the Order of Merit of the Federal Republic of Germany in recognition of her special service to German science.
For almost three decades, Prof. Stachel’s scientific research has taken place at the Institute for Physics of Heidelberg University. She studied physics and chemistry at ETH Zurich (Switzerland) and the University of Mainz, where she also obtained her doctorate in 1982. Then she spent thirteen years as professor at the State University of New York at Stony Brook (USA), before, in 1996, she was appointed to a professorship for experimental physics at Heidelberg University. The physicist’s special research interest is in quark-gluon plasma – a primeval state of matter which today can only be generated with the aid of particle accelerators such as the Large Hadron Collider (LHC).
Prof. Stachel has received numerous awards for her LHC research in the context of the ALICE collaboration at the European research centre CERN in Geneva (Switzerland) and her activities in the scientific arena. Examples are her various involvements in funding agencies for scientific research, membership of high-level scientific academies and societies, and contributions as a reviewer and consultant for national and international scientific research institutions. From 2012 to 2014 she was the first woman to head the German Physical Society (DPG), followed by a two-year term as vice-president.
Heidelberg University has so far awarded the honorary title of Senior Professor distinctus to Konrad Beyreuther, Hermann Bujard (1934–2020), Detlef Junker, Paul Kirchhof, Andreas Kruse, Peter Meusburger (1942–2017), Thomas Rausch, Jochen Tröger and Jürgen Wolfrum.
Abstract:
Motivated by the wide range of kinematics covered by current and planned deep-inelastic scattering (DIS) facilities, we revisit the formalism, practical implementation, and numerical impact of target mass corrections (TMCs) for DIS on unpolarized nuclear targets. An important aspect is that we only use nuclear and later partonic degrees of freedom, carefully avoiding a picture of the nucleus in terms of nucleons. After establishing that formulae used for individual nucleon targets (p, n), derived in the Operator Product Expansion (OPE) formalism, are indeed applicable to nuclear targets, we rewrite expressions for nuclear TMCs in terms of re-scaled (or averaged) kinematic variables. As a consequence, we find a representation for nuclear TMCs that is approximately independent of the nuclear target. We go on to construct a single-parameter fit for all nuclear targets that is in good numerical agreement with full computations of TMCs. We discuss in detail qualitative and quantitative differences between nuclear TMCs built in the OPE and the parton model formalisms, as well as give numerical predictions for current and future facilities.
R. Ruiz, K. F. Muzakka, C. Leger, P. Risse, A. Accardi, P. Duwentäster, T. J. Hobbs, T. Jeo, C.
Keppel, M. Klasen, K. Kovaík, A. Kusina, J. G. Morfín, F. I. Olness, J. F. Owens, I. Schienbein,
J. Y. Yu, „Target mass corrections in lepton – nucleus DIS: Theory and applications to nuclear
PDFs“, Prog. Part. Nucl. Phys. 136, 104096 (2024).
https://www.sciencedirect.com/science/article/abs/pii/S0146641023000777
Related to Project C05
In 2016, the UN declared 11 February as International Day of Women and Girls in Science. A significant gender gap has persisted throughout the years at all levels of science, technology, engineering and mathematics (STEM) disciplines all over the world. Even though women have made tremendous progress towards increasing their participation in higher education, they are still under-represented in these fields.
Our Collaborative Research Center unifies researchers from diverse fields with a common shared interest to investigate isolated quantum systems and universality in extreme conditions. For our success it is of great importance that we foster an inclusive working environment, where everyone is treated respectfully. We believe science is a diverse endeavor: diversity provides new impulses, ideas, perspectives, and innovation. Gender equality has always been a core issue for our Collaborative Research Centre. Therefore, we are celebrating International Day of Women and Girls in Science, using the occasion to emphasize our welcome of all members of CRC ISO QUANT, irrespective of their gender.
Pressemitteilung Universität Heidelberg
08.02.2024
Physiker aus Heidelberg, den Niederlanden und der Schweiz sowie den USA ermitteln neuen Wert für die Neutronenhaut von Blei-208
Messdaten aus Kollisionen schwerer Ionen können Aufschluss über die Neutronenverteilung in Atomkernen geben. Das hat ein internationales Forschungsteam unter der Leitung von Dr. Giuliano Giacalone vom Institut für Theoretische Physik der Universität Heidelberg nachgewiesen. Die Wissenschaftler verwendeten Daten aus Teilchenkollisionen im Large Hadron Collider des europäischen Forschungszentrums CERN in Genf (Schweiz), um daraus die Neutronenhaut des Blei-Isotopen 208 zu ermitteln. Nach Angaben von Dr. Giacalone ermöglicht es dieser neue Forschungsansatz, die Verteilung von Neutronen in Atomkernen systematisch zu bestimmen.
Protonen und Neutronen, die Bausteine von Atomkernen, interagieren mittels der Starken Wechselwirkung, auch bekannt als Kernkraft. Dabei handelt es sich um eine der vier Grundkräfte im Standardmodell der Teilchenphysik, auf die eine Vielzahl von Phänomenen zurückgeht – von der Form der Atomkerne bis zu den Eigenschaften der exotischen Materie, aus der Neutronensterne bestehen. Diese Wechselwirkungen mithilfe fundamentaler Theorie zu berechnen, ist sehr schwierig, wie Dr. Giacalone erläutert. Während die Wissenschaft eine gute Vorstellung davon hat, wie die Protonen in Atomkernen verteilt sind, ist es ungleich schwerer, die Verteilung der Neutronen zu bestimmen. Aufschluss darüber kann die Dicke der sogenannten Neutronenhaut geben, eine Hülle aus überschüssigen Neutronen, die sich an der Oberfläche von besonders schweren Atomkernen wie Blei-208 ansammelt.
„Indem wir die Ausdehnung der Neutronenhaut bestimmen, wollen wir zu einem besseren Verständnis neutronenreicher Materie und der physikalischen Kräfte gelangen, denen sie unterliegt“, erklärt Dr. Govert Nijs vom Massachusetts Institute of Technology (USA), einer der Kooperationspartner in dem internationalen Forschungsteam. Blei-208 eignet sich besonders gut für solche experimentellen Untersuchungen, weil es reich an Neutronen ist, einen einfachen sphärischen Aufbau hat und in der Natur leicht zu finden ist. Frühere Experimente haben bereits einen bestimmten Wert für die Ausdehnung dieser Hülle aus überschüssigen Neutronen ergeben, eine unabhängige Bestimmung aus Experimenten in Teilchenbeschleunigern gab es bisher jedoch nicht. Mit den Datensätzen aus den Kollisionen von Blei-Ionen konnten die Wissenschaftler nun einen Vergleichswert schaffen, der auf den starken Wechselwirkungen von Quarks und Gluonen bei hohen Energien beruht.
Im weltgrößten Teilchenbeschleuniger LHC werden Blei-208-Kerne gezielt zur Kollision gebracht, um das sogenannte Quark-Gluon-Plasma zu reproduzieren. Dabei handelt es sich um einen Urzustand von Materie, in dem sich Quarks und Gluonen, zwei Arten von Elementarteilchen, frei bewegen, ungefähr so wie einige Momente nach dem Urknall. Die Neutronenhaut von Blei-208 beeinflusst nicht nur die Kollisionsrate der Partikel, sondern auch die Anzahl, den durchschnittlichen Impuls und die Verteilungswinkel der durch die Kollision entstehenden neuen Teilchen. Mithilfe der Bayesschen Inferenz – eine Methode zur Ermittlung von theoretischen Modellparametern bei gegebenen experimentellen Beobachtungen – gelang es den Physikern, Rückschlüsse auf den Gesamtradius des Atomkerns sowie auf die Radien der Protonen- und Neutronenverteilung zu ziehen. Aus ihrer Differenz ergibt sich für die Neutronenhaut von Blei-208 ein Wert von 0,217±0,058 Femtometern.
„Unser Ergebnis beruht ausschließlich auf bereits existierenden Datensätzen; somit kann es in Zukunft verbessert werden und ernsthaft mit anderen experimentellen Befunden konkurrieren“, sagt Dr. Wilke van der Schee, der an der Universität Utrecht (Niederlande) und am Forschungszentrum CERN tätig ist. Künftige Messungen von Observablen, die einen genaueren Blick auf die Neutronenverteilungen bieten, könnten dazu beitragen, die Genauigkeit der Bestimmung der Neutronenhaut anhand der LHC-Daten zu verbessern. Davon erhoffen sich die Wissenschaftler nicht nur ein besseres Verständnis neutronenreicher Materie, sondern indirekt auch Einblicke in das Verhalten der Starken Wechselwirkung.
Auf Heidelberger Seite waren die Arbeiten Teil des von der Deutschen Forschungsgemeinschaft geförderten Sonderforschungsbereich 1225 „Isolierte Quantensysteme und Universalität unter extremen Bedingungen“ (ISOQUANT). Die Forschungsergebnisse wurden in der Fachzeitschrift „Physical Review Letters“ veröffentlicht.
Originalpublikation
G. Giacalone, G. Nijs, W. van der Schee: Determination of the Neutron Skin of 208Pb from Ultrarelativistic Nuclear Collisions. Physical Review Letters 131, 202302 (15 November 2023).
DOI: 10.1103/PhysRevLett.131.202302
Kontakt
Dr. Giuliano Giacalone
Institut für Theoretische Physik
Philosophenweg 16, 69120 Heidelberg
giacalone@thphys.uni-heidelberg.de
Press Release Heidelberg University
08.02.2024
Physicists from Heidelberg, the Netherlands and Switzerland, and the USA establish new value for the neutron skin of lead-208
Measurement data from collisions of heavy ions can provide insight into the neutron distribution in atomic nuclei, according to an international research team led by Dr Giuliano Giacalone from the Institute for Theoretical Physics of Heidelberg University. The researchers used data from particle collisions in the Large Hadron Collider (LHC) at the European research centre CERN in Geneva (Switzerland) to determine the neutron skin of lead-208 isotopes. According to Dr Giacalone, this new experimental research approach makes it possible to systematically determine the distribution of neutrons in atomic nuclei.
Protons and neutrons, the building blocks of atomic nuclei, interact through the strong interaction, also known as the nuclear force. It is one of the four fundamental forces in the Standard Model of particle physics and is at the origin of a wealth of phenomena, from the shape of nuclei to the properties of exotic matter that makes up neutron stars. Calculating these interactions from fundamental theory is a very challenging task, as Dr Giacalone explains. While scientists have a good idea of how the protons in nuclei are distributed, it is far more difficult to determine the distribution of neutrons. Information can be gained from the thickness of the neutron skin, a shell of excess neutrons that collect on the surface of especially heavy atomic nuclei like lead-208.
“By determining the extent of the neutron skin, we hope to obtain a better understanding of neutron-rich matter and the physical forces acting on it,” explains Dr Govert Nijs of the Massachusetts Institute of Technology (USA), one of the cooperation partners in the international research team. Lead-208 is especially well-suited for such experimental searches because it is rich in neutrons, has a simple spherical structure, and is readily available in nature. Earlier experiments have already yielded a specific value for the extent of this shell of excess neutrons, yet an independent determination coming from a particle collider experiment did not exist. Using the data sets from the collisions of lead ions, the researchers were able to achieve such a determination and yield a new comparison value in processes based on the strong interactions of quarks and gluons at high energies.
In the LHC, the world’s largest particle accelerator, lead-208 nuclei are brought into collision to reproduce what is known as the quark-gluon plasma, a primordial state of matter in which quarks and gluons, two types of elementary particles, move freely as they did moments after the Big Bang. The neutron skin of lead-208 not only influences the collision rate of particles but also the number, the average momenta, and the angular distribution of the new particles produced by the collision. Using Bayesian inference – a method to determine theoretical model parameters for a given set of experimental observations – the physicists were able to draw conclusions about the total radius of the nucleus as well as the radii of the proton and neutron distributions. The result of their difference produces a value for the neutron skin of lead-208 of 0.217±0.058 femtometres.
“Our results are based solely on existing data sets, such that they can be improved in the future and challenge more seriously other experimental findings,” states Dr Wilke van Schee of Utrecht University (Netherlands) and CERN. Future measurements of observables that offer a closer view of the neutron distributions could help improve the precision of the neutron skin determination from the LHC data. The researchers not only hope to gain a better understanding of neutron-rich matter but also to obtain, indirectly, insight into the behaviour of the strong interaction.
The research in Heidelberg was done under the auspices of Collaborative Research Centre 1225, “Isolated quantum systems and universality under extreme conditions” (ISOQUANT). The research results were published in the journal “Physical Review Letters”.
Original publication:
G. Giacalone, G. Nijs, W. van der Schee: Determination of the Neutron Skin of 208Pb from Ultrarelativistic Nuclear Collisions. Physical Review Letters 131, 202302 (15 November 2023).
DOI: 10.1103/PhysRevLett.131.202302
Contact
Dr. Giuliano Giacalone
Institut für Theoretische Physik
giacalone@thphys.uni-heidelberg.de
Press Release Heidelberg University
08.02.2024
Physicists from Heidelberg, the Netherlands and Switzerland, and the USA establish new value for the neutron skin of lead-208
Measurement data from collisions of heavy ions can provide insight into the neutron distribution in atomic nuclei, according to an international research team led by Dr Giuliano Giacalone from the Institute for Theoretical Physics of Heidelberg University. The researchers used data from particle collisions in the Large Hadron Collider (LHC) at the European research centre CERN in Geneva (Switzerland) to determine the neutron skin of lead-208 isotopes. According to Dr Giacalone, this new experimental research approach makes it possible to systematically determine the distribution of neutrons in atomic nuclei.
Protons and neutrons, the building blocks of atomic nuclei, interact through the strong interaction, also known as the nuclear force. It is one of the four fundamental forces in the Standard Model of particle physics and is at the origin of a wealth of phenomena, from the shape of nuclei to the properties of exotic matter that makes up neutron stars. Calculating these interactions from fundamental theory is a very challenging task, as Dr Giacalone explains. While scientists have a good idea of how the protons in nuclei are distributed, it is far more difficult to determine the distribution of neutrons. Information can be gained from the thickness of the neutron skin, a shell of excess neutrons that collect on the surface of especially heavy atomic nuclei like lead-208.
“By determining the extent of the neutron skin, we hope to obtain a better understanding of neutron-rich matter and the physical forces acting on it,” explains Dr Govert Nijs of the Massachusetts Institute of Technology (USA), one of the cooperation partners in the international research team. Lead-208 is especially well-suited for such experimental searches because it is rich in neutrons, has a simple spherical structure, and is readily available in nature. Earlier experiments have already yielded a specific value for the extent of this shell of excess neutrons, yet an independent determination coming from a particle collider experiment did not exist. Using the data sets from the collisions of lead ions, the researchers were able to achieve such a determination and yield a new comparison value in processes based on the strong interactions of quarks and gluons at high energies.
In the LHC, the world’s largest particle accelerator, lead-208 nuclei are brought into collision to reproduce what is known as the quark-gluon plasma, a primordial state of matter in which quarks and gluons, two types of elementary particles, move freely as they did moments after the Big Bang. The neutron skin of lead-208 not only influences the collision rate of particles but also the number, the average momenta, and the angular distribution of the new particles produced by the collision. Using Bayesian inference – a method to determine theoretical model parameters for a given set of experimental observations – the physicists were able to draw conclusions about the total radius of the nucleus as well as the radii of the proton and neutron distributions. The result of their difference produces a value for the neutron skin of lead-208 of 0.217±0.058 femtometres.
“Our results are based solely on existing data sets, such that they can be improved in the future and challenge more seriously other experimental findings,” states Dr Wilke van Schee of Utrecht University (Netherlands) and CERN. Future measurements of observables that offer a closer view of the neutron distributions could help improve the precision of the neutron skin determination from the LHC data. The researchers not only hope to gain a better understanding of neutron-rich matter but also to obtain, indirectly, insight into the behaviour of the strong interaction.
The research in Heidelberg was done under the auspices of Collaborative Research Centre 1225, “Isolated quantum systems and universality under extreme conditions” (ISOQUANT). The research results were published in the journal “Physical Review Letters”.
Original publication:
G. Giacalone, G. Nijs, W. van der Schee: Determination of the Neutron Skin of 208Pb from Ultrarelativistic Nuclear Collisions. Physical Review Letters 131, 202302 (15 November 2023).
DOI: 10.1103/PhysRevLett.131.202302
Contact
Dr. Giuliano Giacalone
Institut für Theoretische Physik
giacalone@thphys.uni-heidelberg.de
Abstract:
Variational quantum algorithms offer fascinating prospects for the solution of combinatorial optimization problems using digital quantum computers. However, the achievable performance in such algorithms and the role of quantum correlations therein remain unclear. Here, we shed light on this open issue by establishing a tight connection to the seemingly unrelated field of quantum metrology: Metrological applications employ quantum states of spin ensembles with a reduced variance to achieve an increased sensitivity, and we cast the generation of such squeezed states in the form of finding optimal solutions to a combinatorial MaxCut problem with an increased precision. By solving this optimization problem with a quantum approximate optimization algorithm (QAOA), we show numerically as well as on an IBM Quantum chip how highly squeezed states are generated in a systematic procedure that can be adapted to a wide variety of quantum machines. Moreover, squeezing tailored for the QAOA of the MaxCut permits us to propose a figure of merit for future hardware benchmarks. Exploiting the connection, we show how the performance can be improved by warm-starting the optimization algorithm with the squeezed state.
G. C. Santra, F. Jendrzejewski, P. Hauke, D. J. Egger, „Squeezing and quantum approximate
optimization“, Phys. Rev. A 109, 012413 (2024).
https://journals.aps.org/pra/abstract/10.1103/PhysRevA.109.012413
Related to Project B04
Pressemitteilung Nr. 122/2023
21. November 2023
Sonderforschungsbereich ISOQUANT zeigt Werke der ukrainisch-niederländischen Künstlerin Alexandra Hunts
Quantenzustände zwischen Ordnung und Unordnung als Analogie zur Fragilität politischer Systeme sind Thema einer Ausstellung an der Universität Heidelberg, die Wissenschaft und Kunst verbindet. Sie zeigt Installationen, die die ukrainisch-niederländische Künstlerin Alexandra Hunts im Rahmen einer Science & Art Collaboration am Sonderforschungsbereich „Isolierte Quantensysteme und Universalität unter extremen Bedingungen“ (SFB 1225 ISOQUANT) geschaffen hat. Die künstlerischen Arbeiten, darunter eine mehrere Meter lange Stickerei auf Stoff, sind inspiriert von dem Dialog mit den Heidelberger Forscherinnen und Forschern. Die Ausstellung „many-body problem“ wird am Donnerstag, 23. November 2023, im European Institute for Neuromorphic Computing (EINC) auf dem Campus Im Neuenheimer Feld eröffnet und ist dann im EINC sowie im Gebäude des Instituts für Theoretische Physik am Philosophenweg bis zum 21. Dezember zu sehen.
Als Artist-in-Residence verbrachte Alexandra Hunts zwischen Februar und Juli 2022 drei Monate in Heidelberg, um Einblicke in die Forschungstätigkeiten der Mitglieder am SFB 1225 ISOQUANT zu erhalten und im Rahmen dieser SciArt Residency neue Perspektiven an der Schnittstelle von Wissenschaft und Kunst zu erschließen. Mit Blick auf die Entwicklungen in ihrem Heimatland Ukraine beschloss die Künstlerin, wechselnde Quantenzustände und die sich wandelnden Zustände politischer Systeme zum Thema ihrer Installationen zu machen. Die neun Meter lange Stickerei auf altem sowjetischem Leinen wurde in der Ukraine hergestellt. Die gestickten Ornamente sind das Ergebnis eines gemeinsam mit Alexandra Hunts entwickelten Computerprogramms, mit dem sich verschiedene Arten von Lawinenabgängen simulieren lassen. In einem weiteren Kunstwerk erinnert die stetige Rotation zweier Glasskulpturen, gefüllt mit Weizenernte aus der Ukraine, an komplexe Stundengläser.
Alexandra Hunts wurde 1990 in Lemberg (Ukraine) geboren. Sie erwarb einen Bachelor-Abschluss an der niederländischen Königlichen Kunstakademie in Den Haag und einen Master-Abschluss an der Kunstakademie Malmö (Schweden). Derzeit ist sie Stipendiatin der Rijksakademie van beeldende kunsten in Amsterdam (Niederlande). Alexandra Hunts arbeitet mit verschiedenen Medien, darunter Fotografie, Video, Installation und Skulptur. Ihre Arbeiten wurden unter anderem in Norwegen, den Niederlanden und in Dänemark ausgestellt; sie befinden sich in öffentlichen und privaten Sammlungen. Alexandra Hunts lebt und arbeitet in Amsterdam.
In Anwesenheit der Künstlerin wird die Ausstellung am 23. November eröffnet. Zur Begrüßung und Einführung sprechen Prof. Dr. Jürgen Berges, Sprecher des SFB 1225 ISOQUANT, sowie Wissenschaftskommunikatorin Dr. Lisa Ringena und Koordinatorin Tina Kuka, die ebenfalls am Sonderforschungsbereich tätig sind. Die Ausstellungseröffnung findet im Foyer des European Institute for Neuromorphic Computing, Im Neuenheimer Feld 225a, statt und beginnt um 19.00 Uhr. Die Installationen im EINC und im Institut für Theoretische Physik, Philosophenweg 12, können bis zum 21. Dezember wochentags zwischen 10 und 17 Uhr besichtigt werden.
Kontakt
Dr. Lisa Ringena
SFB1225 ISOQUNAT
Institut für Theoretische Physik
Philosophenweg 12
69120 Heidelberg
Tel.: +49 6221 54 9458
l.ringena@thphys.uni-heidelberg.de
Our Collaborative Research Center unifies researchers from diverse fields with a common shared interest to investigate isolated quantum systems and universality in extreme conditions. For our success it is of great importance that we foster an inclusive working environment, where everyone is treated respectfully. We believe science is a diverse endeavor: diversity provides new impulses, ideas, perspectives, and innovation.
Today (November 18) is the International Day of LGBTQIA+ People in STEM. The date is symbolic of the 60th anniversary of American Astronomer and gay activist Frank Kameny’s US Supreme Court fight against workplace discrimination. We would like to use this occasion to emphasize our welcome of all members of CRC ISOQUANT, irrespective of their gender or sexual identity.
Abstract:
Phase transitions in a non-perturbative regime can be studied by ab initio Lattice Field Theory methods. The status and future research directions for LFT investigations of Quantum Chromo-Dynamics under extreme conditions are reviewed, including properties of hadrons and of the hypothesized QCD axion as inferred from QCD topology in different phases. We discuss phase transitions in strong interactions in an extended parameter space, and the possibility of model building for Dark Matter and Electro-Weak Symmetry Breaking. Methodological challenges are addressed as well, including new developments in Artificial Intelligence geared towards the identification of different phases and transitions.
G. Aarts et al., “Phase transition in particle physics: Results and perspective from lattice Quantum Chromodynamics”, Prog. Part. Nucl. Phys. 133, 104070 (2023).
https://www.sciencedirect.com/science/article/abs/pii/S0146641023000510?via%3Dihub
Related to Project A01, A02, C05
Abstract:
We compute masses of the two lightest glueballs from spectral reconstructions of timelike interaction channels of the four-gluon vertex in Landau gauge Yang-Mills theory. The Euclidean spacelike dressings of the vertex are calculated with the functional renormalization group. For the spectral reconstruction of these Euclidean data, we employ Gaussian process regression. The glueball resonances can be identified straightforwardly and we obtain 𝑚𝑠𝑐=1870(75) MeV as well as 𝑚𝑝𝑠=2700(120) MeV, in accordance with functional bound state and lattice calculations.
J. M. Pawlowski, C. S. Schneider, J. Turnwald, J. M. Urban, N. Wink, „Yang-Mills glueball
masses from spectral reconstruction“, Phys. Rev. D 108, 076018 (2023).
https://journals.aps.org/prd/abstract/10.1103/PhysRevD.108.076018
Related to Project A02, C06
Abstract:
We prove a general class of continuous variable entanglement criteria based on the Husimi Q distribution, which represents a quantum state in canonical phase space, by employing a theorem by Lieb and Solovej. We discuss their generality, which roots in the possibility to optimize over the set of concave functions, from the perspective of continuous majorization theory and show that with this approach families of entropic as well as second moment criteria follow as special cases. All derived criteria are compared with corresponding marginal based criteria, and the strength of the phase-space approach is demonstrated for a family of prototypical example states where only our criteria flag entanglement. Furthermore, we explore their optimization prospects in two experimentally relevant scenarios characterized by sparse data: Finite detector resolution and finite statistics. In both scenarios optimization leads to clear improvements enlarging the class of detected states and the signal-to-noise ratio of the detection, respectively.
M. Gärttner, T. Haas, J. Noll, „Detecting continuous-variable entanglement in phase space with the Q distribution“, Phys. Rev. A 108, 042410 (2023).
https://journals.aps.org/pra/abstract/10.1103/PhysRevA.108.042410
Related to Project A06
Abstract:
We present a general class of entanglement criteria for continuous variable systems. Our criteria are based on the Husimi Q distribution and allow for optimization over the set of all concave functions rendering them extremely general and versatile. We show that several entropic criteria and second moment criteria are obtained as special cases. Our criteria reveal entanglement of families of states undetected by any commonly used criteria and provide clear advantages under typical experimental constraints such as finite detector resolution and measurement statistics.
M. Gärttner, T. Haas, J. Noll, „General Class of Continuous Variable Entanglement Criteria“, Phys. Rev. Lett. 131, 150201 (2023).
https://journals.aps.org/prl/abstract/10.1103/PhysRevLett.131.150201
Related to Project A06
Abstract:
We calculate the spectral function of light quark flavours in 2+1 flavour vacuum QCD in the isospin-symmetric approximation. We employ spectral Dyson-Schwinger equations and compute the non-perturbative quark propagator directly in real-time, using recent spectral reconstruction results from Gaussian process regression of gluon propagator data in 2+1 flavour lattice QCD. Our results feature a pole-like peak structure at time-like momenta larger than the propagator’s gapping scale as well as a negative scattering continuum, which we exploit assuming an analytic pole-tail split during the iterative solution. The computation is augmented with a general discussion of the impact of the quark-gluon vertex and the gluon propagator on the analytic structure of the quark propagator. In particular, we investigate under which conditions the quark propagator shows unphysical complex poles. Our results offer a wide range of applications, encompassing the ab-initio calculation of transport as well as resonance properties in QCD.
J. Horak, J. M. Pawlowski, N. Wink, „On the quark spectral function in QCD“, SciPost Phys. 15, 149 (2023).
https://scipost.org/10.21468/SciPostPhys.15.4.149
Related to Project A02, C06
Press Release Max-Planck-Institut für Kernphysik Heidelberg
04.10.2023
Measurement of the g factor of the electron in hydrogen-like tin confirms the standard model of particle physics under extremely high electric field strengths.
Quantum electrodynamics is the best-tested theory in physics. It describes all electrical and magnetic interactions of light and matter. Scientists at the Max-Planck-Institut für Kernphysik in Heidelberg (MPIK) have now used precision measurements on their ALPHATRAP experiment to investigate the magnetic properties of electrons bound to highly ionized tin atoms. Such tests provide insights into the behavior of particles under extreme field strengths. They also serve as a starting point for the search for new physics.
Like other charged elementary particles, the electron has a magnetic moment. Its exact value is one of the most precisely known measurements in physics and is in excellent agreement with the theoretical predictions of quantum electrodynamics. Theory and experiment are in agreement to more than ten decimal places. However, these values apply only to the “free” electron, which is not subject to external fields. But especially in the case of very strong fields, such as those present in the immediate vicinity of atomic nuclei, additional factors could come into play – such as previously unknown elementary particles or deviations from the known laws of nature.
A research team combining scientists from several divisions at MPIK has now achieved an important milestone here. “We have succeeded in producing hydrogen-like tin ions and storing them for months in the ALPHATRAP ion trap,” says Jonathan Morgner, first author of the new study and PhD student in Professor Klaus Blaum’s division. The experiment is led by Sven Sturm, and the theory group led by Zoltán Harman from Christoph Keitel’s division performed the calculations. Morgner continues, “Thanks to the long storage time, we were able to measure the magnetic moment with unprecedented precision.” The study appeared in the prestigious journal Nature.
Hydrogen-like tin has only one electron in its shell – just like conventional hydrogen. However, the atomic nucleus of tin has 50 protons, and the neutral element consist of 50 electrons in its shell. “So we first had to remove 49 electrons,” Morgner says. “To achieve this, we used an electron beam ion trap, the Heidelberg-EBIT, which is a device for generating highly charged ions, developed by José Crespo López-Urrutia from the division of Thomas Pfeifer.” In this experimental setup, a cloud of about 100,000 tin ions is bombarded with high-energy electrons. In the process, the ions successively lose their bound electrons. Afterwards the ions that have only one electron left in their shells are filtered and fed into the particle trap of the ALPHATRAP experiment. There, the magnetic properties of the electrons are measured.
ALPHATRAP is a high-precision experiment whose heart is a Penning trap, in which charged particles are held in place with electromagnetic fields. It also has a cryogenic vacuum system that uses low temperatures to create an extremely good vacuum. This is necessary as the highly charged tin ions would immediately acquire electrons from any surrounding atoms. This would make longer-term measurements impossible. In addition, the preparation of hydrogen-like tin is very time-consuming.
The researchers were then able to measure the so-called g factor of the electron on the captured tin ions using irradiated microwaves. At the matching frequency, the electrons in the applied magnetic field in the trap make so-called spin flips – i.e. a reorientation of their “magnetic needle”. This effect allows highly accurate measurements of the g factor, also called “gyromagnetic factor”. This is a measure of how strong the magnetic field of the electron is.
The dimensionless value of the g factor is about 2. The exact value can be predicted by quantum electrodynamics, and depends on the environment of the electron. And this is where the highly charged tin ions come into play. Since they have only one electron, they can be described in theory similarly to a hydrogen atom. This simplifies the calculations enormously. However, due to the high charge of the tin nucleus, extremely high electric fields of around 1015 volts per centimetre are present on the position of the electron around the atomic nucleus. These field strengths are orders of magnitude stronger than what can be realized today even with the most powerful laser systems in any experiments. Thus, such atomic nuclei are ideally suited to test the predictions of quantum electrodynamics especially in the high-field regime, i.e., under extreme conditions.
The complicated calculations of the theoretical value of the g factor at strong fields provide somewhat less precise predictions than for the free electron, due to the additional interaction with the nucleus. According to theory, the g factor should be gtheo = 1.910 561 821 (299). The experimental value measured in the ALPHATRAP apparatus has much higher accuracy and is gexp = 1.910 562 059 (1). “The two values are in very good agreement, so this is an excellent confirmation of quantum electrodynamics,” Morgner said. “This shows that our previous understanding of physics works even at such extreme fields.” Until now, comparable measurements of the g factor of electrons had only been performed for much lighter elements such as silicon.
Thanks to the successful measurements, the next step would be to experiment with even heavier ions. Hydrogen-like lead or uranium have a much higher electric field than tin. Further upgrades are currently being implemented to enable further and even stronger fundamental tests of quantum electrodynamics in the future.
(Dirk Eidemüller / MPIK)
Original publication:
Stringent test of QED with hydrogenlike tin
J. Morgner, B. Tu, C.M. König, T. Sailer, F. Heiße, H. Bekker, B. Sikora, C. Lyu, V. A. Yerokhin, Z. Harman, J. R. Crespo López-Urrutia, C. H. Keitel, S. Sturm and K. Blaum
Nature (04.10.2023). DOI: 10.1038/s41586-023-06453-2
Contact
PD Dr. Sven Sturm
MPI für Kernphysik
sven.sturm@mpi-hd.mpg.de
Tel.: +49 6221 516-447
PD Dr. Zoltán Harman
MPI für Kernphysik
harman@mpi-hd.mpg.de
Tel.: +49 6221 516-163
Pressemitteilung Max-Planck-Institut für Kernphysik Heidelberg
04.10.2023
Messung des g-Faktors des Elektrons in wasserstoffartigem Zinn bestätigt das Standardmodell der Teilchenphysik unter extrem hohen elektrischen Feldstärken.
Die Quantenelektrodynamik ist die am besten getestete Theorie der gesamten Physik. Sie beschreibt alle elektrischen und magnetischen Wechselwirkungen von Licht und Materie. Wissenschaftler:innen des Max-Planck-Instituts für Kernphysik in Heidelberg (MPIK) haben jetzt mit Präzisionsmessungen an ihrem Experiment ALPHATRAP die magnetischen Eigenschaften von Elektronen untersucht, die an hochgradig ionisierte Zinn-Atome gebunden waren. Solche Tests ermöglichen Einblicke in das Verhalten von Teilchen unter extremen Feldstärken. Außerdem dienen sie als Plattform für die Suche nach neuer Physik.
Wie die anderen geladenen Elementarteilchen besitzt auch das Elektron ein magnetisches Moment. Dessen genauer Betrag ist einer der am präzisesten bekannten Messwerte und befindet sich in hervorragendem Einklang mit den theoretischen Vorhersagen der Quantenelektrodynamik. Theorie und Experiment sind zu mehr als zehn Nachkommastellen in Einklang. Allerdings gelten diese Werte nur für das „freie“ Elektron, das keinen externen Feldern unterliegt. Gerade bei sehr starken Feldern, wie sie in unmittelbarer Nähe von Atomkernen vorliegen, könnten jedoch neue Faktoren ins Spiel kommen – etwa bislang unbekannte Elementarteilchen oder Abweichungen von den bekannten Naturgesetzen.
Ein Forschungsteam aus mehreren Arbeitsgruppen am MPIK hat hier nun einen wichtigen Meilenstein erzielt. „Es ist uns gelungen, wasserstoffartige Zinn-Ionen zu erzeugen und monatelang in unserer ALPHATRAP-Ionenfalle zu speichern“, sagt Jonathan Morgner, Erstautor der neuen Studie und Doktorand der Forschungsgruppe von Professor Klaus Blaum. Geleitet wird das Experiment von Sven Sturm, die Theoriegruppe um Zoltán Harman aus der Abteilung von Christoph Keitel hat die Berechnungen durchgeführt. Morgner fährt fort: „Dank der langen Speicherdauer konnten wir das magnetische Moment mit bisher unerreichter Genauigkeit vermessen.“ Die Studie ist in der renommierten Fachzeitschrift „Nature“ erschienen.
Wasserstoffartiges Zinn besitzt lediglich ein Elektron in der Hülle – genau wie herkömmlicher Wasserstoff. Der Atomkern von Zinn weist jedoch 50 Protonen auf, so dass dieses Element normalerweise auch 50 Elektronen in der Schale hat. „Wir mussten also zunächst 49 Elektronen entfernen“, sagt Morgner. „Dazu haben wir unsere Elektronenstrahl-Ionenfalle genutzt, die Heidelberg-EBIT, eine Falle zur Erzeugung hochgeladener Ionen, die von José Crespo López-Urrutia aus der Abteilung von Thomas Pfeifer entwickelt wurde.“ In dieser Anlage wird eine Wolke aus rund 100.000 Zinn-Ionen mit hochenergetischen Elektronen beschossen. Dabei verlieren die Ionen sukzessive ihre Elektronen. Ein Filtersystem separiert aus diesen Ionen dann die wenigen aus, die nur noch ein Elektron in der Hülle haben, und speist diese in die Teilchenfalle des ALPHATRAP-Experiments ein. Dort werden dann die magnetischen Eigenschaften der Elektronen vermessen.
ALPHATRAP ist ein Hochpräzisions-Experiment, dessen Herzstück eine Penning-Falle ist, in dieser werden geladene Teilchen mit elektromagnetischen Feldern festgehalten. Außerdem besitzt sie ein kryogenes Vakuumsystem, das mit Hilfe tiefer Temperaturen ein extrem gutes Vakuum erzeugt. Das ist notwendig, weil die hochgeladenen Zinn-Ionen etwaigen Fremdatomen sofort Elektronen entreißen. Das würde längerfristige Messreihen unmöglich machen. Zudem ist die Präparation wasserstoffartigen Zinns sehr aufwändig.
An den gefangenen Zinn-Ionen konnten die Forscher:innen dann mit Hilfe eingestrahlter Mikrowellen den sogenannten g-Faktor des Elektrons messen. Bei der passenden Frequenz machen die Elektronen in dem angelegten Magnetfeld in der Falle sogenannte Spin-Flips – also Umorientierung ihrer „Magnetnadel“. Dieser Effekt erlaubt hochgenaue Messungen des g-Faktors, auch „gyromagnetischer Faktor“ genannt. Dies ist ein Maß dafür, wie stark das Magnetfeld des Elektrons ist.
Der dimensionslose Wert des g-Faktors ist ungefähr 2. Der genaue Wert kann von der Quantenelektrodynamik vorhergesagt werden, und hängt vom Umfeld des Elektrons ab. Und hier kommen die hochgeladenen Zinn-Ionen ins Spiel. Da sie nur ein Elektron besitzen, lassen sie sich von der Theorie her ähnlich wie ein Wasserstoffatom beschreiben. Das vereinfacht die Berechnungen enorm. Allerdings liegen auf der engen Bahn des Elektrons um den Atomkern aufgrund der hohen Ladung des Zinnkerns extrem hohe elektrische Felder von rund 1015 Volt pro Zentimeter vor. Derartige Feldstärken sind um Größenordnungen stärker als das, was sich heute selbst mit den stärksten Lasersystemen in solchen Experimenten realisieren lässt. Damit eignen sich derartige Atomkerne hervorragend, um die Vorhersagen der Quantenelektrodynamik auch im Hochfeld-Regime, d.h. unter extremen Bedingungen, zu überprüfen.
Die komplizierten Berechnungen zum Theoriewert des g-Faktors bei starken Feldern liefern etwas weniger präzise Vorhersagen als beim freien Elektron, aufgrund der zusätzlichen Interaktion mit dem Atomkern. Der g-Faktor sollte laut Theorie gtheo = 1,910 561 821 (299) betragen. Der in der ALPHATRAP-Ionenfalle gemessene experimentelle Wert hat eine wesentlich höhere Genauigkeit und beträgt gexp = 1,910 562 059 (1). „Die beiden Werte sind in sehr guter Übereinstimmung, das ist also eine ausgezeichnete Bestätigung der Quantenelektrodynamik“, so Morgner. „Unser bisheriges Verständnis von Physik funktioniert also auch bei solch extremen Feldern.“ Bislang waren vergleichbare Messungen des g-Faktors von Elektronen nur bei deutlich leichteren Elementen wie Silizium durchgeführt worden.
Dank der geglückten Messungen tut sich nun die Möglichkeit auf, auch mit noch schwereren Ionen zu arbeiten. Wasserstoffartiges Blei oder Uran weisen nochmals ein wesentlich höheres elektrisches Feld auf als Zinn. Die Heidelberger Anlage wird deshalb bereits modernisiert, um weitere und noch stärkere fundamentale Tests der Quantenelektrodynamik zu ermöglichen.
(Dirk Eidemüller)
Originalpublikation:
Stringent test of QED with hydrogenlike tin
J. Morgner, B. Tu, C.M. König, T. Sailer, F. Heiße, H. Bekker, B. Sikora, C. Lyu, V. A. Yerokhin, Z. Harman, J. R. Crespo López-Urrutia, C. H. Keitel, S. Sturm and K. Blaum
Nature (04.10.2023). DOI: 10.1038/s41586-023-06453-2
Kontakt
PD Dr. Sven Sturm
MPI für Kernphysik
sven.sturm@mpi-hd.mpg.de
Tel.: +49 6221 516-447
PD Dr. Zoltán Harman
MPI für Kernphysik
harman@mpi-hd.mpg.de
Tel.: +49 6221 516-163
Abstract:
Inner-shell electrons naturally sense the electric field close to the nucleus, which can reach extreme values beyond 1015 V cm−1 for the innermost electrons1. Especially in few-electron, highly charged ions, the interaction with the electromagnetic fields can be accurately calculated within quantum electrodynamics (QED), rendering these ions good candidates to test the validity of QED in strong fields. Consequently, their Lamb shifts were intensively studied in the past several decades2,3. Another approach is the measurement of gyromagnetic factors (g factors) in highly charged ions4,5,6,7. However, so far, either experimental accuracy or small field strength in low-Z ions5,6 limited the stringency of these QED tests. Here we report on our high-precision, high-field test of QED in hydrogen-like 118Sn49+. The highly charged ions were produced with the Heidelberg electron beam ion trap (EBIT)8 and injected into the ALPHATRAP Penning-trap setup9, in which the bound-electron g factor was measured with a precision of 0.5 parts per billion (ppb). For comparison, we present state-of-the-art theory calculations, which together test the underlying QED to about 0.012%, yielding a stringent test in the strong-field regime. With this measurement, we challenge the best tests by means of the Lamb shift and, with anticipated advances in the g-factor theory, surpass them by more than an order of magnitude.
J. Morgner, B. Tu, C. M. König, T. Sailer, F. Heiße, H. Bekker, B. Sikora, C. Lyu, V. A. Yerokhin, Z. Karman, J. R. Crespo López-Urrutia, C. H. Keitel, S. Sturm, K. Blaum, “Stringent test of QED with hydrogen-like tin”, Nature 622, 53-57 (2023).
https://doi.org/10.1038/s41586-023-06453-2
Related to Project B01, B02
Abstract:
Emerging sampling algorithms based on normalizing flows have the potential to solve ergodicity problems in lattice calculations. Furthermore, it has been noted that flows can be used to compute thermodynamic quantities which are difficult to access with traditional methods. This suggests that they are also applicable to the density-of-states approach to complex action problems. In particular, flow-based sampling may be used to compute the density directly, in contradistinction to the conventional strategy of reconstructing it via measuring and integrating the derivative of its logarithm. By circumventing this procedure, the accumulation of errors from the numerical integration is avoided completely and the overall normalization factor can be determined explicitly. In this proof-of-principle study, we demonstrate our method in the context of two-component scalar field theory where the internal 𝑂(2) symmetry is explicitly broken by an imaginary external field. First, we concentrate on the zero-dimensional case which can be solved exactly. We show that with our method, the Lee-Yang zeroes of the associated partition function can be successfully located. Subsequently, we confirm that the flow-based approach correctly reproduces the density computed with conventional methods in one- and two-dimensional models.
J. M. Pawlowski, J. M. Urban, „Flow-based density of states for complex actions“, Phys. Rev. D 108, 054511 (2023).
https://journals.aps.org/prd/abstract/10.1103/PhysRevD.108.054511
Related to Project A01, A02, C01, C02, C06
Abstract:
We present a comprehensive non-perturbative study of the phase structure of the asymptotically safe Standard Model. The physics scales included range from the asymptotically safe trans-Planckian regime in the ultraviolet, the intermediate high-energy regime with electroweak symmetry breaking to strongly correlated QCD in the infrared. All flows are computed with a self-consistent functional renormalisation group approach, using a vertex expansion in the fluctuation fields. In particular, this approach takes care of all physical threshold effects and the respective decoupling of ultraviolet degrees of freedom. Standard Model and gravity couplings and masses are fixed by their experimental low energy values. Importantly, we accommodate for the difference between the top pole mass and its Euclidean analogue. Both, the correct mass determination and the threshold effects have a significant impact on the qualitative properties, and in particular on the stability properties of the specific ultraviolet-infrared trajectory with experimental Standard Model physics in the infrared. We show that in the present rather advanced approximation the matter part of the asymptotically safe Standard Model has the same number of relevant parameters as the Standard Model, and is asymptotically free. This result is based on the novel UV fixed point found in the present work: the fixed point Higgs potential is flat but has two relevant directions. These results and their analysis are accompanied by a thorough discussion of the systematic error of the present truncation, also important for systematic improvements.
Á. Pastor-Gutiérrez, J. M. Pawlowski, M. Reichert, „The Asymptotically Safe Standard Model:
From quantum gravity to dynamical chiral symmetry breaking“, SciPost Phys. 15, 105 (2023).
https://scipost.org/10.21468/SciPostPhys.15.3.105
Related to Project A02
Abstract:
We study nonequilibrium dynamics of SU(2) lattice gauge theory in Minkowski space-time in a classical-statistical regime, where characteristic gluon occupancies are much larger than unity. In this strongly correlated system far from equilibrium, the correlations of energy and topological densities show self-similar behavior related to a turbulent cascade toward higher momentum scales. We employ persistent homology to infer topological features of the gluonic plasma via a hierarchy of simplicial and cubical complexes. All topological observables under investigation are also manifestly gauge invariant and are shown to exhibit self-similar evolution, which relates the spatial and temporal properties of the plasma in terms of universal scaling exponents and functions. The findings may help one to understand the early stages of heavy-ion collisions in the limit of high energies, and our methods can also facilitate the topological analysis of other complex systems such as those encountered in experiments with ultracold quantum gases.
D. Spitz, K. Boguslavski, J. Berges, “Probing universal dynamics with topological data analysis
in a gluonic plasma”, Phys. Rev. D 108, 056016 (2023).
https://journals.aps.org/prd/abstract/10.1103/PhysRevD.108.056016
Related to Project B03
Abstract:
We derive renormalised finite functional flow equations for quantum field theories in real and imaginary time that incorporate scale transformations of the renormalisation conditions, hence implementing a flowing renormalisation. The flows are manifestly finite in general non-perturbative truncation schemes also for regularisation schemes that do not implement an infrared suppression of the loops in the flow. Specifically, this formulation includes finite functional flows for the effective action with a spectral Callan-Symanzik cutoff, and therefore gives access to Lorentz invariant spectral flows. The functional setup is fully non-perturbative and allows for the spectral treatment of general theories. In particular, this includes theories that do not admit a perturbative renormalisation such as asymptotically safe theories. Finally, the application of the Lorentz invariant spectral functional renormalisation group is briefly discussed for theories ranging from real scalar and Yukawa theories to gauge theories and quantum gravity.
J. Braun, Y. Chen, W. Fu, A. GeiSSel, J. Horak, C. Huang, F. Ihssen, J. M. Pawlowski, M. Reichert, F. Rennecke, Y. Tan, S. Töpfel, J. Wessely, N. Wink, „Renormalised spectral flows“, SciPost Phys. Core 6, 061 (2023).
https://scipost.org/10.21468/SciPostPhysCore.6.3.061
Related to Project A02, B03, C01, C02, C06
Abstract:
In the present work we set up a general functional renormalisation group framework for the computation of complex effective actions. For explicit computations we consider both flows of the Wilsonian effective action and the one-particle irreducible (1PI) effective action. The latter is based on an appropriate definition of a Legendre transform for complex actions, and we show its validity by comparison to exact results in zero dimensions, as well as a comparison to results for the Wilsonian effective action. In the present implementations of the general approaches, the flow of the Wilsonian effective action has a wider range of applicability and we obtain results for the effective potential of complex fields in -theories from zero up to four dimensions. These results are also compared with results from the 1PI effective action within its range of applicability. The complex effective action also allows us to determine the location of the Lee-Yang zeros for general parameter values. We also discuss the extension of the present results to general theories including QCD.
F. Ihssen, J. M. Pawlowski, “Functional flows for complex effective actions”, SciPost Phys. 15, 074 (2023).
https://scipost.org/10.21468/SciPostPhys.15.2.074
Related to Project A02, C05, C01
Abstract:
We report on a scheme for loading dysprosium atoms into a narrow-line three-dimensional magneto-optical trap (3D MOT). Our approach replaces the conventional Zeeman slower with a two-dimensional (2D) MOT operating on the broad 421-nm line to create a high-flux beam of slow atoms. Even in the absence of a push beam, we demonstrate efficient loading of the 3D MOT, which operates on the narrower 626-nm intercombination line. Adding push beams working at either 421 nm or 626 nm, significant enhancement of the loading rate is achieved. We reach the best performance, with an enhancement factor of 3.6, using a push beam red-detuned to the 626-nm line. With loading rates greater than 108atoms/s achieved at a moderate oven reservoir temperature of 800∘C, our method offers similar or greater performance than Zeeman-slower-based systems. Our 2D-MOT-based approach constitutes a promising first step towards state-of-the-art quantum gas experiments with several advantages over the Zeeman-slower-based setup and is readily adaptable to other open-shell lanthanides.
S. Jin, J. Gao, K. Chandrashekara, C. Gölzhäuser, J. Schöner, L. Chomaz, “Two-dimensional magneto-optical trap of dysprosium atoms as a compact source for efficient loading of a narrowline three-dimensional magneto-optical trap”, Phys. Rev. A 108, 023719 (2023).
https://journals.aps.org/pra/abstract/10.1103/PhysRevA.108.023719
Related to Project A07
Abstract:
We show that scalar quantum field theory in four Euclidean dimensions with global O(N)3 symmetry and imaginary tetrahedral coupling is asymptotically free and bounded from below in the large-N limit. While the Hamiltonian is non-Hermitian, the full quantum effective action for the large-N theory only depends on the square of that coupling which is real. A perturbative analysis uncovers that the renormalization group flow of the quartic couplings connects a Gaussian ultraviolet fixed point to a strongly interacting theory in the infrared. This realizes a renormalizable field theory which exhibits nontrivial dynamics, such as direct scattering, while still being analytically tractable also nonperturbatively. Our findings open up a way to address outstanding problems in strongly coupled theories from first principles.
J. Berges, R. Gurau, T. Preis, “Asymptotic freedom in a strongly interacting scalar quantum field theory in four Euclidean dimensions”, Phys. Rev. D 108, 016019 (2023).
https://journals.aps.org/prd/abstract/10.1103/PhysRevD.108.016019
Related to Project B03
We are pleased to announce that registration for the annual retreat of the IsoQuant YRC of the SFB 1225 is now open!
The retreat will be held from 25 to 28 of September at the Hotel Loewen in the Schwarzwald.
The main goal of this event is to provide a platform for cross-project collaboration and an opportunity to intensify exchange and networking between young researchers (undergraduates, PhD students, PostDocs) within IsoQuant.
The retreat is intended to be highly interactive and participants will get to know the main topics both through introductory talks, as well as through hands-on miniature projects to deepen their understanding of the topics.
The following invited speakers will join this workshop:
-
Dr. Fabian Renneke (University of Giessen) on QCD and the functional Renormalization Group,
-
Dr. Robert Ott (University of Innsbruck & IQOQI Austrian Academy of Sciences) on quantum many-body physics,
-
Dr. Maximillian Prüfer (TU Vienna), on non-equilibrium quantum many body systems.
To participate, please fill out the registration form.
Please note that the total number of participants is set at 25 and all costs are covered. The registration deadline is 31 August. More details will follow once registration closes.
If you have any questions, please do not hesitate to contact us at sfb1225@thphys.uni-heidelberg.de.
Abstract:
We consider two-component fermions with a zero-range interaction both in two and three dimensions and calculate the bulk viscosity for an arbitrary scattering length in the high-temperature regime. We evaluate the Kubo formula for the bulk viscosity using an expansion with respect to the fugacity, which acts as a small parameter at high temperatures. In the zero-frequency limit of the Kubo formula, pinch singularities emerge that reduce the order of the fugacity by one. These singularities can turn higher-order vertex corrections at nonzero frequencies into the leading order at zero frequency, so that all such contributions have to be resummed. We present an exact microscopic computation for the bulk viscosity in the high-temperature regime by taking into account these pinch singularities. For negative scattering lengths, we derive the complete bulk viscosity at second order in fugacity and show that a self-consistent equation to resum the vertex corrections is identical to a linearized kinetic equation. For positive scattering lengths, a new type of pinch singularity arises for bound pairs. We show that the pinch singularity for bound pairs leads to a first-order contribution to the bulk viscosity, which is one order lower than that for negative scattering lengths, and that the vertex corrections also provide first-order contributions. We propose a new kinetic equation for bound pairs that derives from a self-consistent equation to resum the vertex corrections.
K. Fujii, T. Enss, “Bulk viscosity of resonantly interacting fermions in the quantum virial
expansion”, Annals of Physics 453, 169296 (2023).
https://www.sciencedirect.com/science/article/abs/pii/S0003491623000817?via%3Dihub
Related to Project C02, C03, ABC
Abstract:
We present the Mathematica package QMeS-Derivation, available on GitHub. It derives symbolic functional equations from a given master equation. The latter include functional renormalisation group equations, Dyson-Schwinger equations, Slavnov-Taylor and Ward identities and their modifications in the presence of momentum cutoffs. The modules allow to derive the functional equations, take functional derivatives, trace over field space, apply a given truncation scheme, and do momentum routings while keeping track of prefactors and signs that arise from fermionic commutation relations. The package furthermore contains an installer as well as Mathematica notebooks with showcase examples.
J. M. Pawlowski, C. S. Schneider, N. Wink, “QMeS-Derivation: Mathematica package for the symbolic derivation of functional equations”, Comput. Phys. Commun. 287 (2023).
https://www.sciencedirect.com/science/article/abs/pii/S0010465523000565?via%3Dihub
Related to Project A02, C01
Abstract:
A frequent starting point of quantum computation platforms are two-state quantum systems, i.e., qubits. However, in the context of integer optimization problems, relevant to scheduling optimization and operations research, it is often more resource-efficient to employ quantum systems with more than two basis states, so-called qudits. Here, we discuss the quantum approximate optimization algorithm (QAOA) for qudits, layout its implementation in platforms with long-range interactions between qudits such as trapped ions, cold atomic mixtures, Rydberg atoms and atoms in cavities. We illustrate how the QAOA can be used to formulate a variety of integer optimization problems such as graph coloring problems or electric vehicle (EV) charging optimization. In addition, we comment on the implementation of constraints and describe three methods to include these into a quantum circuit of a QAOA by penalty contributions to the cost Hamiltonian, conditional gates using ancilla qubits, and a dynamical decoupling strategy. Finally, as a showcase of qudit-based QAOA, we present numerical results for a charging optimization problem mapped onto a max-k-graph coloring problem. Our work illustrates the flexibility of qudit systems to solve integer optimization problems.
Y. Deller, S. Schmitt, M. Lewenstein, S. Lenk, Steve M. Federer, F. Jendrzejewski, P. Hauke, V. Kasper, “Quantum approximate optimization algorithm for qudit systems”, Phys. Rev. A 107, 062410 (2023).
https://journals.aps.org/pra/abstract/10.1103/PhysRevA.107.062410
Related to Project B04
Abstract:
We consider two heavy particles immersed in a Bose-Einstein condensate in three dimensions and compute their mutual interaction induced by excitations of the medium. For an ideal Bose gas, the induced interaction is Newtonian up to a shift in distance, which depends on the coupling strength between impurities and Bosons. For a real BEC, we find that, on short distances, the induced potential is dominated by three-body physics of a single Boson bound to the impurities, leading to an Efimov potential. At large distances of the order of the healing length, a Yukawa potential emerges instead. In particular, we find that both regimes are realized for all impurity-boson couplings and determine the corresponding crossover scales. The transition from the real to the ideal condensate at low gas parameters is investigated.
M. Drescher, M. Salmhofer, T. Enss, “Medium-induced interaction between impurities in a Bose-Einstein condensate”, Phys. Rev. A 107, 063301 (2023).
https://journals.aps.org/pra/abstract/10.1103/PhysRevA.107.063301
Related to Project C03, C02
Die Ausstellung “The History of Uncertainty (Die Geschichte der Verunsicherung)” des Künstlers Philip Kanwischer wird präsentiert von Isoquant und Kalamari Klub und wird am 15. Juni, 2023, 19:00 Uhr im Physikalischen Institut der Universität Heidelberg eröffnet
Während seiner Kooperation mit Forscher*innen des Sonderforschungsbereichs Isoquant beschäftigte sich Philip Kanwischer mit der fundamentalen Rolle des Zufalls in der Quantenphysik. Im Zuge der Ausstellung „The History of Uncertainty (Die Geschichte der Verunsicherung)“ bespielt er nun einen Teil des Physikalischen Instituts mit den daraus resultierenden Arbeiten. Kanwischer, dessen Arbeiten häufig im assoziativen Spiel mit Sprache begründet sind, verknüpft den Begriff des Zufalls kulturgeschichtlich mit dem Meer. Von dort aus hangelt er sich in Form von Fotografien, Objekten und filmischen Collagen erzählerisch entlang gesunkener römischer Handelsschiffe, der Geschichte des Versicherungswesens und den aktuellen Lieferkettenproblemen, ohne dabei den Bezug zur Quantenphysik zu verlieren.
Die Ausstellung “The History of Uncertainty (Die Geschichte der Verunsicherung)” wird von Nicolas Reinhart kuratiert und ist Teil der SciArt-Kooperation des Sonderforschungsbereichs Isoquant an der Universität Heidelberg und dem internationalen Kollektiv Kalamari Klub. Die Kooperation wurde 2021 ins Leben gerufen und hat das Ziel, anhand der Zusammenarbeit zwischen Künstler:innen, Wissenschaftler:innen und der Öffentlichkeit den interdisziplinären Dialog und künstlerische Forschung zu fördern.
Der Künstler Philip Kanwischer wurde im Rahmen der SciArt-Kooperation als Artist-in-Residence eingeladen und arbeitete zwischen Oktober 2021 und März 2022 in Heidelberg. Kanwischer,
geboren 1991 in Bamberg, studierte Fotografie an der Hochschule für Grafik und Buchkunst in Leipzig und an der Accademia die Belle Arti in Napoli, Italien. In seinen Arbeiten verknüpft Kanwischer Bild und Textmaterial zu narrativen Raumsituationen und thematisiert dabei u.a. die Wahrnehmung von Katastrophen und das Spannungsverhältnis zwischen Mensch und Natur. Seine Werke wurden in zahlreichen Einzel- und Gruppenausstellungen gezeigt. Er lebt und arbeitet in Leipzig.
Eröffnung:
Donnerstag, 15. Juni 2023, 19:00
Physikalisches Institut, Im Neuenheimer Feld 226, 69120 Heidelberg
Begrüßung und Einführung durch:
Dr. Lisa Ringena, Science Communication, Isoquant
Tina Kuka, Coordination, Isoquant
Nicolas Reinhart. Artistic Director, Kalamari Klub
Prof. Dr. Markus K. Oberthaler. Steering Board Member, Isoquant
Der Künstler ist anwesend.
Pressekontakt
Nicolas Reinhart
Artistic Director, Kalamari Klub
nico@kalamariklub.org
Dr. Lisa Ringena, Science Communication, IsoQuant
Tina Kuka, Coordination, IsoQuant
scicom-isoquant@thphys.uni-heidelberg.de
Mehr Information
https://www.kalamariklub.org
https://www.isoquant-heidelberg.de
The exhibition “The History of Uncertainty (Die Geschichte der Verunsicherung)” by the artist Philip Kanwischer is presented by IsoQuant and Kalamari Klub and opens June 15, 2023, 19:00 CET at Physikalisches Institut, University of Heidelberg.
During his collaboration with researchers from the Collaborative Research Centre IsoQuant, Philip Kanwischer explored the fundamental role of probability in quantum physics. In his exhibition “The History of Uncertainty (Die Geschichte der Verunsicherung)”, he now shows the resulting works in a section of the Physikalisches Institut. Kanwischer, whose works are often rooted in an associative play of language, connects the concept of probability to the sea. Starting from there, he uses photographs, objects and cinematic collages to navigate narratively through sunken Roman merchant ships, the history of insurance, and current supply chain issues, all the while maintaining the connection to quantum physics.
The exhibition “The History of Uncertainty (Die Geschichte der Verunsicherung)” is curated by Nicolas Reinhart and produced as part of a SciArt collaboration between the Collaborative Research Centre IsoQuant at the University of Heidelberg and the international artist collective Kalamari Klub. The collaboration was established in 2021 and aims to initiate curiosity-driven engagement between artists, scientists and other members of the society, promoting a spirit of co-learning and equality while helping to communicate knowledge through cross-disciplinary collaboration and artistic research.
The artist Philip Kanwischer was invited as SciArt artist-in-residence and spent six months in Heidelberg between October 2021 and March 2022. Kanwischer, born 1991 in Bamberg, Germany,
studied Photography at the Academy of Fine Arts in Leipzig, Germany and at the Accademia di Belle Arti in Napoli, Italy. In his work, he combines images and text into narrative installations, studying the perception of catastrophic events and the tense relation between human and nature. His works have been included in numerous solo and group shows. He lives and works in Leipzig, Germany.
The History of Uncertainty (Die Geschichte der Verunsicherung)
Exhibition by Philip Kanwischer, 16.6.–21.7.2023
Opening reception: Thursday, June 15, 2023, 19:00 CET
Physikalisches Institut, Im Neuenheimer Feld 226, 69120 Heidelberg
Welcome and introductions by:
Dr. Lisa Ringena, Science Communication, IsoQuant
Tina Kuka, Coordination, IsoQuant
Nicolas Reinhart. Artistic Director, Kalamari Klub
Prof. Dr. Markus K. Oberthaler. Steering Board Member, IsoQuant
The artist is present.
Presented by IsoQuant and Kalamari Klub
Curated by Nicolas Reinhart
Press contacts:
Nicolas Reinhart
Artistic Director, Kalamari Klub
nico@kalamariklub.org
Dr. Lisa Ringena, Science Communication, IsoQuant
Tina Kuka, Coordination, IsoQuant
scicom-isoquant@thphys.uni-heidelberg.de
More information:
https://www.kalamariklub.org
https://www.isoquant-heidelberg.de
The exhibition “The History of Uncertainty (Die Geschichte der Verunsicherung)” by the artist Philip Kanwischer is presented by IsoQuant and Kalamari Klub and opens June 15, 2023, 19:00 CET at Physikalisches Institut, University of Heidelberg.
During his collaboration with researchers from the Collaborative Research Centre IsoQuant, Philip Kanwischer explored the fundamental role of probability in quantum physics. In his exhibition “The History of Uncertainty (Die Geschichte der Verunsicherung)”, he now shows the resulting works in a section of the Physikalisches Institut. Kanwischer, whose works are often rooted in an associative play of language, connects the concept of probability to the sea. Starting from there, he uses photographs, objects and cinematic collages to navigate narratively through sunken Roman merchant ships, the history of insurance, and current supply chain issues, all the while maintaining the connection to quantum physics.
The exhibition “The History of Uncertainty (Die Geschichte der Verunsicherung)” is curated by Nicolas Reinhart and produced as part of a SciArt collaboration between the Collaborative Research Centre IsoQuant at the University of Heidelberg and the international artist collective Kalamari Klub. The collaboration was established in 2021 and aims to initiate curiosity-driven engagement between artists, scientists and other members of the society, promoting a spirit of co-learning and equality while helping to communicate knowledge through cross-disciplinary collaboration and artistic research.
The artist Philip Kanwischer was invited as SciArt artist-in-residence and spent six months in Heidelberg between October 2021 and March 2022. Kanwischer, born 1991 in Bamberg, Germany,
studied Photography at the Academy of Fine Arts in Leipzig, Germany and at the Accademia di Belle Arti in Napoli, Italy. In his work, he combines images and text into narrative installations, studying the perception of catastrophic events and the tense relation between human and nature. His works have been included in numerous solo and group shows. He lives and works in Leipzig, Germany.
The History of Uncertainty (Die Geschichte der Verunsicherung)
Exhibition by Philip Kanwischer, 16.6.–21.7.2023
Opening reception: Thursday, June 15, 2023, 19:00 CET
Physikalisches Institut, Im Neuenheimer Feld 226, 69120 Heidelberg
Welcome and introductions by:
Dr. Lisa Ringena, Science Communication, IsoQuant
Tina Kuka, Coordination, IsoQuant
Nicolas Reinhart. Artistic Director, Kalamari Klub
Prof. Dr. Markus K. Oberthaler. Steering Board Member, IsoQuant
The artist is present.
Presented by IsoQuant and Kalamari Klub
Curated by Nicolas Reinhart
Press contacts:
Nicolas Reinhart
Artistic Director, Kalamari Klub
nico@kalamariklub.org
Dr. Lisa Ringena, Science Communication, IsoQuant
Tina Kuka, Coordination, IsoQuant
scicom-isoquant@thphys.uni-heidelberg.de
More information:
https://www.kalamariklub.org
https://www.isoquant-heidelberg.de
Abstract:
We demonstrate the capability of flying focus (FF) laser pulses with ℓ=1 orbital angular momentum (OAM) to transversely confine ultrarelativistic charged particle bunches over macroscopic distances while maintaining a tight bunch radius. A FF pulse with ℓ=1 OAM creates a radial ponderomotive barrier that constrains the transverse motion of particles and travels with the bunch over extended distances. As compared with freely propagating bunches, which quickly diverge due to their initial momentum spread, the particles cotraveling with the ponderomotive barrier slowly oscillate around the laser pulse axis within the spot size of the pulse. This can be achieved at FF pulse energies that are orders of magnitude lower than required by Gaussian or Bessel pulses with OAM. The ponderomotive trapping is further enhanced by radiative cooling of the bunch resulting from rapid oscillations of the charged particles in the laser field. This cooling decreases the mean-square radius and emittance of the bunch during propagation.
M. Formanek, J. P. Palastro, M. Vranic, D. Ramsey, A. Di Piazza, “Charged particle beam transport in a flying focus pulse with orbital angular momentum”, Phys. Rev. E 107, 055213 (2023).
https://journals.aps.org/pre/abstract/10.1103/PhysRevE.107.055213
Related to Project B02
Abstract:
In this paper, we investigate the XY model in the presence of an additional potential term that independently tunes the vortex fugacity favouring their nucleation. By increasing the strength of this term and thereby the vortex chemical potential µ, we observe significant changes in the phase diagram with the emergence of a normal vortex-antivortex lattice as well as a superconducting vortex-antivortex crystal (lattice supersolid) phase. We examine the transition lines between these two phases and the conventional non-crystalline one as a function of both the temperature and the chemical potential. Our findings suggest the possibility of a peculiar tricritical point where second-order, first-order, and infinite-order transition lines meet. We discuss the differences between the present phase diagram and previous results for two-dimensional Coulomb gas models. Our study provides important insights into the behaviour of the modified XY model and opens up new possibilities for investigating the underlying physics of unconventional phase transitions.
I. Maccari, N. Defenu, C. Castellani, T. Enss, “Vortex supersolid in the XY model with tunable vortex fugacity”, J. Phys.: Condens. Matter 35, 334001 (2023).
https://iopscience.iop.org/article/10.1088/1361-648X/acd295
Related to Project C02, C03
Abstract:
We theoretically investigate three-body losses in a single-component Fermi gas near a p-wave Feshbach resonance in the interacting, nonunitary regime. We extend the cascade model introduced by Waseem et al. [M. Waseem, J. Yoshida, T. Saito, and T. Mukaiyama, Phys. Rev. A 99, 052704 (2019)] to describe the elastic and inelastic collision processes. We find that the loss behavior exhibits a n3 and an anomalous n2 density dependence for a ratio of elastic-to-inelastic collision rate larger and smaller than 1, respectively. The corresponding evolutions of the energy distribution show collisional cooling or evolution toward low-energetic nonthermalized steady states, respectively. These findings are particularly relevant for understanding atom loss and the energetic evolution of ultracold gases of fermionic lithium atoms in their ground state.
K.Welz, M. Gerken, B. Zhu, E. Lippi, M. Rautenberg, L. Chomaz, M.Weidemüller, “Anomalous loss behavior in a single-component Fermi gas close to a p-Wave Feshbach resonance”, Phys. Rev. A 107, 053310 (2023).
https://journals.aps.org/pra/abstract/10.1103/PhysRevA.107.053310
Related to Project C03
Abstract:
Neural quantum states are a new family of variational Ansätze for quantum-many body wave functions with advantageous properties in the notoriously challenging case of two spatial dimensions. Since their introduction, a wide variety of different network architectures have been employed to study paradigmatic models in quantum many-body physics with a particular focus on quantum spin models. Nonetheless, many questions remain about the effect that the choice of architecture has on the performance on a given task. In this work, we present a unified comparison of a selection of popular network architectures and symmetrization schemes employed for ground-state searches of prototypical spin Hamiltonians, namely, the two-dimensional transverse-field Ising model and the J1−J2 model. In the presence of a nontrivial sign structure of the ground states, we find that the details of symmetrization crucially influence the performance. We describe this effect in detail and discuss its consequences, especially for autoregressive models, as their direct sampling procedure is not compatible with the symmetrization procedure that we found to be optimal.
M. Reh, M. Schmitt, M. Gärttner, “Optimizing design choices for neural quantum states”, Phys. Rev. B 107, 195115 (2023).
https://journals.aps.org/prb/abstract/10.1103/PhysRevB.107.195115
Related to Project A06
Abstract:
We compute the strong coupling constant of Landau gauge QCD in the full complex momentum plane, both directly and via spectral reconstruction. In particular, we consider the Taylor coupling given by the product of ghost and gluon dressing functions. Assuming spectral representations for the latter, we first show that also the coupling obeys such a representation. The subsequent spectral reconstruction of the coupling data, obtained from 2+1 flavor lattice QCD results for the ghost and gluon, is based on a probabilistic inversion of this representation using Gaussian process regression with analytically enforced asymptotics. In contradistinction, our direct calculation relies on earlier reconstruction results for the ghost and gluon spectral functions themselves, as well as data obtained in functional QCD. Apart from its relevance for studies of resonances or scattering processes, the calculation also serves as a nontrivial benchmark of our reconstruction approach. The results show remarkable agreement, testifying to the reliability of the method.
J. Horak, J. M. Pawlowski, J. Turnwald, J. M. Urban, N. Wink, S. Zafeiropoulos, “Nonperturbative strong coupling at timelike momenta”, Phys. Rev. D 107, 076019 (2023).
https://journals.aps.org/prd/abstract/10.1103/PhysRevD.107.076019
Related to Project A02, C06
Abstract:
We investigate dynamical chiral symmetry breaking and the emergence of mesonic bound states from the infrared dynamics of four-quark scatterings. Both phenomena originate from the resonant scalar-pseudoscalar channel of the four-quark scatterings, and we compute the functional renormalisation group (fRG) flows of the Fierz-complete four-quark interaction of up and down quarks with its channel momentum dependence. This is done in the isospin symmetric case, also including the flow of the quark two-point function. This system can be understood as the fRG analogues of the complete Bethe-Salpeter equations and quark gap equation. The pole mass of the pion is determined from both direct calculations of the four-quark flows in the Minkowski regime of momenta and the analytic continuation based on results in the Euclidean regime, which are consistent with each other.
J. M. Pawlowski, W.-J. Fu, C. Huang, Y.-Y. Tan, “Four-quark scatterings in QCD I”, SciPost Phys. 14, 069 (2023).
https://scipost.org/10.21468/SciPostPhys.14.4.069
Related to Project A02
Abstract:
Low energy effective theories give access to regimes of the QCD phase diagram that to date are hard to simulate directly with lattice QCD or with functional approaches. For lattice QCD this includes the small temperature and/or large density regime. In both regimes the lower UV cutoff in low enery effective theories may soften computational problems. Moreover, lattice results for low energy effective theories serve as benchmark results for functional approaches for these effective theories. Here we present lattice results for the scalar O(4) and quark-meson models. We simulate the theory via Stochastic Quantisation and report on the effects of employing coloured noise, a method that allows control over the momentum scale of the simulation.
F. Attanasio, J. P. Klinger, J. M. Pawlowski, “Low energy effective theories on the lattice with coloured noise”, PoS LATTICE2022, 290 (2023).
Related to Project A02
Abstract:
We extend the theory of nonthermal fixed points to the case of anomalously slow universal scaling dynamics according to the sine-Gordon model. This entails the derivation of a kinetic equation for the momentum occupancy of the scalar field from a nonperturbative two-particle irreducible effective action, which resums a series of closed loop chains akin to a large-N expansion at next-to-leading order. The resulting kinetic equation is analyzed for possible scaling solutions in space and time that are characterized by a set of universal scaling exponents and encode self-similar transport to low momenta. Assuming the momentum occupancy distribution to exhibit a scaling form we can determine the exponents by identifying the dominating contributions to the scattering integral and power counting. If the field exhibits strong variations across many wells of the cosine potential, the scattering integral is dominated by the scattering of many quasiparticles such that the momentum of each single participating mode is only weakly constrained. Remarkably, in this case, in contrast to wave turbulent cascades, which correspond to local transport in momentum space, our results suggest that kinetic scattering here is dominated by rather nonlocal processes corresponding to a spatial containment in position space. The corresponding universal correlation functions in momentum and position space corroborate this conclusion. Numerical simulations performed in accompanying work yield scaling properties close to the ones predicted here.
P. Heinen, A. N. Mikheev, T. Gasenzer, “Anomalous scaling at non-thermal fixed points of the sine-Gordon model”, Phys. Rev. A 107 (2023).
https://journals.aps.org/pra/abstract/10.1103/PhysRevA.107.043303
Related to Project A04, A07
Press Release Max-Planck-Institut für Kernphysik Heidelberg
30.03.2023
Priv. Doz. Dr. Sven Sturm has been awarded one of the prestigious ERC Advanced Grants in the latest round of calls for proposals by the European Research Council. His LSym project, which will be supported with about 2.5 million Euros, aims to understand the mystery of antimatter. This is already the third successful ERC Advanced Grant application at the MPIK.
Why does our universe consist almost exclusively of matter and not antimatter? In fact, our current theories of physics state that almost identical amounts of matter and antimatter should have been created in the Big Bang. However, this seems incompatible with the observation that our universe today contains almost no antimatter anymore.
The solution to this great mystery seems to be closely related to the fundamental properties of matter and antimatter. Our Standard Model, i.e., the summary of our present knowledge of fundamental interactions, involves a deep symmetry of matter and antimatter, the so-called CPT (charge-parity-time) symmetry. Specifically, this implies that the fundamental properties of matter and antimatter, such as mass, charge, and magnetic moments, must be identical (up to the sign). It is precisely this anti-matter symmetry that Dr. Sven Sturm wants to put to the test with his novel experiment “LSym (Lepton Symmetry Experiment)”, because if it were to be violated even very slightly, this would have a very decisive impact on our understanding of physics.
To this end, the researcher and his team will, for the first time, simultaneously store a single electron and its antimatter equivalent, the positron, in a novel Penning trap and measure them with an accuracy of 14 digits – orders of magnitude more precise than was previously possible. In the strong magnetic field of this trap, the spins of both particles spin (“precess”) very rapidly around the trap’s magnetic field axis, much like microscopic gyroscopes. If for example the positron was slightly lighter than the positron, this could be detected in the experiment as a tiny difference in the rotation speed. However, to achieve the targeted accuracy, the particles must be extremely cold, namely in the quantum mechanical ground state of motion. They then form a so-called Coulomb crystal. “Developing the new technologies needed to do this will be one of the first challenges for the project, but I am confident that we will succeed,” Sturm explains. Conditions at the Max Planck Institut für Kernphysik (MPIK) in Heidelberg are ideal for us, since we can rely on years of experience with high-precision experiments in ion traps, as well as have access to excellent on-site mechanical and electrical workshops. In addition, we work closely with the theory department of the institute to accurately predict quantum electrodynamic contributions needed for a measurement,” he adds.
If successful, LSym will provide the world’s most sensitive test of matter-antimatter symmetry for leptons, helping to solve the mystery of matter/antimatter asymmetry in our universe.
Priv. Doz. Dr. Sven Sturm is a group leader in the Blaum division at the Max Planck Institut für Kernphysik. He studied physics at the University of Heidelberg before receiving his PhD with honors from Johannes Gutenberg University Mainz in 2012. Since then, he has been working as a scientist at MPIK and is responsible for several groundbreaking results in the determination of, for example, electron or proton mass.
Contact
PD Dr. Sven Sturm
MPI für Kernphysik
sven.sturm@mpi-hd.mpg.de
Tel.: +49 6221 516-447
Pressemitteilung Max-Planck-Institut für Kernphysik Heidelberg
30.03.2023
In der jüngsten Ausschreibungsrunde des European Research Council (ERC) hat Priv. Doz. Dr. Sven Sturm einen der renommierten ERC Advanced Grants mit einem Umfang von etwa 2,5 Millionen Euro eingeworben. Sein Projekt LSym möchte dem Geheimnis der Antimaterie weiter auf den Grund gehen. Am MPIK ist dies bereits der dritte erfolgreiche Antrag eines ERC Advanced Grants.
Warum besteht unser Universum fast ausschließlich aus Materie und nicht etwa aus Antimaterie? Tatsächlich besagen unsere heutigen Theorien der Physik, dass im Urknall fast identische Mengen an Materie und Antimaterie entstanden sein müssten. Dass unser Universum heute aber nahezu keine Antimaterie mehr enthält, lässt sich damit nicht erklären.
Die Lösung dieses großen Rätsels scheint eng mit den fundamentalen Eigenschaften von Materie und Antimaterie verbunden zu sein. Unser Standardmodell, also die Zusammenfassung unseres heutigen Wissens über fundamentale Wechselwirkungen, beinhaltet eine tiefe Symmetrie von Materie und Antimaterie, die sogenannte CPT (charge-parity-time) Symmetrie. Konkret bedeutet dies, dass die grundlegenden Eigenschaften, wie beispielsweise Masse, Ladung und magnetische Momente, von Materie und Antimaterie (bis auf das Vorzeichen) identisch sein müssen. Genau diese Anti-/Materie-Symmetrie möchte Dr. Sven Sturm mit seinem neuartigen Experiment „LSym (Lepton Symmetry Experiment)“ auf die Probe stellen, denn falls sie auch nur ganz geringfügig verletzt sein sollte, hätte dies ganz entscheidende Bedeutung für unser Verständnis der Physik.
Dazu werden der Forscher und sein Team jeweils ein einziges Elektron und sein Antimaterie-Äquivalent, das Positron, erstmals gleichzeitig in einer neuartigen Penningfalle speichern und auf 14 Stellen genau vermessen – Größenordnungen genauer als dies bisher möglich war. Im extrem starken Magnetfeld dieser Falle drehen sich („präzedieren“) die Spins beider Teilchen, ähnlich wie mikroskopische Kreisel, sehr schnell um die Magnetfeldachse der Falle. Falls beispielsweise das Positron geringfügig leichter sein sollte als das Elektron, könnte dies im Experiment als ein winziger Unterschied in der Drehgeschwindigkeit detektiert werden. Um die angepeilte Genauigkeit erreichen zu können müssen die Teilchen aber extrem kalt sein, nämlich im quantenmechanischen Grundzustand der Bewegung. Sie bilden dann einen sogenannten Coulomb-Kristall. „Die dazu notwendigen neuen Technologien zu entwickeln wird eine der ersten Herausforderungen für das Projekt sein, aber ich bin zuversichtlich, dass uns dies gelingen wird“, erläutert Sturm. „Am Max Planck Institut für Kernphysik (MPIK) in Heidelberg sind hierfür beste Voraussetzungen, da wir einerseits auf jahrelange Erfahrung mit Hochpräzisionsexperimenten in Ionenfallen zurück greifen können, also auch hervorragende Werkstätten vor Ort zur Verfügung haben. Zudem arbeiten wir eng mit der Theorieabteilung am Institut zusammen, um die für eine Messung nötigen Beiträge der Quantenelektrodynamik genau vorhersagen zu können“, fügt er hinzu.
Wenn dies gelingt, wird LSym den weltweit empfindlichsten Test der Materie-Antimaterie-Symmetrie für Leptonen durchführen und so zur Lösung des Rätsels der Materie/Antimaterie-Asymmetrie in unserem Universum beitragen.
Priv. Doz. Dr. Sven Sturm ist Gruppenleiter in der Abteilung Blaum am MPIK. Er studierte an der Universität Heidelberg Physik, bevor er 2012 an der Johannes Gutenberg-Universität Mainz mit Auszeichnung promovierte. Seitdem ist er als Wissenschaftler am MPIK tätig und für mehrere bahnbrechende Ergebnisse in der Bestimmung etwa der Elektronen- oder Protonenmasse verantwortlich.
Kontakt
PD Dr. Sven Sturm
MPI für Kernphysik
sven.sturm@mpi-hd.mpg.de
Tel.: +49 6221 516-447
Abstract:
The sensitivity of particle-level fiducial cross section measurements from ATLAS, CMS and LHCb to a leptophobic top-colour model is studied. The model has previously been the subject of resonance searches. Here we compare it directly to state-of-the-art predictions for Standard Model top quark production and also take into account next-to-leading order predictions for the new physics signal. We make use of the Contur framework to evaluate the sensitivity of the current measurements, first under the default Contur assumption that the measurement and the SM exactly coincide, and then using the full SM theory calculation for at next-to-leading and next-to-next-to-leading order as the background model. We derive exclusion limits, discuss the differences between these approaches, and compare to the limits from resonance searches by ATLAS and CMS.
M. M. Altakach, J. M. Butterworth, T. Jeo, M. Klasen, I. Schienbein, “Probing a leptophobic top-colour model with cross section measurements and precise signal and background predictions: a case study”, SciPost Phys. Core 6, 014 (2023).
https://scipost.org/10.21468/SciPostPhysCore.6.1.014
Related to Project C05
Abstract:
We investigate an ultracold mixture of Bose gases interacting via spin-changing collisions by studying the dynamics of spin fluctuations. The experimental implementation employs 23Na and 7Li atoms, which are prepared out of equilibrium across a wide range of initial conditions. We identify three regimes in the dynamics of the system for different initial states: a long-lived metastable regime, an instability range with strong growth of fluctuations, and a regime approaching thermal equilibrium. Theoretical modeling of the data allows us to reconstruct effective potentials which characterize the different dynamical regimes of the system.
A. Hegde, R. Ott, A. Xia, V. Kasper, J. Berges, F. Jendrzejewski, “Nonequilibrium dynamics of fluctuations in an ultracold atomic mixture”, Phys. Rev. A 107, L031302 (2023).
https://journals.aps.org/pra/abstract/10.1103/PhysRevA.107.L031302
Related to Project B04, B03, A01, C03
Abstract:
We numerically study for the first time the nonlinear GLR-MQ evolution equations for nuclear parton distribution function (nPDFs) to next-to-leading order accuracy and quantify the impact of gluon recombination at small x. Using the nCTEQ15 nPDFs as input, we confirm the importance of the nonlinear corrections for small x≲10−3, whose magnitude increases with a decrease of x and an increase of the atomic number A. We find that at x=10−5 and for heavy nuclei, after the upward evolution from Q0=2 GeV to Q=10 GeV, the quark singlet Ω(x,Q2) and the gluon G(x,Q2) distributions become reduced by 9%–15%, respectively. The relative effect is much stronger for the downward evolution from Q0=10 GeV to Q=2 GeV, where we find that Ω(x,Q2) is suppressed by 40%, while G(x,Q2) is enhanced by 140%. These trends propagate into the FA2(x,Q2) nuclear structure function and the FAL(x,Q2) longitudinal structure function, which after the downward evolution become reduced by 45% and enhanced by 80%, respectively. Our analysis indicates that the nonlinear effects are most pronounced in FAL(x,Q2) and are already quite sizable at x∼10−3 for heavy nuclei. We have checked that our conclusions very weakly depend on the choice of input nPDFs. In particular, using the EPPS21 nPDFs as input, we obtain quantitatively similar results.
J. Rausch, V. Guzey, M. Klasen, “Numerical evaluation of the nonlinear gribov-levin-ryskinmueller-qiu evolution equations for nuclear parton distribution functions”, Phys. Rev. D 107, 054003 (2023).
https://journals.aps.org/prd/abstract/10.1103/PhysRevD.107.054003
Related to Project C05
Abstract:
In einer Supraflüssigkeit können sich Anregungen ohne Reibung ausbreiten, wie man anhand von Wolken aus ultrakalten Atomen hervorragend studieren kann. In unseren Experimenten in Heidelberg ist es uns gelungen, zum ersten Mal die Koexistenz zweier sehr unterschiedlicher Supraflüssigkeiten zu realisieren und mittels neuartiger Methoden die Struktur dieser besonderen Quantenmaterie zu untersuchen.
M. Prüfer, D. Spitz, H. Strobel, “Supraflüssigkeit mit Spin”, Physik in unserer Zeit 54, (2023).
https://onlinelibrary.wiley.com/doi/full/10.1002/piuz.202370204
Related to Project B04
Abstract:
The scattering properties of spin-polarized Fermi gases are dominated by p-wave interactions. Besides their inherent angular dependence, these interactions differ from their s-wave counterparts as they also require the presence of a finite effective range in order to understand the low-energy properties of the system. In this article we examine how the shear viscosity and thermal conductivity of a three-dimensional spin-polarized Fermi gas in the normal phase depend on the effective range and the scattering volume in both the weakly and strongly interacting limits. We show that although the shear viscosity and thermal conductivity both explicitly depend on the effective range near resonance, the Prandtl number which parametrizes the ratio of momentum to thermal diffusivity does not have an explicit interaction dependence both at resonance and for weak interactions in the low-energy limit. In contrast to s-wave systems, p-wave scattering exhibits an additional resonance at weak attraction from a quasi-bound state at positive energies, which leads to a pronounced dip in the shear viscosity at specific temperatures.
J. Maki, T. Enss, “Transport in p-wave interacting Fermi gases”, Phys. Rev. A 107, 023317 (2023).
https://journals.aps.org/pra/abstract/10.1103/PhysRevA.107.023317
Related to Project C02, C03
Abstract:
We investigate the structure of confining and deconfining phases in SU(2) lattice gauge theory via persistent homology, which gives us access to the topology of a hierarchy of combinatorial objects constructed from given data. Specifically, we use filtrations by traced Polyakov loops, topological densities, holonomy Lie algebra fields, as well as electric and magnetic fields. This allows for a comprehensive picture of confinement. In particular, topological densities form spatial lumps which show signatures of the classical probability distribution of instanton-dyons. Signatures of well-separated dyons located at random positions are encoded in holonomy Lie algebra fields, following the semiclassical temperature dependence of the instanton appearance probability. Debye screening discriminating between electric and magnetic fields is visible in persistent homology and pronounced at large gauge coupling. All employed constructions are gauge-invariant without a priori assumptions on the configurations under study. This work showcases the versatility of persistent homology for statistical and quantum physics studies, barely explored to date.
D. Spitz, J. M. Urban, J. M. Pawlowski, “Confinement in non-Abelian lattice gauge theory via persistent homology”, Phys. Rev. D 107, 034506 (2023).
https://journals.aps.org/prd/abstract/10.1103/PhysRevD.107.034506
Related to Project A01, B03
Abstract:
The dynamics of correlated systems is relevant in many fields ranging from cosmology to plasma physics. However, they are challenging to predict and understand even for classical systems due to the typically large numbers of particles involved. Here, we study the evolution of an ultracold, correlated many-body system with repulsive interactions and initial correlations set by the Rydberg blockade using the analytical framework of Kinetic Field Theory (KFT). The KFT formalism is based on the path-integral formulation for classical mechanics and was first developed and successfully used in cosmology to describe structure formation in Dark Matter. The theoretical framework offers a high flexibility regarding the initial configuration and interactions between particles and, in addition, is computationally cheap. More importantly, the analytic approach allows us to gain better insight into the processes which dominate the dynamics. In this work we show that KFT can be applied in a much more general context and study the evolution of a correlated ion plasma. We find good agreement between the analytical KFT results for the evolution of the correlation function and results obtained from numerical simulations. We use the correlation functions obtained with KFT to compute the temperature increase in the ionic system due to disorder-induced heating. For certain choices of parameters we observe that the effect can be reversed, leading to correlation cooling. Due to its numerical efficiency as compared to numerical simulations, a detailed study using KFT can help to constrain parameter spaces where disorder-induced heating is minimal in order to reach the regime of strong coupling.
E. Kozlikin, R. Lilow, M. Pauly, A. Schuckert, A. Salzinger, M. Bartelmann, M. Weidemüller, “Ultracold plasmas from strongly anti-correlated Rydberg gases in the Kinetic Field Theory formalism”, arXiv:2302.01807 (2023).
https://arxiv.org/abs/2302.01807
Related to Project A05
Abstract:
We employ the equal-time formulation of quantum field theory to derive effective kinetic theories, first for a weakly coupled non-relativistic Bose gas, and then for a strongly correlated system of self-interacting -component fields. Our results provide the link between state-of-the-art measurements of equal-time effective actions using quantum simulator platforms, as employed in [Phys. Rev. X 10, 011020 (2020); Nat. Phys. 16, 1012 (2020)], and observables underlying effective kinetic or hydrodynamic descriptions. New non-perturbative approximation schemes can be developed and certified this way, where the a priori time-local formulation of the equal-time effective action has crucial advantages over the conventional closed-time-path approach which is non-local in time.
R. Ott, T. V. Zache, J. Berges, „Equal-time approach to real-time dynamics of quantum fields“,
SciPost Phys. 14, 011 (2023).
https://scipost.org/10.21468/SciPostPhys.14.2.011
Related to Project B03, B04, A03, A04, A01
Abstract:
We study the dynamics of perturbations around nonthermal fixed points associated with universal scaling phenomena in quantum many-body systems far from equilibrium. For an N-component scalar quantum field theory in 3+1 space-time dimensions, we determine the stability scaling exponents using a self-consistent large-N expansion to next-to-leading order. Our analysis reveals the presence of both stable and unstable perturbations, the latter leading to quasiexponential deviations from the fixed point in the infrared. We identify a tower of far-from-equilibrium quasiparticle states and their dispersion relations by computing the spectral function. With the help of linear response theory, we demonstrate that unstable dynamics arises from a competition between elastic scattering processes among the quasiparticle states. What ultimately renders the fixed point dynamically attractive is the phenomenon of a “scaling instability,” which is the universal scaling of the unstable regime toward the infrared due to a self-similar quasiparticle cascade. Our results provide ab initio understanding of emergent stability properties in self-organized scaling phenomena.
T. Preis, M. P. Heller, J. Berges, “Stable and Unstable Perturbations in Universal Scaling Phenomena Far from Equilibrium”, Phys. Rev. Lett. 130, 031602 (2023).
https://journals.aps.org/prl/abstract/10.1103/PhysRevLett.130.031602
Related to Project A01, A03, A04, A05, B04
Press Release Max-Planck-Institut für Kernphysik Heidelberg
11.01.2023
The Max Planck Institute for Nuclear Physics congratulates Prof. Dr. Christoph Keitel on receiving the Willis E. Lamb Award for Laser Science and Quantum Optics. The award ceremony will be held Wednesday, Jan. 11, 2023, at the Physics of Quantum Electronics (PQE) conference Winter Colloquium in Snowbird, Utah
In addition to C. Keitel, Franco Nori and Eric Van Stryland will also be awarded the prize. The award honors the American physics Nobel laureate Willis Eugene Lamb, Jr. who laid essential foundations in numerous fields of physics and technology and, above all, made groundbreaking discoveries in quantum electrodynamics with the discovery of the Lamb shift. Among previous laureates are numerous outstanding personalities in physics. Researchers well known to the Institute, such as Paul Corkum, Olga Kocharovskaya, Mikhail Lukin, Gerald Mourou, and Peter Zoller, among others, received the award in previous years.
C. Keitel is awarded the prize for his pioneering contributions to the interaction between high-power laser fields and matter. He has been head of the department “Theoretical Quantum Dynamics and Quantum Electrodynamics ” at the Max Planck Institute for Nuclear Physics since 2004.
Contact
Honorarprof. Dr. Christoph H. Keitel
MPI für Kernphysik
email: keitel@mpi-hd.mpg.de
phone: +49 6221 516-150
Pressemitteilung Max-Planck-Institut für Kernphysik Heidelberg
11.01.2023
Das Max-Planck-Institut für Kernphysik gratuliert Prof. Dr. Christoph Keitel zur Verleihung des Willis E. Lamb Award for Laser Science and Quantum Optics. Die Verleihung findet am Mittwoch, den 11. Januar 2023 im Rahmen des Winterkolloquiums der Physics-of-Quantum-Electronics-Konferenz in Snowbird, Utah, statt.
Neben C. Keitel werden auch Franco Nori und Eric Van Stryland mit dem Preis ausgezeichnet. Die Auszeichnung ist nach dem amerikanischen Physik-Nobelpreisträger Willis Eugene Lamb, Jr. benannt, der auf zahlreichen Gebieten der Physik und Technologie wesentliche Grundsteine gelegt hat und vor allem für die Entdeckung der Lamb Verschiebung wegweisend in der Quantenelektrodynamik war. Unter den bisherigen Preisträgern sind zahlreiche herausragende Persönlichkeiten der Physik. So erhielten unter anderem dem Institut gut bekannte Forscher wie Paul Corkum, Olga Kocharovskaya, Mikhail Lukin, Gerald Mourou und Peter Zoller diese Ehrung in den vergangenen Jahren.
Christoph Keitel wird in diesem Jahr für seine bahnbrechenden Beiträge zur Wechselwirkung zwischen Hochleistungs-Laserfeldern und Materie ausgezeichnet, er ist seit 2004 Direktor der Abteilung „Theoretische Quantendynamik und Quantenelektrodynamik“ am Max-Planck-Institut für Kernphysik.
Kontakt
Honorarprof. Dr. Christoph H. Keitel
MPI für Kernphysik
keitel@mpi-hd.mpg.de
Tel.: +49 6221 516-150
Abstract:
Standard lattice formulations of non-relativistic Fermi gases with two spin components suffer from a sign problem in the cases of repulsive contact interactions and attractive contact interactions
with spin imbalance. We discuss the nature of this sign problem and the applicability of stochastic quantisation with complex Langevin evolution in both cases. For repulsive interactions, we find
that the results converge, using adaptive step size scaling and a Gaussian regulator to modify the lattice action. We present results on density profiles and correlations of a harmonically trapped
system in one spatial dimension.
F. Attanasio, M. Bauer, and J. M. Pawlowski, “Density profiles and correlations of harmonically trapped ultracold fermions via complex Langevin”, PoS LATTICE2022, 363 (2023).
Related to Project C01, C02
Abstract:
Lattice gauge theories are fundamental to such distinct fields as particle physics, condensed matter or quantum information theory. The recent progress in the control of artificial quantum systems already allows for studying Abelian lattice gauge theories in tabletop experiments. However, the realization of non-Abelian models remains challenging. Here, we employ a coherent quantum control scheme to enforce non-Abelian gauge invariance, and discuss this idea in detail for a one-dimensional SU(2) lattice gauge system. We comment on how to extend our scheme to other non-Abelian gauge symmetries and higher spatial dimensions. Because of its wide applicability, the presented coherent control scheme provides a promising route for the quantum simulation of non-Abelian lattice gauge theories.
V. Kasper, T.V. Zache, F. Jendrzejewski, M. Lewenstein, E. Zohar, “Non-Abelian gauge invariance from dynamical decoupling”, Phys. Rev. D 107, (2023).
https://journals.aps.org/prd/abstract/10.1103/PhysRevD.107.014506
Related to Project B04
Abstract:
Since the achievement of quantum degeneracy in gases of chromium atoms in 2004, the experimental investigation of ultracold gases made of highly magnetic atoms has blossomed. The field has yielded the observation of many unprecedented phenomena, in particular those in which long-range and anisotropic dipole–dipole interactions (DDIs) play a crucial role. In this review, we aim to present the aspects of the magnetic quantum-gas platform that make it unique for exploring ultracold and quantum physics as well as to give a thorough overview of experimental achievements. Highly magnetic atoms distinguish themselves by the fact that their electronic ground-state configuration possesses a large electronic total angular momentum. This results in a large magnetic moment and a rich electronic transition spectrum. Such transitions are useful for cooling, trapping, and manipulating these atoms. The complex atomic structure and large dipolar moments of these atoms also lead to a dense spectrum of resonances in their two-body scattering behaviour. These resonances can be used to control the interatomic interactions and, in particular, the relative importance of contact over dipolar interactions. These features provide exquisite control knobs for exploring the few- and many-body physics of dipolar quantum gases. The study of dipolar effects in magnetic quantum gases has covered various few-body phenomena that are based on elastic and inelastic anisotropic scattering. Various many-body effects have also been demonstrated. These affect both the shape, stability, dynamics, and excitations of fully polarised repulsive Bose or Fermi gases. Beyond the mean-field instability, strong dipolar interactions competing with slightly weaker contact interactions between magnetic bosons yield new quantum-stabilised states, among which are self-bound droplets, droplet assemblies, and supersolids. Dipolar interactions also deeply affect the physics of atomic gases with an internal degree of freedom as these interactions intrinsically couple spin and atomic motion. Finally, long-range dipolar interactions can stabilise strongly correlated excited states of 1D gases and also impact the physics of lattice-confined systems, both at the spin-polarised level (Hubbard models with off-site interactions) and at the spinful level (XYZ models). In the present manuscript, we aim to provide an extensive overview of the various related experimental achievements up to the present.
L. Chomaz, I. Ferrier-Barbut, F. Ferlaino, B. Laburthe-Tolra, B. L. Lev, T. Pfau, “Dipolar physics: A review of experiments with magnetic quantum gases”, Rep. Prog. Phys 86, 026401 (2022).
https://iopscience.iop.org/article/10.1088/1361-6633/aca814
Related to Project A07
Abstract:
Achieving strong interactions in fermionic many-body systems is a major theme of research in condensed matter physics. It is well-known that interactions between fermions can be mediated through a bosonic medium, such as a phonon bath or Bose-Einstein condensate (BEC). Here, we show that such induced attraction can be resonantly enhanced when the bosonic medium is a two-component spinor BEC. The strongest interaction is achieved by tuning the boson-boson scattering to the quantum critical spinodal point of the BEC where the sound velocity vanishes. The fermion pairing gap and the superconducting critical temperature can thus be dramatically enhanced. We propose two experimental realizations of this scenario, with exciton-polariton systems in two-dimensional semiconductors and ultracold atomic Bose-Fermi mixtures.
G. Bighin, P. A. Murthy, N. Defenu, T. Enss, “Resonantly enhanced superconductivity mediated by spinor condensates”, arXiv:2212.07419 (2022).
https://arxiv.org/abs/2212.07419
Related to Project C02, C03
Abstract:
Quantitative numerical analyses of interacting dilute Bose-Einstein condensates are most often based on semiclassical approximations. Since the complex-valued field-theoretic action of the Bose gas does not offer itself to the direct application of standard Monte Carlo techniques, simulations beyond their scope by now almost exclusively rely on quantum-mechanical techniques. Here we explore an alternative approach based on a Langevin-type sampling in an extended state space, known as the complex Langevin (CL) algorithm. While the use of the CL technique has a long-standing history in high-energy physics, in particular in the simulation of QCD at finite baryon density, applications to ultracold atoms are still in their infancy. Here we examine the applicability of the CL approach for a one- and two-component, three-dimensional nonrelativistic Bose gas in thermal equilibrium, below and above the Bose-Einstein phase transition. By comparison with analytic descriptions at the Gaussian level, including Bogoliubov and Hartree-Fock theory, we find that the method allows computing reliably and efficiently observables in the regime of experimentally accessible parameters. Close to the transition, quantum corrections lead to a shift of the critical temperature which we reproduce within the numerical range known in the literature. With this work, we aim to provide a first test of CL as a potential out-of-the-box tool for the simulation of experimentally realistic situations, including trapping geometries and multicomponent (or multiple species) models.
P. Heinen, T. Gasenzer, “Complex langevin approach to interacting bose gases”, Phys. Rev. A 106, 063308 (2022).
https://journals.aps.org/pra/abstract/10.1103/PhysRevA.106.063308
Related to Project A04
Abstract:
We examine coarsening of field-excitation patterns of the sine-Gordon (SG) model, in two and three spatial dimensions, identifying it as universal dynamics near non-thermal fixed points. The SG model is relevant in many different contexts, from solitons in quantum fluids to structure formation in the universe. The coarsening process entails anomalously slow self-similar transport of the spectral distribution of excitations towards low energies, induced by the collisional interactions between the field modes. The focus is set on the non-relativistic limit exhibiting particle excitations only, governed by a Schrödinger-type equation with Bessel-function non-linearity. The results of our classical statistical simulations suggest that, in contrast to wave turbulent cascades, in which the transport is local in momentum space, the coarsening is dominated by rather non-local processes corresponding to a spatial containment in position space. The scaling analysis of a kinetic equation obtained with path-integral techniques corroborates this numerical observation and suggests that the non-locality is directly related to the slowness of the scaling in space and time. Our methods, which we expect to be applicable to more general types of models, could open a long-sought path to analytically describing universality classes behind domain coarsening and phase-ordering kinetics from first principles, which are usually modelled in a near-equilibrium setting by a phenomenological diffusion-type equation in combination with conservation laws.
P. Heinen, A. N. Mikheev, C.-M. Schmied, T. Gasenzer, “Non-thermal fixed points of universal sine-Gordon coarsening dynamics”, Dec. 2, 2022, arXiv:2212.01162 (2022).
https://arxiv.org/abs/2212.01162
Related to Project A04
Abstract:
The accurate numerical solution of partial differential equations (PDEs) is a central task in numerical analysis allowing to model a wide range of natural phenomena by employing specialized solvers depending on the scenario of application. Here, we develop a variational approach for solving PDEs governing the evolution of high dimensional probability distributions. Our approach naturally works on the unbounded continuous domain and encodes the full probability density function through its variational parameters, which are adapted dynamically during the evolution to optimally reflect the dynamics of the density. In contrast to previous works, this dynamical adaptation of the parameters is carried out using an explicit prescription avoiding iterative gradient descent. For the considered benchmark cases we observe excellent agreement with numerical solutions as well as analytical solutions for tasks that are challenging for traditional computational approaches.
M. Reh, M. Gärttner, “Variational Monte Carlo Approach to Partial Differential Equations with Neural Networks”, Mach. Learn.: Sci. Technol. 3, 04LT02 (2022).
https://iopscience.iop.org/article/10.1088/2632-2153/aca317
Related to Project A06
Abstract:
We investigate the long-range behavior of the induced Casimir interaction between two spinless heavy impurities, or polarons, in superfluid cold atomic gases. With the help of effective field theory (EFT) of a Galilean invariant superfluid, we show that the induced impurity-impurity potential at long distance universally shows a relativistic van der Waals-like attraction (∼1/r7) resulting from the exchange of two superfluid phonons. We also clarify finite temperature effects from the same two-phonon exchange process. The temperature T introduces the additional length scale cs/T with the speed of sound cs. Leading corrections at finite temperature scale as T6/r for distances r≪cs/T smaller than the thermal length. For larger distances the potential shows a nonrelativistic van der Waals behavior (∼T/r6) instead of the relativistic one. Our EFT formulation applies not only to weakly coupled Bose or Fermi superfluids but also to those composed of strongly correlated unitary fermions with a weakly coupled impurity. The sound velocity controls the magnitude of the van der Waals potential, which we evaluate for the fermionic superfluid in the BCS-BEC crossover.
K. Fujii, M. Hongo, T. Enss, “Universal van der Waals Force between Heavy Polarons in Superfluids”, Phys. Rev. Lett. 129, 233401 (2022).
https://journals.aps.org/prl/abstract/10.1103/PhysRevLett.129.233401
Related to Project C02, C03
Abstract:
We explore the advantages of a class of entanglement criteria for continuous variable systems based on the Husimi Q-distribution in scenarios with sparse experimental data. The generality of these criteria allows optimizing them for a given entangled state and experimental setting. We consider the scenario of coarse grained measurements, or finite detector resolution, where the values of the Husimi Q-distribution are only known on a grid of points in phase space, and show how the entanglement criteria can be adapted to this case. Further, we examine the scenario where experimental measurements amount to drawing independent samples from the Husimi distribution. Here, we customize our entanglement criteria to maximize the statistical significance of the detection for a given finite number of samples. In both scenarios optimization leads to clear improvements enlarging the class of detected states and the signal-to-noise ratio of the detection, respectively.
M. Gärttner, T. Haas, J. Noll, “Optimizing detection of continuous variable entanglement for limited data”, Nov. 30, 2022, arXiv:2211.17168 (2022).
https://arxiv.org/abs/2211.17168
Related to Project A06
Abstract:
In most cosmological models, rapid expansion of space marks the first moments of the Universe and leads to the amplification of quantum fluctuations1. The description of subsequent dynamics and related questions in cosmology requires an understanding of the quantum fields of the standard model and dark matter in curved spacetime. Even the reduced problem of a scalar quantum field in an explicitly time-dependent spacetime metric is a theoretical challenge2,3,4,5, and thus a quantum field simulator can lead to insights. Here we demonstrate such a quantum field simulator in a two-dimensional Bose–Einstein condensate with a configurable trap6,7 and adjustable interaction strength to implement this model system. We explicitly show the realization of spacetimes with positive and negative spatial curvature by wave-packet propagation and observe particle-pair production in controlled power-law expansion of space, using Sakharov oscillations to extract amplitude and phase information of the produced state. We find quantitative agreement with analytical predictions for different curvatures in time and space. This benchmarks and thereby establishes a quantum field simulator of a new class. In the future, straightforward upgrades offer the possibility to enter unexplored regimes that give further insight into relativistic quantum field dynamics.
C. Viermann, M. Sparn, N. Liebster, M. Hans, E. Kath, Á. Parra-López, M. Tolosa-Simeón,
N. Sánchez-Kuntz, T. Haas, H. Strobel, S. Floerchinger, M. K. Oberthaler, „Quantum field
simulator for dynamics in curved spacetime“, Nature 611, 260264 (2022).
https://www.nature.com/articles/s41586-022-05313-9
Related to Project A04, B04
Abstract:
The solution of gauge theories is one of the most promising applications of quantum technologies. Here, we discuss the approach to the continuum limit for U(1) gauge theories regularized via finite-dimensional Hilbert spaces of quantum spin-S operators, known as quantum link models. For quantum electrodynamics (QED) in one spatial dimension, we numerically demonstrate the continuum limit by extrapolating the ground state energy, the scalar, and the vector meson masses to large spin lengths S, large volume N, and vanishing lattice spacing a. By exactly solving Gauss’s law for arbitrary S, we obtain a generalized PXP spin model and count the physical Hilbert space dimension analytically. This allows us to quantify the required resources for reliable extrapolations to the continuum limit on quantum devices. We use a functional integral approach to relate the model with large values of half-integer spins to the physics at topological angle Θ=π. Our findings indicate that quantum devices will in the foreseeable future be able to quantitatively probe the QED regime with quantum link models.
T. V. Zache, M. Van Damme, J. C. Halimeh, P. Hauke, D. Banerjee, “Toward the continuum limit of a (1+1)D quantum link Schwinger model”, Phys. Rev. D 106, (2022).
https://journals.aps.org/prd/abstract/10.1103/PhysRevD.106.L091502
Related to Project B04
Abstract:
Optical atomic clocks are the most accurate measurement devices ever constructed and have found many applications in fundamental science and technology. The use of highly charged ions (HCI) as a new class of references for highest-accuracy clocks and precision tests of fundamental physics has long been motivated by their extreme atomic properties and reduced sensitivity to perturbations from external electric and magnetic fields compared with singly charged ions or neutral atoms. Here we present the realization of this new class of clocks, based on an optical magnetic-dipole transition in Ar13+. Its comprehensively evaluated systematic frequency uncertainty of 2.2 × 10−17 is comparable with that of many optical clocks in operation. From clock comparisons, we improve by eight and nine orders of magnitude on the uncertainties for the absolute transition frequency and isotope shift (40Ar versus 36Ar), respectively. These measurements allow us to investigate the largely unexplored quantum electrodynamic (QED) nuclear recoil, presented as part of improved calculations of the isotope shift, which reduce the uncertainty of previous theory by a factor of three. This work establishes forbidden optical transitions in HCI as references for cutting-edge optical clocks and future high-sensitivity searches for physics beyond the standard model.
S.A. King, L.J. Spieß, P. Micke et al., “An optical atomic clock based on a highly charged ion”, Nature 611, 43 (2022).
https://www.nature.com/articles/s41586-022-05245-4
Related to Project B01
Abstract:
Strongly interacting quantum systems subject to quenched disorder exhibit intriguing phenomena such as glassiness and many-body localization. Theoretical studies have mainly focused on disorder in the form of random potentials, while many experimental realizations naturally feature disorder in the interparticle interactions. Inspired by cold Rydberg gases, where such disorder can be engineered using the dipole blockade effect, we study a Heisenberg XXZ spin model where the disorder is exclusively due to random spin-spin couplings, arising from power-law interactions between randomly positioned spins. Using established spectral and eigenstate properties and entanglement entropy, we show that this system exhibits a localization crossover and identify strongly interacting pairs as emergent local conserved quantities in the system, leading to an intuitive physical picture consistent with our numerical results.
A. Braemer, T. Franz, M. Weidemüller, M. Gärttner, “Pair localization in dipolar systems with tunable positional disorder”, Phys. Rev. B 106, 134212 (2022).
https://journals.aps.org/prb/abstract/10.1103/PhysRevB.106.134212
Related to Project A05
Abstract:
The absolute atomic mass of 208Pb has been determined with a fractional uncertainty of 7×10−11 by measuring the cyclotron-frequency ratio R of 208Pb41+ to 132Xe26+ with the high-precision Penning-trap mass spectrometer Pentatrap and computing the binding energies EPb and EXe of the missing 41 and 26 atomic electrons, respectively, with the ab initio fully relativistic multi-configuration Dirac–Hartree–Fock (MCDHF) method. R has been measured with a relative precision of 9×10−12. EPb and EXe have been computed with an uncertainty of 9.1 eV and 2.1 eV, respectively, yielding 207.976650571(14) u (u=9.3149410242(28)×108 eV/c2) for the 208Pb neutral atomic mass. This result agrees within 1.2σ with that from the Atomic-Mass Evaluation (AME) 2020, while improving the precision by almost two orders of magnitude. The new mass value directly improves the mass precision of 14 nuclides in the region of Z = 81–84 and is the most precise mass value with A>200. Thus, the measurement establishes a new region of reference mass values which can be used e.g. for precision mass determination of transuranium nuclides, including the superheavies.
K. Kromer, C. Lyu, M. Door, P. Filianin, Z. Harman, J. Herkenhoff, W. Huang, C. H. Keitel, D. Lange, Y. N. Novikov, C. Schweiger, S. Eliseev, K. Blaum, “High-precision mass measurement of doubly magic 208 Pb”,
https://link.springer.com/article/10.1140/epja/s10050-022-00860-1
Related to Project B01, B02
Abstract:
Bose–Einstein condensates are an ideal platform to explore dynamical phenomena emerging in the many-body limit, such as the build-up of long-range coherence, superfluidity or spontaneous symmetry breaking. Here we study the thermalization dynamics of an easy-plane ferromagnet employing a homogeneous one-dimensional spinor Bose gas. We demonstrate the dynamic emergence of effective long-range coherence for the spin field and verify spin-superfluidity by experimentally testing Landau’s criterion. We reveal the structure of one massive and two massless emerging modes—a consequence of explicit and spontaneous symmetry breaking, respectively. Our experiments allow us to observe the thermalization of an easy-plane ferromagnetic Bose gas. The relevant momentum-resolved observables are in agreement with a thermal prediction obtained from an underlying microscopic model within the Bogoliubov approximation. Our methods and results are a step towards a quantitative understanding of condensation dynamics in large magnetic spin systems and the study of the role of entanglement and topological excitations for their thermalization.
M. Prüfer, D. Spitz, S. Lannig, H. Strobel, J. Berges, M. K. Oberthaler, “Condensation and thermalization of an easy-plane ferromagnet in a spinor Bose gas”, Nat. Phys. 18, 1459 (2022).
https://www.nature.com/articles/s41567-022-01779-6
Related to Project B04
Abstract:
The shift of atomic energy levels due to hadronic vacuum polarization is evaluated in a semiempirical way for hydrogenlike ions and for muonic hydrogen. A parametric hadronic polarization function obtained from experimental cross sections of e−e+ annihilation into hadrons is applied to derive an effective relativistic Uehling potential. The energy corrections originating from hadronic vacuum polarization are calculated for low-lying levels using analytical Dirac-Coulomb wave functions, as well as bound wave functions accounting for the finite nuclear size. Closed formulas for the hadronic Uehling potential of an extended nucleus as well as for the relativistic energy shift in the case of a pointlike nucleus are derived. These results are compared to existing analytic formulas from nonrelativistic theory.
S. Breidenbach, E. Dizer, H. Cakir, and Z. Harman., “Hadronic vacuum polarization correction to atomic energy levels”, Phys. Rev. A 106, 042805 (2022).
https://journals.aps.org/pra/abstract/10.1103/PhysRevA.106.042805
Related to Project B02
Abstract:
Modern day quantum simulators can prepare a wide variety of quantum states but the accurate estimation of observables from tomographic measurement data often poses a challenge. We tackle this problem by developing a quantum state tomography scheme which relies on approximating the probability distribution over the outcomes of an informationally complete measurement in a variational manifold represented by a convolutional neural network. We show an excellent representability of prototypical ground- and steady states with this ansatz using a number of variational parameters that scales polynomially in system size. This compressed representation allows us to reconstruct states with high classical fidelities outperforming standard methods such as maximum likelihood estimation. Furthermore, it achieves a reduction of the estimation error of observables by up to an order of magnitude compared to their direct estimation from experimental data.
T. Schmale, M. Reh, M. Gärttner, “Efficient quantum state tomography with convolutional neural networks”, npj Quantum Inf 8, 115 (2022).
https://www.nature.com/articles/s41534-022-00621-4
Related to Project A05, A06
Abstract:
In the presence of an electromagnetic background plane wave field, electron, positron, and photon states are not stable because electrons and positrons emit photons and photons decay into electron-positron pairs. This decay of the particle states leads to an exponential damping term in the probabilities of single nonlinear Compton scattering and nonlinear Breit-Wheeler pair production. In this paper, we investigate analytically and numerically the probabilities of nonlinear Compton scattering and nonlinear Breit-Wheeler pair production including the particle states’ decay. For this, we first compute spin- and polarization-resolved expressions of the probabilities, provide some of their asymptotic behaviors, and show that the results of the total probabilities are independent of the spin and polarization bases. Then, we present several plots of the total and differential probabilities for different pulse lengths and for different spin and polarization quantum numbers. We observe that it is crucial to take into account the damping of the states in order for the probabilities to stay always below unity, and we show that the damping factors also scale with the intensity and pulse duration of the background field. In the case of nonlinear Compton scattering, we show numerically that the total probability behaves like a Poissonian distribution in the regime where the photon recoil is negligible. In all considered cases, the kinematic conditions are such that the final particles momenta transverse to the propagation direction of the plane wave are always much smaller than the particles longitudinal momenta and the main spread of the momentum distribution on the transverse plane is along the direction of the plane wave electric field.
T. Podszus, V. Dinu, A. Di Piazza, “Nonlinear compton scattering and nonlinear breit-wheeler pair production including the damping of particle states”, Phys. Rev. D 106, 056014 (2022).
https://journals.aps.org/prd/abstract/10.1103/PhysRevD.106.056014
Related to Project B02
Press Release Max Planck Institute for Nuclear Physics Heidelberg
19. September 2022
Since 06.09.2022 Klaus Blaum is officially a full member of the Heidelberger Akademie der Wissenschaften. As director at the MPI for Nuclear Physics, he heads the division “Stored and Cooled Ions”, is currently one of the three vice-presidents of the Max Planck Society and a member of the Faculty of Physics and Astronomy at Heidelberg University. He has received numerous awards for his ground-breaking scientific work and twice won an Advanced Grant from the European Research Council. In 2019, he was admitted to the Royal Swedish Academy of Sciences as a foreign member of the Physics Class.
The Heidelberger Akademie der Wissenschaften was constituted in 1909 as the Baden Academy of Sciences. As the state academy of Baden-Württemberg, it is a society of scientists and non-university research institution, sponsors research projects, organises scientific conferences and public lecture series, and promotes young scientists. Divided into two departments, almost all scientific disciplines are represented in the Academy. Scientists from the state of Baden-Württemberg who have distinguished themselves through outstanding scientific achievements are elected as full members of the Heidelberger Akademie der Wissenschaften.
Contact
Prof. Dr. Klaus Blaum
Phone: +49 6221 516-859
Email: klaus.blaum@mpi-hd.mpg.de
Press Release Max Planck Institute for Nuclear Physics Heidelberg
19. September 2022
Since 06.09.2022 Klaus Blaum is officially a full member of the Heidelberger Akademie der Wissenschaften. As director at the MPI for Nuclear Physics, he heads the division “Stored and Cooled Ions”, is currently one of the three vice-presidents of the Max Planck Society and a member of the Faculty of Physics and Astronomy at Heidelberg University. He has received numerous awards for his ground-breaking scientific work and twice won an Advanced Grant from the European Research Council. In 2019, he was admitted to the Royal Swedish Academy of Sciences as a foreign member of the Physics Class.
The Heidelberger Akademie der Wissenschaften was constituted in 1909 as the Baden Academy of Sciences. As the state academy of Baden-Württemberg, it is a society of scientists and non-university research institution, sponsors research projects, organises scientific conferences and public lecture series, and promotes young scientists. Divided into two departments, almost all scientific disciplines are represented in the Academy. Scientists from the state of Baden-Württemberg who have distinguished themselves through outstanding scientific achievements are elected as full members of the Heidelberger Akademie der Wissenschaften.
Contact
Prof. Dr. Klaus Blaum
Phone: +49 6221 516-859
Email: klaus.blaum@mpi-hd.mpg.de
Pressemitteilung Max-Planck-Institut für Kernphysik Heidelberg
19. September 2022
Seit 06.09.2022 ist Klaus Blaum offiziell ordentliches Mitglied der Heidelberger Akademie der Wissenschaften. Als Direktor am MPI für Kernphysik leitet er die Abteilung „Gespeicherte und gekühlte Ionen“, ist derzeit einer der drei Vizepräsidenten der Max-Planck-Gesellschaft und Mitglied der Fakultät für Physik und Astronomie der Universität Heidelberg. Für seine bahnbrechenden wissenschaftlichen Arbeiten erhielt er zahlreiche Preise und gewann zweimal einen Advanced Grant des Europäischen Forschungsrats. 2019 wurde er als auswärtiges Mitglied der Physikklasse in die Königlich-Schwedische Akademie der Wissenschaften aufgenommen.
Die Heidelberger Akademie der Wissenschaften wurde 1909 als badische Akademie der Wissenschaften konstituiert. Als Landesakademie Baden-Württembergs ist sie Gelehrtengesellschaft und außeruniversitäre Forschungseinrichtung, Trägerin von Forschungsprojekten, veranstaltet wissenschaftliche Tagungen sowie öffentliche Vortragsreihen und fördert den wissenschaftlichen Nachwuchs. Untergliedert in zwei Abteilungen sind fast alle Wissenschaftsdisziplinen in der Akademie repräsentiert. Zu ordentlichen Mitgliedern der Heidelberger Akademie der Wissenschaften werden Wissenschaftlerinnen und Wissenschaftler des Landes Baden-Württemberg gewählt, die sich durch hervorragende wissenschaftliche Leistungen ausgewiesen haben.
Kontakt
Prof. Dr. Klaus Blaum
Tel.: +49 6221 516-859
E-Mail: klaus.blaum@mpi-hd.mpg.de
Pressemitteilung Max-Planck-Institut für Kernphysik Heidelberg
19. September 2022
Seit 06.09.2022 ist Klaus Blaum offiziell ordentliches Mitglied der Heidelberger Akademie der Wissenschaften. Als Direktor am MPI für Kernphysik leitet er die Abteilung „Gespeicherte und gekühlte Ionen“, ist derzeit einer der drei Vizepräsidenten der Max-Planck-Gesellschaft und Mitglied der Fakultät für Physik und Astronomie der Universität Heidelberg. Für seine bahnbrechenden wissenschaftlichen Arbeiten erhielt er zahlreiche Preise und gewann zweimal einen Advanced Grant des Europäischen Forschungsrats. 2019 wurde er als auswärtiges Mitglied der Physikklasse in die Königlich-Schwedische Akademie der Wissenschaften aufgenommen.
Die Heidelberger Akademie der Wissenschaften wurde 1909 als badische Akademie der Wissenschaften konstituiert. Als Landesakademie Baden-Württembergs ist sie Gelehrtengesellschaft und außeruniversitäre Forschungseinrichtung, Trägerin von Forschungsprojekten, veranstaltet wissenschaftliche Tagungen sowie öffentliche Vortragsreihen und fördert den wissenschaftlichen Nachwuchs. Untergliedert in zwei Abteilungen sind fast alle Wissenschaftsdisziplinen in der Akademie repräsentiert. Zu ordentlichen Mitgliedern der Heidelberger Akademie der Wissenschaften werden Wissenschaftlerinnen und Wissenschaftler des Landes Baden-Württemberg gewählt, die sich durch hervorragende wissenschaftliche Leistungen ausgewiesen haben.
Kontakt
Prof. Dr. Klaus Blaum
Tel.: +49 6221 516-859
E-Mail: klaus.blaum@mpi-hd.mpg.de
Abstract:
Phonons have the characteristic linear dispersion relation of massless relativistic particles. They arise as low-energy excitations of Bose-Einstein condensates and, in nonhomogeneous situations, are governed by a space- and time-dependent acoustic metric. We discuss how this metric can be experimentally designed to realize curved spacetime geometries, in particular, expanding Friedmann-Lemaître-Robertson-Walker cosmologies, with negative, vanishing, or positive spatial curvature. A nonvanishing Hubble rate can be obtained through a time-dependent scattering length of the background condensate. For relativistic quantum fields, this leads to the phenomenon of particle production, which we describe in detail. We explain how particle production and other interesting features of quantum field theory in curved spacetime can be tested in terms of experimentally accessible correlation functions.
Abstract:
We discuss a reformulation of QED in which matter and gauge fields are integrated out explicitly, resulting in a many-body Lorentz covariant theory of 0+1-dimensional worldlines describing superpairs of spinning charges interacting through Lorentz forces. This provides a powerful, string inspired definition of amplitudes to all-loop orders. In particular, one obtains a more general formulation of Wilson loops and lines, with exponentiated dynamical fields and spin precession contributions, and worldline contour averages exactly defined through first quantized path integrals. We discuss in detail the attractive features of this formalism for high order perturbative computations. We show that worldline S-matrix elements, to all-loop orders in perturbation theory, can be constructed to be manifestly free of soft singularities, with infrared (IR) divergences captured and removed by endpoint photon exchanges at infinity that are equivalent to the soft coherent dressings of the Dyson S-matrix proposed by Faddeev and Kulish. We discuss these IR structures and make connections with soft theorems, the Abelian exponentiation of IR divergences and cusp anomalous dimensions. Follow-up papers will discuss the efficient computation of cusp anomalous dimensions and universal features of soft theorems.
X. Feal, A. Tarasov, R. Venugopalan, “QED as a many-body theory of worldlines: General formalism and infrared structure”, Phys. Rev. D 106, 056009 (2022).
https://journals.aps.org/prd/abstract/10.1103/PhysRevD.106.056009
Related to Project B03
Abstract:
The mobile impurity in a Bose-Einstein condensate (BEC) is a paradigmatic many-body problem. For weak interaction between the impurity and the BEC, the impurity deforms the BEC only slightly and it is well described within the Fröhlich model and the Bogoliubov approximation. For strong local attraction this standard approach, however, fails to balance the local attraction with the weak repulsion between the BEC particles and predicts an instability where an infinite number of bosons is attracted toward the impurity. Here we present a solution of the Bose polaron problem beyond the Bogoliubov approximation which includes the local repulsion between bosons and thereby stabilizes the Bose polaron even near and beyond the scattering resonance. We show that the Bose polaron energy remains bounded from below across the resonance and the size of the polaron dressing cloud stays finite. Our results demonstrate how the dressing cloud replaces the attractive impurity potential with an effective many-body potential that excludes binding. We find that at resonance, including the effects of boson repulsion, the polaron energy depends universally on the effective range. Moreover, while the impurity contact is strongly peaked at positive scattering length, it remains always finite. Our solution highlights how Bose polarons are self-stabilized by repulsion, providing a mechanism to understand quench dynamics and nonequilibrium time evolution at strong coupling.
R. Schmidt and T. Enss, “Self-stabilized bose polarons”, SciPost Phys. 13, 054 (2022).
https://scipost.org/SciPostPhys.13.3.054
Related to Project C02, C03
Abstract:
In this paper, we report on the development of a fast high-voltage switch, which is based on two enhancement mode N-channel silicon carbide metal–oxide–semiconductor field-effect transistors in push–pull configuration. The switch is capable of switching high voltages up to 600 V on capacitive loads with rise and fall times on the order of 10 ns and pulse widths ≥20 ns. Using this switch, it was demonstrated that, from the charge state distribution of bunches of highly charged ions ejected from an electron beam ion trap with a specific kinetic energy, single charge states can be separated by fast switching of the high voltage applied to a Bradbury–Nielsen Gate with a resolving power of about 100.
C. Schweigera, M. Door, P. Filianin, J. Herkenhoff, K. Kromer, D. Lange, D. Marschall, A. Rischka, T. Wagner, S. Eliseev, K. Blaum: “Fast silicon carbide MOSFET based high-voltage push–pull switch for charge state separation of highly charged ions with a Bradbury–Nielsen gate”, Rev. Sci. Instrum. 93, (2022).
https://aip.scitation.org/doi/pdf/10.1063/5.0083515
Related to Project B01
Abstract:
Lattice simulations along with studies in continuum QCD indicate that non-perturbative quantum fluctuations lead to an infrared regularisation of the gluon propagator in covariant gauges in the form of an effective mass-like behaviour. In the present work we propose an analytic understanding of this phenomenon in terms of gluon condensation through a dynamical version of the Higgs mechanism, leading to the emergence of color condensates. Within the functional renormalisation group approach we compute the effective potential of covariantly constant field strengths, whose non-trivial minimum is related to the color condensates. In the physical case of an SU(3) gauge group this is an octet condensate. The value of the gluon mass obtained through this procedure compares very well to lattice results and the mass gap arising from alternative dynamical scenarios.
J. Horak, F. Ihssen, J. Papavassiliou, J. M. Pawlowski, A. Weber, C. Wetterich, “Gluon condensates and effective gluon mass”, SciPost Phys. 13, (2022).
https://scipost.org/10.21468/SciPostPhys.13.2.042
Related to Project A02, C06
Abstract:
We present a phenomenological analysis of events with two high transverse momentum (pT) jets separated by a large (pseudo-)rapidity interval void of particle activity, also known as jet-gap-jet events. In the limit where the collision energy s√ is much larger than any other momentum scale, the jet-gap-jet process is described in terms of perturbative pomeron exchange between partons within the Balitsky-Fadin-Kuraev-Lipatov (BFKL) limit of perturbative quantum chromodynamics (QCD). The BFKL pomeron exchange amplitudes, with resummation at the next-to-leading logarithmic approximation, have been embedded in the PYTHIA8 Monte Carlo event generator. Standard QCD dijet events are simulated at next-to-leading order in αs matched to parton showers with POWHEG+PYTHIA8. We compare our calculations to measurements by the CDF, D0, and CMS experiments at center-of-mass energies of 1.8, 7 and 13 TeV. The impact of the theoretical scales, the parton densities, final- and initial-state radiation effects, multiple parton interactions, and pT thresholds and multiplicities of the particles in the rapidity gap on the jet-gap-jet signature is studied in detail. With a strict gap definition (no particle allowed in the gap), the shapes of most distributions are well described except for the CMS azimuthal-angle distribution at 13 TeV. The survival probability is surprisingly well modelled by multiparton interactions in PYTHIA8. Without multiparton interactions, theoretical predictions based on two-channel eikonal models agree qualitatively with fits to the experimental data.
C. Baldenegro, P. Gonzalez Duran, M. Klasen, C. Royon, J. Salomon, “Jets separated by a large pseudorapidity gap at the Tevatron and at the LHC”, J. High Energ. Phys. 2022, 250 (2022).
https://doi.org/10.1007/JHEP08(2022)250
Related to Project C05
International Conference: Quantum Systems in Extreme Conditions (QSEC2022)
13-18 Nov 2022, NH Hotel Bingen
REGISTRATION NOW OPEN!
https://indico.physi.uni-heidelberg.de/event/504/
The understanding of quantum systems in extreme conditions requires the resolution of outstanding questions, relevant for a wide range of topical applications from particle and nuclear physics to atomic and condensed matter physics. Many such systems exhibit characteristic common properties despite dramatic differences in key parameters such as temperature, density, field strength and others. The existence of universal regimes, where even quantitative agreements between seemingly disparate physical systems can be observed, drives a remarkable convergence of research activities across traditional lines of specialisation. In turn, the identification and investigation of non-universal properties plays a vital role for an improved under-
standing of fundamental differences between the systems.
The International Conference on Quantum Systems in Extreme Conditions (QSEC2022) brings together experimental and theoretical researchers working on topics that are particularly driven by such cross-disciplinary developments, in particular:
- Far from equilibrium dynamics and thermalisation
- Quantum systems with strong fields
- Phase structure, large fluctuations and quantum critical phenomena
Keynote talks will provide overviews about quantum systems in extreme conditions in heavy ion collisions, precision spectroscopy in highly charged ions, and ultracold quantum gases. Contributed talks are dedicated to recent progress in these systems. Abundant discussion time and two poster sessions are foreseen for the vital interactions between experimental and theoretical physicists working in the different areas.
Keynote Speakers
Laura Fabbietti (Germany)
Thierry Giamarchi (Switzerland)
Gianluca Gregori (United Kingdom)
Rudi Grimm (Austria)
Marianna Safronova (USA)
Dam Thanh Son (USA)
Invited speakers
Jasmine Brewer (Switzerland)
Raphaelle Bailhache (Germany)
David Clément (France)
Alina Czajka (Poland)
Elina Fuchs (Germany)
Felix Karbstein (Germany)
Esther Menz (Germany)
Julian Schmitt (Germany)
Giulia Semeghini (USA, tbc)
Tapio Simula (Australia)
Federica Surace (USA)
Xiaojun Yao (USA)
Abstract:
A fluid-dynamic approach to the diffusion of heavy quarks in the quark-gluon plasma (QGP) is presented. Specifically, we analyze the Fokker-Planck equation for the momentum transport of heavy quarks from a fluid perspective and use a mapping to second-order fluid dynamics to determine conductivities and relaxation times governing their spatial diffusion. By investigating the relation between the two approaches, we provide new insights concerning the level of local thermalization of charm and bottom quarks inside the expanding QGP. Our results indicate that a fluid-dynamic description of diffusion is feasible for charm quarks at least for the latest stages of the fireball evolution.
F. Capellino, A. Beraudo, A. Dubla, S. Floerchinger, S. Masciocchi, J. Pawlowski, I. Selyuzhenkov, “Fluid-dynamic approach to heavy-quark diffusion in the quark-gluon plasma”, Phys. Rev. D 106, 034021 (2022).
https://journals.aps.org/prd/abstract/10.1103/PhysRevD.106.034021
Related to Project A02, A01
Abstract:
Recent research has demonstrated the usefulness of neural networks as variational ansatz functions for quantum many-body states. However, high-dimensional sampling spaces and transient autocorrelations confront these approaches with a challenging computational bottleneck. Compared to conventional neural networks, physical-model devices offer a fast, efficient and inherently parallel substrate capable of related forms of Markov chain Monte Carlo sampling. Here, we demonstrate the ability of a neuromorphic chip to represent the ground states of quantum spin models by variational energy minimization. We develop a training algorithm and apply it to the transverse field Ising model, showing good performance at moderate system sizes (N≤10). A systematic hyperparameter study shows that scalability to larger system sizes mainly depends on sample quality, which is limited by temporal parameter variations on the analog neuromorphic chip. Our work thus provides an important step towards harnessing the capabilities of neuromorphic hardware for tackling the curse of dimensionality in quantum many-body problems.
R. Klassert, A. Baumbach, M. A. Petrovici, M. Gärttner, “Variational learning of quantum ground states on spiking neuromorphic hardware”, iScience 25, 104707 (2022).
https://www.sciencedirect.com/science/article/pii/S2589004222009798?via%3Dihub
Related to Project A05, A06
Abstract:
We study Floquet engineering of the tunnel coupling between a pair of one-dimensional bosonic quasicondensates in a tilted double-well potential. By modulating the energy difference between the two wells, we reestablish tunnel coupling and precisely control its amplitude and phase. This allows us to initiate coherence between two initially uncorrelated Bose gases and prepare different initial states in the emerging sine-Gordon Hamiltonian. We fully characterize the Floquet system and study the dependence of both equilibrium properties and relaxation on the modulation.
S.-C. Ji, T. Schweigler, M. Tajik, F. Cataldini, J. Sabino, F. S. Møller, S. Erne, J. Schmiedmayer, “Floquet engineering a bosonic josephson junction”, Phys. Rev. Lett. 129, 080402 (2022).
https://journals.aps.org/prl/abstract/10.1103/PhysRevLett.129.080402
Related to Project A03
Abstract:
Understanding how closed quantum systems dynamically approach thermal equilibrium presents a major unresolved problem in statistical physics. Generically, it is expected that non-integrable quantum systems thermalize as they comply with the Eigenstate Thermalization Hypothesis (ETH)}. A notable exception to this is the phenomenon of many-body localization, where the emergence of local conserved quantities prevents thermalization, which has been observed in finite low-dimensional systems. We study an ensemble of Heisenberg spins with a tunable distribution of random coupling strengths realized by a Rydberg quantum simulator. The total magnetization as a function of external field after a quench serves as a probe for thermalization. We find that such an isolated quantum system exhibits a non-thermalizing regime despite being non-integrable. It is shown that thermalization can be restored by reducing the disorder in the coupling strengths. As our system consists of up to 4000 spins, we thus show that closed quantum systems can fail to reach thermal equilibrium even at system sizes approaching the thermodynamic limit.
T. Franz, S. Geier, C. Hainaut, A. Signoles, N. Thaicharoen, A. Tebben, A. Salzinger, A. Braemer, M. Gärttner, G. Zürn, M. Weidemüller, “Absence of thermalization in an interacting system of thousands of quantum spins”, arXiv:2207.14216 (2022).
https://arxiv.org/abs/2207.14216
Related to Project A05
Abstract:
Light-matter interaction is well understood on the single-atom level and routinely used to manipulate atomic gases. However, in denser ensembles, collective effects emerge that are caused by light-induced dipole-dipole interactions and multiple photon scattering. Here, we report on the observation of a mechanical deformation of a cloud of ultracold 87Rb atoms due to the collective interplay of the atoms and a homogenous light field. This collective light scattering results in a self-confining potential with interesting features: It exhibits nonlocal properties, is attractive for both red- and blue-detuned light fields, and induces a remarkably strong force that depends on the gradient of the atomic density. Our experimental observations are discussed in the framework of a theoretical model based on a local-field approach for the light scattered by the atomic cloud. Our study provides a new angle on light propagation in high-density ensembles and expands the range of tools available for tailoring interactions in ultracold atomic gases.
M. Maiwöger, M. Sonnleitner, T. Zhang, I. Mazets, M. Mallweger, D. Rätzel, F. Borselli, S. Erne, J. Schmiedmayer, P. Haslinger, “Observation of Light-Induced Dipole-Dipole Forces in Ultracold Atomic Gases”, Phys. Rev. X 12, 031018 (2022).
https://journals.aps.org/prx/abstract/10.1103/PhysRevX.12.031018
Related to Project A03
Abstract:
Calculating the dynamics of gauge theories, which underlie some of the most successful models in physics, is extremely challenging for classical computers. An alternative to computation is quantum simulation using tunable physical systems in which gauge symmetry constraints can be effectively implemented. Zhou et al. studied the thermalization of a U(1)-symmetric gauge theory using cold bosonic atoms trapped in a tilted staggered optical lattice. The system’s evolution depended on whether the gauge constraint was enforced. Additionally, different gauge-symmetric initial states with the same energy density evolved to the same thermal state.
Z.-Y. Zhou, G.-X. Su, J. C. Halimeh, R. Ott, H. Sun, P. Hauke, B. Yang, Z.-S. Yuan, J. Berges, J.-W. Pan, “Thermalization dynamics of a gauge theory on a quantum simulator”, Science 377 (2022).
https://www.science.org/doi/10.1126/science.abl6277
Related to Project B04, A01, A02, C06
Abstract:
The nuclear parton distribution functions (nPDFs) of gluons are known to be difficult to determine with fits of deep inelastic scattering (DIS) and Drell-Yan (DY) data alone. Therefore, the nCTEQ15 analysis of nuclear PDFs added inclusive neutral pion production data from RHIC to help in constraining the gluon. In this analysis, we present a new global analysis of nuclear PDFs based on a much larger set of single inclusive light hadron data from RHIC and the LHC. Using our new nCTEQ code (nCTEQ++) with an optimized version of INCNLO we study systematically the limitations of the theory and the impact of the fragmentation function uncertainty.
P. Duwentäster, L. A. Husová, T. Jeo, M. Klasen, K. Kovaík, A. Kusina, K. F. Muzakka, F. I. Olness, I. Schienbein, J.Y. Yu, “Constraining the nuclear gluon PDF with inclusive hadron production data ”, SciPost Phys Proc. 8, 045 (2022).
https://scipost.org/10.21468/SciPostPhysProc.8.045
Related to Project C05
Abstract:
Many-body systems driven out of equilibrium can exhibit scaling flows of the quantum state. For a sudden quench to resonant interactions between particles we construct a class of analytical scaling solutions for the time evolved wave function with a complex scale parameter. These solutions determine the exact dynamical scaling of observables such as the pair correlation function, the contact and the fidelity. We give explicit examples of the nonequilibrium dynamics for two trapped fermions or bosons quenched to unitarity, for ideal Bose polarons, and for resonantly interacting, Borromean three-body systems. These solutions reveal universal scaling properties of interacting many-body systems that arise from the buildup of correlations at short times after the quench.
T. Enss, N. Cuadra Braatz, G. Gori, “Complex scaling flows in the quench dynamics of interacting particles”, Phys. Rev. A 106, 013308 (2022).
https://journals.aps.org/pra/abstract/10.1103/PhysRevA.106.013308
Related to Project C02, C03
Abstract:
We use the Hamiltonian formulation of kinetic theory to perform a stability analysis of nonthermal fixed points in a non-Abelian plasma. We construct a perturbative expansion of the Fokker-Planck collision kernel in an adiabatic approximation and show that the (next-to-)leading order solutions reproduce the known nonthermal fixed point scaling exponents. Working at next-to-leading order, we derive the stability equations for scaling exponents and find the relaxation rate to the nonthermal fixed point. This approach provides the basis for an understanding of the prescaling phenomena observed in QCD kinetic theory and nonrelativistic Bose gas systems.
A. Mikheev, A. Mazeliauskas, J. Berges, “Stability analysis of nonthermal fixed points in longitudinally expanding kinetic theory”, Phys. Rev. D 105, 116025 (2022).
https://journals.aps.org/prd/abstract/10.1103/PhysRevD.105.116025
Related to Project A01, B04, A03, A04
Abstract:
Using dual theories embedded into a larger unphysical Hilbert space along entanglement cuts, we study the entanglement structure of Z2 lattice gauge theory in (2+1) spacetime dimensions. We demonstrate Li and Haldane’s conjecture, and show consistency of the entanglement Hamiltonian with the Bisognano-Wichmann theorem. Studying nonequilibrium dynamics after a quench, we provide an extensive description of thermalization in Z2 gauge theory which proceeds in a characteristic sequence: Maximization of the Schmidt rank and spreading of level repulsion at early times, self-similar evolution with scaling coefficients α=0.8±0.2 and β=0.0±0.1 at intermediate times, and finally thermal saturation of the von Neumann entropy.
N. Müller, T.V. Zache, R. Ott, “Thermalization of Gauge Theories from their Entanglement Spectrum”, Phys. Rev. Lett. 129, 011601 (2022).
https://journals.aps.org/prl/abstract/10.1103/PhysRevLett.129.011601
Related to Project B03, A06
Abstract:
The solution of the Dirac equation in the presence of an arbitrary plane wave, corresponding to the so-called Volkov states, has provided an enormous insight in strong-field QED. In [Phys. Rev. A \textbf{103}, 076011 (2021)] a new “fully quasiclassical” representation of the Volkov states has been found, which is equivalent to the one known in the literature but which more transparently shows the quasiclassical nature of the quantum dynamics of an electron in a plane-wave field. Here, we derive the corresponding expression of the propagator by constructing it using the fully quasiclassical form of the Volkov states. The found expression allows one, together with the fully quasiclassical expression of the Volkov states, to compute probabilities in strong-field QED in an intense plane wave by manipulating only 2-by-2 rather than 4-by-4 Dirac matrices as in the usual approach. Moreover, apart from the exponential functions featuring the classical action of an electron in a plane wave, the fully quasiclassical Volkov propagator only depends on the electron kinetic four-momentum in the plane wave, which is a gauge-invariant quantity. Finally, we also compute the tadpole diagram in a plane wave starting from the Volkov propagator and we show that, although it is divergent, its contribution can be always absorbed via a renormalization of the external field.
A. Di Piazza, F. P. Fronimos: “Quasiclassical representation of the Volkov propagator and the tadpole diagram in a plane wave”, arXiv:2201.08101 (2022).
https://journals.aps.org/prd/abstract/10.1103/PhysRevD.105.116019
Related to Project B02
Abstract:
We present a new Monte Carlo event generator for the hadronic production of four top quarks in the POWHEG BOX framework. Besides the dominant next-to-leading order QCD corrections at O(α5s) we also include all subleading electroweak productions channels at leading-order accuracy. We validate our theoretical predictions by comparing to parton-shower matched predictions obtained within the MC@NLO framework for stable top quarks. Furthermore, we investigate in detail the various sources of theoretical uncertainties. Finally, we investigate a single lepton plus jets signature to study for the first time the impact of the electroweak production modes as well as spin-correlation effects at the fiducial level.
T. Ježo and M. Kraus, “Hadroproduction of four top quarks in POWHEG BOX”, Phys. Rev. D 105, 114024 (2022).
https://journals.aps.org/prd/abstract/10.1103/PhysRevD.105.114024
Related to Project C05
Abstract:
Quantum electrodynamics (QED) is one of the most fundamental theories of physics and has been shown to be in excellent agreement with experimental results. In particular, measurements of the electron’s magnetic moment (or g factor) of highly charged ions in Penning traps provide a stringent probe for QED, which allows testing of the standard model in the strongest electromagnetic fields. When studying the differences between isotopes, many common QED contributions cancel owing to the identical electron configuration, making it possible to resolve the intricate effects stemming from the nuclear differences. Experimentally, however, this quickly becomes limited, particularly by the precision of the ion masses or the magnetic field stability. Here we report on a measurement technique that overcomes these limitations by co-trapping two highly charged ions and measuring the difference in their g factors directly. We apply a dual Ramsey-type measurement scheme with the ions locked on a common magnetron orbit, separated by only a few hundred micrometres, to coherently extract the spin precession frequency difference. We have measured the isotopic shift of the bound-electron g factor of the isotopes 20Ne9+ and 22Ne9+ to 0.56-parts-per-trillion (5.6 × 10−13) precision relative to their g factors, an improvement of about two orders of magnitude compared with state-of-the-art techniques. This resolves the QED contribution to the nuclear recoil, accurately validates the corresponding theory and offers an alternative approach to set constraints on new physics.ly extended many-body systems.
T. Sailer, V. Debierre, Z. Harman, F. Heiße, C. KÖnig, J. Morgner, B. Tu, A.V. Volotka, C. H. Keitel, K. Blaum, S. Sturm: “Measurement of the bound-electron g-factor difference in coupled ions”, Nature 606, 479-483 (2022).
https://www.nature.com/articles/s41586-022-04807-w
Related to Project B01, B02
Abstract:
Pairing is the fundamental requirement for fermionic superfluidity and superconductivity. To understand the mechanism behind pair formation is an ongoing challenge in the study of many strongly correlated fermionic systems. Cooper pairs are the key ingredient to BCS theory as the microscopic explanation of conventional superconductivity. They form between particles of opposite spin and momentum at the Fermi surface of the system. Here, we directly observe Cooper pairs in a mesoscopic two-dimensional Fermi gas. We apply an imaging scheme that enables us to extract the full in-situ momentum distribution of a strongly interacting Fermi gas with single particle and spin resolution. Our ultracold gas allows us to freely tune between a completely non-interacting, unpaired system and weak attractions, where we find Cooper pair correlations at the Fermi surface. When increasing the attractive interactions even further, the pairs gradually turn into deeply bound molecules breaking up the Fermi surface. Our mesoscopic system is closely related to the physics of nuclei, superconducting grains or quantum dots. With the precise control over interactions, particle number and potential landscape in our experiment, the observables we establish in this work provide a new approach to longstanding questions concerning not only such mesoscopic systems but also their connection to the macroscopic world.
M. Holten, L. Bayha, K. Subramanian, S. Brandstetter, C. Heintze, P. Lunt, P. M. Preiss, S. Jochim: ” Observation of Cooper Pairs in a Mesoscopic 2D Fermi Gas”, Nature 606, 287-291 (2022).
https://www.nature.com/articles/s41586-022-04678-1
Related to Project A05, C01, ABC
Abstract
We discuss a reformulation of QED in which matter and gauge fields are integrated out explicitly, resulting in a many-body Lorentz covariant theory of 0+1 dimensional worldlines describing super-pairs of spinning charges interacting through Lorentz forces. This provides a powerful, string inspired definition of amplitudes to all loop orders. In particular, one obtains a more general formulation of Wilson loops and lines, with exponentiated dynamical fields and spin precession contributions, and worldline contour averages exactly defined through first quantized path integrals. We discuss in detail the attractive features of this formalism for high order perturbative computations. We show that worldline S-matrix elements, to all loop orders in perturbation theory, can be constructed to be manifestly free of soft singularities, with infrared (IR) divergences captured and removed by endpoint photon exchanges at infinity that are equivalent to the soft coherent dressings of the Dyson S-matrix proposed by Faddeev and Kulish. We discuss these IR structures and make connections with soft theorems, the Abelian exponentiation of IR divergences and cusp anomalous dimensions. Follow-up papers will discuss the efficient computation of cusp anomalous dimensions and universal features of soft theorems.
X. Feal, A. Tarasov, R. Venugopalan, “QED as a many-body theory of worldlines: I. General formalism and infrared structure”, arXiv:2206.04188 (2022)
https://arxiv.org/abs/2206.04188
Related to Project A01
Abstract:
In covariant gauges, the gluonic mass gap in Yang-Mills theory manifests itself in the basic observation that the massless pole in the perturbative gluon propagator disappears in nonperturbative calculations, but the origin of this behavior is not yet fully understood. We summarize a recent study of the respective dynamics with Dyson-Schwinger equations in Landau-gauge Yang-Mills theory. We identify the parameter that distinguishes the massive Yang-Mills regime from the massless decoupling solutions, whose endpoint is the scaling solution. Similar to the PT-BFM scheme, we find evidence that mass generation in the transverse sector is triggered by longitudinal massless poles.
G. Eichmann, J. M. Pawlowski, “Studying mass generation for gluons”, SciPost Phys. Proc. 6, 018 (2022).
https://scipost.org/SciPostPhysProc.6.018
Related to Project A02
Abstract:
Generalized Hydrodynamics (GHD) has recently been devised as a method to solve the dynamics of integrable quantum many-body systems beyond the mean-field approximation. In its original form, a major limitation is the inability to predict equal-time correlations. Here we present a new method to treat thermal fluctuations of a 1D bosonic degenerate gas within the GHD framework. We show how the standard results using the thermodynmaic Bethe ansatz can be obtained through sampling of collective bosonic excitations, revealing the connection or duality between GHD and effective field theories such as the standard hydrodynamic equations. As an example, we study the damping of a coherently excited density wave and show how equal-time phase correlation functions can be extracted from the GHD evolution. Our results present a conceptually new way of treating fluctuations beyond the linearized regime of GHD.
F. Møller, S. Erne, N. J. Mauser, J. Schmiedmayer, I. E. Mazets, “Bridging Effective Field Theories and Generalized Hydrodynamics”, arXiv:2205.15871 (2022).
https://arxiv.org/abs/2205.15871
Related to Project A03
Abstract:
Motivated by the possibility to use Bose-Einstein condensates as quantum simulators for spacetime curvature, we study a massless relativistic scalar quantum field in spatially curved Friedmann-Lemaître-Robertson-Walker universes with d=2+1 spacetime dimensions. In particular, we investigate particle production caused by a time-dependent background geometry, by means of the spectrum of fluctuations and several two-point field correlation functions. We derive new analytical results for several expansion scenarios.
N. Sánchez-Kuntz, A. Parra-López, M. Tolosa-Simeón, T. Haas, S. Floerchinger, “Scalar quantum fields in cosmologies with 2+1 spacetime dimensions”, Phys. Rev. D 105, 105020 (2022).
https://journals.aps.org/prd/abstract/10.1103/PhysRevD.105.105020
Related to Project A06, C06
Abstract:
In this talk, we discuss real-time thermalization dynamics of Z2 Lattice Gauge Theory in 2+1 spacetime dimensions. While classical thermalization is commonly associated with chaotic behavior, turbulence and universality, the manifestation of these phenomena in quantum mechanical systems is not clear. However, when viewed through the lens of Entanglement Structure, we find that quantum thermalization proceeds in characteristic stages and reveals phenomena remarkably similar to their classical counterparts: chaos, turbulence and universality.
N. Müller, T. V. Zache, R. Ott, “Quantum thermalization of gauge theories: chaos, turbulence and universality”, PoS LATTICE2021, 441 (2022).
Conference Proceedings: The 38th International Symposium on Lattice Field Theory, LATTICE2021, 26th-30th July 2021, Zoom/Gather@Massachusetts Institute of Technology PoS(LATTICE2021)and universality”, arXiv:2111.01155 (2021).
Related to Project B03, B04
Abstract:
We investigate macroscopic behaviors of fluctuating domain walls in nonequilibrium open systems with the help of the effective field theory based on symmetry. Since the domain wall in open systems breaks the translational symmetry, there appears a gapless excitation identified as the Nambu-Goldstone (NG) mode, which shows the non-propagating diffusive behavior in contrast to those in closed systems. After demonstrating the presence of the diffusive NG mode in the -dimensional dissipative Josephson junction, we provide a symmetry-based general analysis for open systems breaking the one-dimensional translational symmetry. A general effective Lagrangian is constructed based on the Schwinger-Keldysh formalism, which supports the presence of the gapless diffusion mode in the fluctuation spectrum in the thin wall regime. Besides, we also identify a term peculiar to the open system, which possibly leads to the instability in the thick-wall regime or the nonlinear Kardar-Parisi-Zhang coupling in the thin-wall regime although it is absent in the Josephson junction.
T. Ježo and M. Kraus, “Hadroproduction of four top quarks in POWHEG BOX”, Phys. Rev. D 105, 114024 (2022).
https://scipost.org/10.21468/SciPostPhys.12.5.160
Related to Project C05
Abstract:
We study the effects of strong inter-particle interaction on diffraction of a Bose-Einstein condensate of 6Li2 molecules from a periodic potential created by pulses of a far detuned optical standing wave. For short pulses we observe the standard Kapitza-Dirac diffraction, with the contrast of the diffraction pattern strongly reduced for very large interactions due to interaction dependent loss processes. For longer pulses diffraction shows the characteristic for matter waves impinging on an array of tubes and coherent channeling transport. We observe a slowing down of the time evolution governing the population of the momentum modes caused by the strong atom interaction. A simple physical explanation of that delay is the phase shift caused by the self interaction of the forming mater wave patters inside the standing light wave. The phenomenon can be reproduced with one-dimensional mean-field simulation. In addition two contributions to interaction-dependent degradation of the coherent diffraction patterns were identified: (i) in-trap loss of molecules during the lattice pulse, which involves dissociation of Feshbach molecules into free atoms, as confirmed by radio-frequency spectroscopy and (ii) collisions between different momentum modes during separation. This was confirmed by interferometrically recombining the diffracted momenta into the zero-momentum peak, which consequently removed the scattering background.
Q. Liang, C. Li, S. Erne, P. Paranjape, R. Wu, J. Schmiedmayer, “Diffraction of strongly interacting molecular Bose-Einstein condensate from standing wave light pulses”, SciPost Phys.12, 154 (2022)
https://scipost.org/10.21468/SciPostPhys.12.5.154
Related to Project A03
Abstract:
In global analyses of nuclear parton distribution functions (nPDFs), neutrino deep-inelastic scattering (DIS) data have been argued to exhibit tensions with the data from charged-lepton DIS. Using the nCTEQ framework, we investigate these possible tensions both internally and with the data sets used in our recent nPDF analysis nCTEQ15WZSIH. We take into account nuclear effects in the calculation of the deuteron structure function FD2 using the CJ15 analysis. The resulting nPDF fit, nCTEQ15WZSIHdeut, serves as the basis for our comparison with inclusive neutrino DIS and charm dimuon production data. Using χ2 hypothesis testing, we confirm evidence of tensions with these data and study the impact of the proton PDF baseline as well as the treatment of data correlation and normalization uncertainties. We identify the experimental data and kinematic regions that generate the tensions and present several possible approaches how a consistent global analysis with neutrino data can be performed. We show that the tension can be relieved using a kinematic cut at low x (x>0.1) and also investigate a possibility of managing the tensions by using uncorrelated systematic errors. Finally, we present a different approach identifying a subset of neutrino data which leads to a consistent global analysis without any additional cuts. Understanding these tensions between the neutrino and charged-lepton DIS data is important not only for a better flavor separation in global analyses of nuclear and proton PDFs, but also for neutrino physics and for searches for physics beyond the Standard Model.
K.F.Muzakka, P.Duwentäster, T.J.Hobbs, T.Ježo, M.Klasen, K.Kovařík, A.Kusina, J.G.Morfín, F.I.Olness, R.Ruiz, I.Schienbein, J.Y.Yu, “Compatibility of Neutrino DIS Data and Its Impact on Nuclear Parton Distribution Functions”, arXiv:2204.13157 (2022).
https://arxiv.org/abs/2204.13157
Related to Project C05
Abstract:
Collective phenomena in quantum many-body systems are often described in terms of hydrodynamics, an appropriate framework when the involved particle numbers are effectively macroscopic. We propose to use experiments on expanding clouds of few and many interacting cold atoms to investigate the emergence of hydrodynamics as a function of particle number. We consider gases confined in two-dimensional elliptically deformed traps, and we employ the manifestation of elliptic flow as an indicator of collective behavior. We quantify the response of the gas to the deformation of the trapping potential, and show how such information can be used to establish how many atoms are needed for the system to develop a degree of collectivity comparable to that expected in the hydrodynamic limit. This method permits one, in particular, to exploit observations made in expanding atomic gases to shed light on the apparent hydrodynamic behavior of mesoscopic systems of quarks and gluons formed in the scattering of light ions in high-energy collider experiments.
S. Floerchinger, G. Giacalone, L. H. Heyen, L. Tharwat, „Qualifying collective behavior in
expanding ultracold gases as a function of particle number“, Phys. Rev. C 105, 044908 (2022).
https://journals.aps.org/prc/abstract/10.1103/PhysRevC.105.044908
Related to Project C06
Abstract:
A clear understanding of nuclear parton distribution functions (nPDFs) plays a crucial role in the interpretation of collider data taken at the Relativistic Heavy Ion Collider (RHIC), the Large Hadron Collider (LHC) and in the near future at the Electron-Ion Collider (EIC). Even with the recent inclusions of vector boson and light meson production data, the uncertainty of the gluon PDF remains substantial and limits the interpretation of heavy ion collision data. To obtain new constraints on the nuclear gluon PDF, we extend our recent nCTEQ15WZ+SIH analysis to inclusive quarkonium and open heavy-flavor meson production data from the LHC. This vast new data set covers a wide kinematic range and puts strong constraints on the nuclear gluon PDF down to x≲10−5. The theoretical predictions for these data sets are obtained from a data-driven approach, where proton-proton data are used to determine effective scattering matrix elements. This approach is validated with detailed comparisons to existing next-to-leading order (NLO) calculations in non-relativistic QCD (NRQCD) for quarkonia and in the general-mass variable-flavor-number scheme (GMVFNS) for the open heavy-flavored mesons. In addition, the uncertainties from the data-driven approach are determined using the Hessian method and accounted for in the PDF fits. This extension of our previous analyses represents an important step toward the next generation of PDFs not only by including new data sets, but also by exploring new methods for future analyses.
P. Duwentäster, T. Ježo, M. Klasen, K. Kovařík, A. Kusina, K.F. Muzakka, F.I. Olness, R. Ruiz, I. Schienbein, J.Y. Yu, “Impact of heavy quark and quarkonium data on nuclear gluon PDFs”, arXiv:2204.09982 (2022).
https://arxiv.org/abs/2204.09982
Related to Project C05
Abstract:
We use the resonant dipole-dipole interaction between Rydberg atoms and a periodic external microwave field to engineer XXZ spin Hamiltonians with tunable anisotropies. The atoms are placed in one-dimensional (1D) and two-dimensional (2D) arrays of optical tweezers. As illustrations, we apply this engineering to two iconic situations in spin physics: the Heisenberg model in square arrays and spin transport in 1D. We first benchmark the Hamiltonian engineering for two atoms and then demonstrate the freezing of the magnetization on an initially magnetized 2D array. Finally, we explore the dynamics of 1D domain-wall systems with both periodic and open boundary conditions. We systematically compare our data with numerical simulations and assess the residual limitations of the technique as well as routes for improvement. The geometrical versatility of the platform, combined with the flexibility of the simulated Hamiltonians, opens up exciting prospects in the fields of quantum simulation, quantum information processing, and quantum sensing.
P. Scholl, H. J. Williams, G. Bornet, F.Wallner, D. Barredo, L. Henriet, A. Signoles, C. Hainaut, T. Franz, S. Geier, A. Tebben, A. Salzinger, G. Zürn, T. Lahaye, M. Weidemüller, A. Browaeys, “Microwave Engineering of Programmable XXZ Hamiltonians in Arrays of Rydberg Atoms”, PRX Quantum 3, 020303 (2022).
https://journals.aps.org/prxquantum/abstract/10.1103/PRXQuantum.3.020303
Related to Project A05
Abstract:
We comprehensively investigate two distinct mechanisms leading to memory loss of non-Gaussian correlations after switching off the interactions in an isolated quantum system undergoing out-of-equilibrium dynamics. The first mechanism is based on spatial scrambling and results in the emergence of locally Gaussian steady states in large systems evolving over long times. The second mechanism, characterized as `canonical transmutation’, is based on the mixing of a pair of canonically conjugate fields, one of which initially exhibits non-Gaussian fluctuations while the other is Gaussian and dominates the dynamics, resulting in the emergence of relative Gaussianity even at finite system sizes and times. We evaluate signatures of the occurrence of the two candidate mechanisms in a recent experiment that has observed Gaussification in an atom-chip controlled ultracold gas and elucidate evidence that it is canonical transmutation rather than spatial scrambling that is responsible for Gaussification in the experiment. Both mechanisms are shown to share the common feature that the Gaussian correlations revealed dynamically by the quench are already present though practically inaccessible at the initial time. On the way, we present novel observations based on the experimental data, demonstrating clustering of equilibrium correlations, analyzing the dynamics of full counting statistics, and utilizing tomographic reconstructions of quantum field states. Our work aims at providing an accessible presentation of the potential of atom-chip experiments to explore fundamental aspects of quantum field theories in quantum simulations.
M. Gluza, T. Schweigler, M. Tajik, J. Sabino, F. Cataldini, F. S. Møller, S.-C. Ji, B. Rauer, J. Schmiedmayer, J. Eisert, S. Sotiriadis, “Mechanisms for the emergence of Gaussian correlations”, SciPost Phys. 12, 113 (2022).
https://scipost.org/10.21468/SciPostPhys.12.3.113
Related to Project A03
Abstract:
We discuss a remarkable correspondence between the description of black holes as highly occupied condensates of N weakly interacting gravitons and that of color glass condensates (CGCs) as highly occupied gluon states. In both cases, the dynamics of “wee partons” in Regge asymptotics is controlled by emergent semihard scales that lead to perturbative unitarization and classicalization of 2→N particle amplitudes at weak coupling. In particular, they attain a maximal entropy permitted by unitarity, bounded by the inverse coupling α of the respective constituents. Strikingly, this entropy is equal to the area measured in units of the Goldstone constant corresponding to the spontaneous breaking of Poincaré symmetry by the corresponding graviton or gluon condensate. In gravity, the Goldstone constant is the Planck scale, and gives rise to the Bekenstein-Hawking entropy. Likewise, in the CGC, the corresponding Goldstone scale is determined by the onset of gluon screening. We point to further similarities in black hole formation, thermalization and decay, to that of the glasma matter formed from colliding CGCs in ultrarelativistic nuclear collisions, which decays into a quark-gluon plasma.
G. Dvali, R. Venugopalan, “Classicalization and unitarization of wee partons in QCD and gravity: The CGC-black hole correspondence”, Phys. Rev. D 105, 056026 (2022).
https://journals.aps.org/prd/abstract/10.1103/PhysRevD.105.056026
Related to Project B03
Abstract:
We numerically study out-of-equilibrium dynamics in a family of Heisenberg models with 1/r6 power-law interactions and positional disorder. Using the semi-classical discrete truncated Wigner approximation (dTWA) method, we investigate the time evolution of the magnetization and ensemble-averaged single-spin purity for a strongly disordered system after initializing the system in an out-of-equilibrium state. We find that both quantities display robust glassy behavior for almost any value of the anisotropy parameter of the Heisenberg Hamiltonian. Furthermore, a systematic analysis allows us to quantitatively show that, for all the scenarios considered, the stretch power lies close to the one analytically obtained in the Ising limit. This indicates that glassy relaxation behavior occurs widely in disordered quantum spin systems, independent of the particular symmetries and integrability of the Hamiltonian.
P. Schultzen, T. Franz, C. Hainaut, S. Geier, A. Salzinger, A. Tebben, G. Zürn, M. Gärttner, M. Weidemüller, “Semiclassical simulations predict glassy dynamics for disordered heisenberg models”, Phys. Rev. B 105, L100201 (2022).
https://journals.aps.org/prb/abstract/10.1103/PhysRevB.105.L100201
Related to Project A05
Abstract:
Entropic uncertainty is a well-known concept to formulate uncertainty relations for continuous variable quantum systems with finitely many degrees of freedom. Typically, the bounds of such relations scale with the number of oscillator modes, preventing a straight-forward generalization to quantum field theories. In this work, we overcome this difficulty by introducing the notion of a functional relative entropy and show that it has a meaningful field theory limit. We present the first entropic uncertainty relation for a scalar quantum field theory and exemplify its behavior by considering few particle excitations and the thermal state. Also, we show that the relation implies the multidimensional Heisenberg uncertainty relation.
S. Floerchinger, T. Haas, M. Schröfl, “Relative entropic uncertainty relation for scalar quantum fields”, SciPost Phys. 12, 89 (2022).
https://scipost.org/10.21468/SciPostPhys.12.3.089
Related to Project A06
Abstract:
We reconstruct ghost and gluon spectral functions in 2+1 flavor QCD with Gaussian process regression. This framework allows us to largely suppress spurious oscillations and other common reconstruction artifacts by specifying generic magnitude and length scale parameters in the kernel function. The Euclidean propagator data are taken from lattice simulations with domain wall fermions at the physical point. For the infrared and ultraviolet extensions of the lattice propagators as well as the low-frequency asymptotics of the ghost spectral function, we utilize results from functional computations in Yang-Mills theory and QCD. This further reduces the systematic error significantly. Our numerical results are compared against a direct real-time functional computation of the ghost and an earlier reconstruction of the gluon in Yang-Mills theory. The systematic approach presented in this work offers a promising route toward unveiling real-time properties of QCD.
J. Horak, J. Pawlowski, J. M. Rodríguez-Quintero, J. Turnwald, J. M. Urban, N. Wink, S. Zafeiropoulos, “Reconstructing QCD spectral functions with Gaussian processes”, Phys. Rev. D 105, 036014 (2022).
https://journals.aps.org/prd/abstract/10.1103/PhysRevD.105.036014
Related to Project A02
Abstract:
The interpretation of the emergent collective behaviour of atomic nuclei in terms of deformed intrinsic shapes [1] is at the heart of our understanding of the rich phenomenology of their structure, ranging from nuclear energy to astrophysical applications across a vast spectrum of energy scales. A new window onto the deformation of nuclei has been recently opened with the realization that nuclear collision experiments performed at high-energy colliders, such as the CERN Large Hadron Collider (LHC), enable experimenters to identify the relative orientation of the colliding ions in a way that magnifies the manifestations of their intrinsic deformation [2]. Here we apply this technique to LHC data on collisions of 129Xe nuclei [3-5] to exhibit the first evidence of non-axiality in the ground state of ions collided at high energy. We predict that the low-energy structure of 129Xe is triaxial (a spheroid with three unequal axes), and show that such deformation can be determined from high-energy data. This result demonstrates the unique capabilities of precision collider machines such as the LHC as new means to perform imaging of the collective structure of atomic nuclei.
B. Bally, M. Bender, G. Giacalone, V. Somà, “Evidence of the triaxial structure of 129Xe at the large hadron collider”, Phys. Rev. Lett. 128, 082301 (2022).
https://journals.aps.org/prl/abstract/10.1103/PhysRevLett.128.082301
Related to Project C06
Abstract:
We investigate BRST invariance in Landau gauge Yang-Mills theory with functional methods. To that end, we solve the coupled systems of functional renormalisation group for the momentumdependent ghost and gluon propagator, ghost-gluon, and three- and four-gluon vertex dressings. The equations for both, transverse and longitudinal correlation functions are solved self-consistently: all correlation functions are fed back into the loops. Additionally, we also use the Slavnov-Taylor identities for computing the longitudinal correlation functions on the basis of the above results. Then, the gauge consistency of the solutions is checked by comparing the respective longitudinal correlation functions. We find good agreement of these results, hinting at the gauge consistency of our setup.
J. M. Pawlowski, C. S. Schneider, N. Wink, “On Gauge Consistency In Gauge-Fixed Yang-Mills Theory”, arXiv:2202.11123 (2022).
https://arxiv.org/abs/2202.11123
Related to Project A02, C06
Abstract:
We consider the coupled set of spectral Dyson-Schwinger equations in Yang-Mills theory for ghost and gluon propagators, which gives us access to the ghost and gluon spectral functions. The set-up is used for a systematic analytic evaluation of the constraints on generalised spectral representations in Yang-Mills theory that are most relevant for informed spectral reconstructions. We also provide numerical results for the coupled set of spectral functions for a large range of potential mass gaps of the gluon, and discuss the limitations and extensions of the present work.
J. Horak, J. M. Pawlowski, N. Wink, “On the complex structure of Yang-Mills theory”, arXiv:2202.09333 (2022).
https://arxiv.org/abs/2202.09333
Related to Project A02, C06
Abstract:
We report on the stochastic dynamics of a few sodium atoms immersed in a cold potassium cloud. The studies are realized in a dual-species magneto-optical trap by continuously monitoring the emitted fluorescence of the two atomic species. We investigate the time evolution of sodium and potassium atoms in a unified statistical language and study the detection limits. We resolve the sodium atom dynamics accurately, which provides a fit free analysis. This work paves the path towards precise statistical studies of the dynamical properties of few atoms immersed in complex quantum environments.
R. P. Bhatt, J. Kilinc, L. Höcker, F. Jendrzejewski, “Stochastic dynamics of a few sodium atoms in a cold potassium cloud”, Sci Rep 12, 2422 (2022).
https://www.nature.com/articles/s41598-022-05778-8
Related to Project B04
Abstract:
A highly effective approach to the search for hypothetical new interactions through isotope shift spectroscopy of hydrogen-like ions is presented. A weighted difference of the g factor and ground-state energy is shown to assist in the suppression of detrimental uncertainties from nuclear structure, while preserving the hypothetical contributions from new interactions. Experimental data from only a single isotope pair is required. Account is taken of the small, subleading nuclear corrections, allowing to show that, provided feasible experimental progress is achieved in UV/X-ray spectroscopy, the presented approach can yield competitive bounds on New Physics electron coupling parameters improved by more than an order of magnitude compared to leading bounds from atomic physics.
V. Debierre, C. H. Keitel, Z. Harman, “Tests of physics beyond the Standard Model with single-electron ions”, arXiv:2202.01668 (2022).
https://arxiv.org/abs/2202.01668
Related to Project B02
Abstract:
The notion of the “size” of nucleons and their constituents plays a pivotal role in the current paradigm of the formation and the fluctuations of the quark-gluon plasma produced in high-energy nuclear collision experiments. We report on state-of-the-art hydrodynamic results showing that the correlation between anisotropic flow, v2n, and the mean transverse momentum of hadrons, [pt], possesses a unique sensitivity to the nucleon size in off-central heavy-ion collisions. We argue that existing experimental measurements of this observable support a picture where the relevant length scale characterizing the colliding nucleons is of order 0.5 fm or smaller, and we discuss the broad implications of this finding for future global Bayesian analyses aimed at extracting initial-state and medium properties from nucleus-nucleus collision data, including v2n–[pt] correlations. Determinations of the nucleon size in heavy-ion collisions will provide a solid independent constraint on the initial state of small system collisions, and will establish a deep connection between collective flow data in nucleus-nucleus experiments and data on deep inelastic scattering on protons and nuclei.
G. Giacalone, B. Schenke, C. Shen, “Constraining the nucleon size with relativistic nuclear collisions”, Phys. Rev. Lett. 128, 042301 (2022).
https://journals.aps.org/prl/abstract/10.1103/PhysRevLett.128.042301
Related to Project C06
Abstract:
We perform a dynamical finite-size scaling analysis of a nonequilibrium Bose gas which is confined in the transverse plane. Varying the transverse size, we establish a dimensional crossover for universal scaling properties far from equilibrium. Our results, which are based on classical-statistical real-time lattice simulations, suggest that some aspects of the dynamical universal behavior of anisotropic systems can be classified in terms of fractional spatial dimensions. We discuss our findings in view of recent experimental results with quasi-one-dimensional setups of quenched ultracold quantum gases.
L. Gresista, T. V. Zache, J. Berges, “Dimensional crossover for universal scaling far from equilibrium”, Phys. Rev. A 105, 013320 (2022).
https://journals.aps.org/pra/abstract/10.1103/PhysRevA.105.013320
Related to Project B03, A03, A04, A05
Abstract:
The approximation of quantum states with artificial neural networks has gained a lot of attention during the last years. Meanwhile, analog neuromorphic chips, inspired by structural and dynamical properties of the biological brain, show a high energy efficiency in running artificial neural-network architectures for the profit of generative applications. This encourages employing such hardware systems as platforms for simulations of quantum systems. Here we report on the realization of a prototype using the latest spike-based BrainScaleS hardware allowing us to represent few-qubit maximally entangled quantum states with high fidelities. Bell correlations of pure and mixed two-qubit states are well captured by the analog hardware, demonstrating an important building block for simulating quantum systems with spiking neuromorphic chips.
S. Czischek, A. Baumbach, S. Billaudelle, B. Cramer, L. Kades,
J. M. Pawlowski, M. K. Oberthaler, J. Schemmel, M. A. Petrovici, T.
Gasenzer, and M. Gärttner, “Spiking neuromorphic chip learns entangled quantum states”, SciPost Phys. 12, 039 (2022).
https://scipost.org/10.21468/SciPostPhys.12.1.039
Related to Project A04
Abstract:
We study out-of-equilibrium dynamics in the quantum Ising model with power-law interactions and positional disorder. For arbitrary dimension d and interaction range α≥d we analytically find a stretched exponential decay with stretch power β=d/α for the global magnetization and ensemble-averaged single-spin purity in the thermodynamic limit. We reveal numerically that glassy behavior persists for finite system sizes and sufficiently strong disorder. We conclude that the magnetization decay is due to interaction induced dephasing while entanglement builds up at a smaller rate evident from the decay of single-spin purity, thus providing a microscopic understanding of glassy dynamics in disordered closed quantum systems.
P. Schultzen, T. Franz, S. Geier, A. Salzinger, A. Tebben, C. Hainaut, G. Zürn, M.Weidemüller, M. Gärttner, “Glassy quantum dynamics of disordered ising spins”, Phys. Rev. B 105, L020201 (2022).
https://journals.aps.org/prb/abstract/10.1103/PhysRevB.105.L020201
Related to Project A05
Following the successful SciArt residency together with Kalamari Klub, the next step in the project is the publication of a magazine! maybe. magazine Vol. 2 will collect works from the intersection between art and science. Publication date is in summer 2022. Together with the Kalamari Klub, IsoQuant invites artists from all around the world to apply! The full open call with all guidelines can be found here, below are the most important rules.
We would like to invite all artists working within the realms of analogue and experimental photography to submit their works concerning the interface of art and science in general. We welcome a broad range of works interpreting the links between these two approaches to understand our world.
To be considered for publication in the magazine, please submit a maximum of 10 images. Optionally, you can add a short artist statement that describes the submitted set or body of work. Please submit your work as preview files either via email to magazine@kalamariklub.org, or, alternatively, via SendAnywhere or WeTransfer. All files have to be zipped to one .zip folder; the total size of that folder may not exceed 10 MBs. In case your submission is chosen for publication, we will ask you to send high-quality files for printing. All published artists will receive one magazine free of charge and a discount on additional copies.
All analogue processes, formats, techniques and experience levels are welcome. Please follow guidelines closely.
Guidelines
Submitted works must feature an artistic take on science or be influenced by science or the scientific method. Preferably, submitted works are related to the method of analogue photography (scans from negative or print, works on photographic paper, darkroom-based experimental work, polaroids, cyanotypes, etc.). Single strong artistic positions without direct connection to analogue work will be considered for publication, too.
Optionally, works can be accompanied by an artist statement or a description of the used method. Those may be included in the magazine.
Distribution
Once finalized, the printed magazine will be distributed online and through direct sales at selected locations. All publishing artists and photographers will receive an artists’ copy of the magazine free of charge and additional issues at a discounted rate.
Artist’s rights
The copyright of all images, without exception, remain with the artist. We respect artists as creators and owners of their work.
With your submission, you consent to the publication of the submitted works in the magazine. Images published in the magazine may also be presented on our social media channels and our website. If you do not wish to have your work shown there, please indicate so. We will give photo credits on all publication channels. Your submitted data will not be handed to third parties and will only be used for magazine-related purposes.
Selection process
Images will be selected based on unique artistic vision, a novel perspective towards the topic, and how well they fit into the overall composition of the magazine. The selection will be done by members of Kalamari Klub and IsoQuant. The jury consists of analogue photographers, artists, and scientists who hold a variety of approaches (and opinions) towards photography. We are happy to be exposed to a wide diversity of styles, methods, and approaches.
We will notify you regarding the status of your submission within three weeks after the submission deadline.
If you have any questions, do not hesitate to contact us at magazine@kalamariklub.org.
Checklist:
- Up to 10 images per submission
- Registration & data form
- Submission as a .zip folder containing all files, sent either via mail or WeTransfer
- The .zip folder does not exceed 10MB
- Folder name: lastname_firstname.zip
- File name: lastname_firstname_title_year_[xx].jpg ([xx] represents the numbering of the files). Images without title will be named „untitled“
- Artist statement (optional)
Images must relate to the interface of art and science.
Abstract:
A prerequisite for the comprehensive understanding of many-body quantum systems is a characterization in terms of their entanglement structure. The experimental detection of entanglement in spatially extended many-body systems describable by quantum fields still presents a major challenge. We develop a general scheme for certifying entanglement and demonstrate it by revealing entanglement between distinct subsystems of a spinor Bose-Einstein condensate. Our scheme builds on the spatially resolved simultaneous detection of the quantum field in two conjugate observables which allows the experimental confirmation of quantum correlations between local as well as non-local partitions of the system. The detection of squeezing in Bogoliubov modes in a multi-mode setting illustrates its potential to boost the capabilities of quantum simulations to study entanglement in spatially extended many-body systems.
P. Kunkel, M. Prüfer, S. Lannig, R. Strohmaier, M. Gärttner, H. Strobel, M. K. Oberthaler, “Detecting entanglement structure in continuous many-body quantum systems”, Phys. Rev. Lett. 128, 020402 (2022).
https://journals.aps.org/prl/abstract/10.1103/PhysRevLett.128.020402
Related to Project A06, B04
Abstract:
The functional renormalization group is employed to study the nonlinear regime of late-time cosmic structure formation. This framework naturally allows for nonperturbative approximation schemes, usually guided by underlying symmetries or a truncation of the theory space. An extended symmetry that is related to Galilean invariance is studied and corresponding Ward identities are derived. These are used to obtain (formally) closed renormalization group flow equations for two-point correlation functions in the limit of large wave numbers (small scales). The flow equations are analytically solved in an approximation that is connected to the “sweeping effect” previously described in the context of fluid turbulence.
Galilean invariance, and approximate solutions to the flow equations”, Phys. Rev. D 105, 023506 (2022).
https://journals.aps.org/prd/abstract/10.1103/PhysRevD.105.023506
Related to Project C06
Abstract:
We derive inseparability criteria for the phase space representation of quantum states in terms of variants of Wehrl’s entropy. In contrast to entropic criteria involving differential entropies of marginal phase space distributions, our criteria are based on the Husimi Q-distribution. This is experimentally accessible through the heterodyne detection scheme, avoiding costly tomographic measurements. We apply our entropic criteria to Gaussian states and show that they imply a pair of second-order criteria for moments. We exemplify the strengths of our entropic approach by considering several classes of non-Gaussian states where second-order criteria fail. We show that our criteria certify entanglement in previously undetectable regions highlighting the strength of using the Husimi Q-distribution for entanglement detection.
S. Floerchinger, M. Gärttner, T. Haas, O. R. Stockdale, “Entropic entanglement criteria in phase space”, Phys. Rev. A 105, 012409 (2022).
https://journals.aps.org/pra/abstract/10.1103/PhysRevA.105.012409
Related to Project A06
Abstract:
We reconstruct the Lorentzian graviton propagator in asymptotically safe quantum gravity from Euclidean data. The reconstruction is applied to both the dynamical fluctuation graviton and the background graviton propagator. We prove that the spectral function of the latter necessarily has negative parts similar to, and for the same reasons, as the gluon spectral function. In turn, the spectral function of the dynamical graviton is positive. We argue that the latter enters cross sections and other observables in asymptotically safe quantum gravity. Hence, its positivity may hint at the unitarity of asymptotically safe quantum gravity.
A. Bonanno, T. Denz, J. M. Pawlowski, M. Reichert, “Reconstructing the graviton”, SciPostPhys. 12, 1 (2022).
https://scipost.org/10.21468/SciPostPhys.12.1.001
Related to Project A02, B03, C01
Abstract:
We derive the temporal renormalisation group flow of the energy-momentum tensor at the example of a general scalar theory. The local causal structure of the temporal renormalisation group flow allows to monitor and control causality, unitarity and general conservation laws at each infinitesimal renormalisation group step. We explore energy-conserving truncations in a comparison of generic flows and the causal temporal flow of the energy-momentum tensor. We also observe that the temporal regulator preserves scale invariance, which is violated for generic momentum regulators. Specifically we discuss the relation of these terms to the trace anomaly of the energy-momentum tensor. Moreover, we show that the causal temporal flow of the energy-momentum tensor can be integrated analytically, and demonstrate, that the result is consistent with energy conservation.
M. Heller, J. M. Pawlowski, “Causal Temporal Renormalisation Group Flow of the Energy-Momentum Tensor”, arXiv:2112.12652 (2022).
https://arxiv.org/abs/2112.12652
Related to Project B03
Abstract:
Using next-to-leading order (NLO) perturbative QCD, we calculate the diffractive contribution to inclusive dijet photoproduction in Pb-Pb ultraperipheral collisions (UPCs) at the LHC and find that it does not exceed 5% in small-xA bins in the ATLAS kinematics at sNN−−−−√=5.02 TeV. Its smallness is a result of the restricted kinematics (pT1>20 GeV and xA>0.001) and the large nuclear suppression of nuclear diffractive parton distribution functions predicted in the leading twist model of nuclear shadowing. Thus, in an analysis of new constraints on nuclear parton distribution functions (PDFs) at small xA using the LHC data on inclusive dijet photoproduction in heavy-ion UPCs, one can safely neglect the correction factor due to the excluded diffractive contribution. At the same time, applying our framework to proton-proton UPCs at sNN−−−−√=13 TeV, we find that the ratio of the diffractive and inclusive cross sections of dijet photoproduction can reach 20−25% for xp∼5×10−5.
V. Guzey and M. Klasen, “How large is the diffractive contribution to inclusive dijet photoproduction in UPCs at the LHC?”, Phys. Rev. D 104, 114013 (2021).
https://journals.aps.org/prd/abstract/10.1103/PhysRevD.104.114013
Related to Project C05
Abstract:
In the hydrodynamic framework of heavy-ion collisions, elliptic flow v2 is sensitive to the quadrupole deformation β of the colliding ions. This enables one to test whether the established knowledge on the low-energy structure of nuclei is consistent with collider data from high-energy experiments. We derive a formula based on generic scaling laws of hydrodynamics to relate the difference in v2 measured between collision systems that are close in size to the value of β of the respective species. We validate our formula in simulations of 238U+238U and 197Au+197Au collisions at top Relativistic Heavy Ion Collider (RHIC) energy, and subsequently apply it to experimental data. Using the deformation of 238U from low-energy experiments, we find that RHIC v2 data implies 0.16≲|β|≲0.20 for 197Au nuclei, i.e., significantly more deformed than reported in the literature, posing an interesting issue in nuclear phenomenology.
G. Giacalone, J. Jia, C. Zhang, “Impact of Nuclear Deformation on Relativistic Heavy-Ion Collisions: Assessing Consistency in Nuclear Physics across Energy Scales”, Phys. Rev. Lett. 127, 242301 (2021).
https://journals.aps.org/prl/abstract/10.1103/PhysRevLett.127.242301
Related to Project C06
Press Release of Heidelberg University
06. December 2021
In recognition of her especially meritorious service to German science, Prof. Dr Johanna Stachel, a physicist at Ruperto Carola, has been awarded the Cross of Merit (1st Class) of the Order of Merit of the Federal Republic of Germany. On the proposal of the Minister-President of Baden-Württemberg, the Federal President hereby honoured the nuclear and particle physicist for her life’s work. Theresia Bauer, Baden-Württemberg’s Minister of Science, Research and the Arts, presented the order on 3 December 2021 during the Physical Colloquium at Heidelberg University. Prof. Stachel had already been awarded the Cross of Merit with riband in 1999.
“In a situation in which fact-based, scientific findings are more important than ever for mastering the challenges before us, we especially need the social commitment of scientists,” said Theresia Bauer when presenting the order. With over 600 publications and many other scientific contributions, Johanna Stachel had increased the visibility of the large Faculty of Physics and Astronomy of Heidelberg University, the minister added. At the same time she paid tribute to Prof. Stachel as an “inspiring scientific teacher”. Furthermore, Johanna Stachel exemplifies what women achieve at the top level in the natural sciences and, with her work, builds “lasting bridges for international cooperation, also in areas beyond project-related scientific collaboration,” said Theresia Bauer.
A proven international expert in the field of nuclear and particle physics, Prof. Stachel works at the European nuclear research centre CERN in Geneva (Switzerland) and is primarily involved in experiments with the Large Hadron Collider (LHC) in the context of the ALICE collaboration. Her special interest is in quark-gluon plasma, a primeval state of matter that today can only be generated with the aid of particle accelerators such as the LHC. As director of the ALICE Transition Radiation Detector project, she studies the behaviour of the state of matter from free quarks and gluons. In particular, her phenomenological work has made a significant contribution to defining the phase boundary between normal matter and quark-gluon plasma with the aid of experimental data. Close collaborators with her in this field are Prof. Dr Peter Braun-Munzinger of the GSI Helmholtz Centre for Heavy Ion Research in Darmstadt and two colleagues at the Universities of Münster and Wrocław (Poland).
Johanna Stachel studied physics and chemistry at ETH Zürich (Switzerland) and at the University of Mainz, where she also earned her doctorate in 1982. Then she spent thirteen years as professor at the State University of New York at Stony Brook (US) before, in 1996, she was appointed to a professorship for experimental physics at Heidelberg University. Since 1996 Prof. Stachel has also carried out research at CERN, from 2000 as project leader and a member of the management board of the ALICE project.
The physicist is a member of various high-level scientific academies and societies, and is active in various ways in funding agencies for scientific research. Prof. Stachel has also served as a reviewer and consultant, e.g. for the Federal Ministry of Education and Research, and for different national and international scientific societies and research institutes. From 2012 to 2014 she was the first woman to head the German Physical Society (DPG), followed by a two-year term as vice-president.
Johanna Stachel’s scientific work has been awarded a number of prizes, notably the Lise Meitner Prize of the European Physical Society and, most recently, the Stern-Gerlach Medal of the German Physical Society – the highest honour given by the DPG in the field of experimental physics. In addition, in 2001 she received the Lautenschläger Research Prize, awarded for the first time, which recognises outstanding achievements in cutting-edge research.
Contact
Prof. Dr. Johanna Stachel
Physikalisches Institut
Im Neuenheimer Feld 226
69120 Heidelberg
phone.: +49 6221- 54 19 501
email: stachel@physi.uni-heidelberg.de
Press Release of Heidelberg University
06. December 2021
In recognition of her especially meritorious service to German science, Prof. Dr Johanna Stachel, a physicist at Ruperto Carola, has been awarded the Cross of Merit (1st Class) of the Order of Merit of the Federal Republic of Germany. On the proposal of the Minister-President of Baden-Württemberg, the Federal President hereby honoured the nuclear and particle physicist for her life’s work. Theresia Bauer, Baden-Württemberg’s Minister of Science, Research and the Arts, presented the order on 3 December 2021 during the Physical Colloquium at Heidelberg University. Prof. Stachel had already been awarded the Cross of Merit with riband in 1999.
“In a situation in which fact-based, scientific findings are more important than ever for mastering the challenges before us, we especially need the social commitment of scientists,” said Theresia Bauer when presenting the order. With over 600 publications and many other scientific contributions, Johanna Stachel had increased the visibility of the large Faculty of Physics and Astronomy of Heidelberg University, the minister added. At the same time she paid tribute to Prof. Stachel as an “inspiring scientific teacher”. Furthermore, Johanna Stachel exemplifies what women achieve at the top level in the natural sciences and, with her work, builds “lasting bridges for international cooperation, also in areas beyond project-related scientific collaboration,” said Theresia Bauer.
A proven international expert in the field of nuclear and particle physics, Prof. Stachel works at the European nuclear research centre CERN in Geneva (Switzerland) and is primarily involved in experiments with the Large Hadron Collider (LHC) in the context of the ALICE collaboration. Her special interest is in quark-gluon plasma, a primeval state of matter that today can only be generated with the aid of particle accelerators such as the LHC. As director of the ALICE Transition Radiation Detector project, she studies the behaviour of the state of matter from free quarks and gluons. In particular, her phenomenological work has made a significant contribution to defining the phase boundary between normal matter and quark-gluon plasma with the aid of experimental data. Close collaborators with her in this field are Prof. Dr Peter Braun-Munzinger of the GSI Helmholtz Centre for Heavy Ion Research in Darmstadt and two colleagues at the Universities of Münster and Wrocław (Poland).
Johanna Stachel studied physics and chemistry at ETH Zürich (Switzerland) and at the University of Mainz, where she also earned her doctorate in 1982. Then she spent thirteen years as professor at the State University of New York at Stony Brook (US) before, in 1996, she was appointed to a professorship for experimental physics at Heidelberg University. Since 1996 Prof. Stachel has also carried out research at CERN, from 2000 as project leader and a member of the management board of the ALICE project.
The physicist is a member of various high-level scientific academies and societies, and is active in various ways in funding agencies for scientific research. Prof. Stachel has also served as a reviewer and consultant, e.g. for the Federal Ministry of Education and Research, and for different national and international scientific societies and research institutes. From 2012 to 2014 she was the first woman to head the German Physical Society (DPG), followed by a two-year term as vice-president.
Johanna Stachel’s scientific work has been awarded a number of prizes, notably the Lise Meitner Prize of the European Physical Society and, most recently, the Stern-Gerlach Medal of the German Physical Society – the highest honour given by the DPG in the field of experimental physics. In addition, in 2001 she received the Lautenschläger Research Prize, awarded for the first time, which recognises outstanding achievements in cutting-edge research.
Contact
Prof. Dr. Johanna Stachel
Physikalisches Institut
Im Neuenheimer Feld 226
69120 Heidelberg
phone.: +49 6221- 54 19 501
email: stachel@physi.uni-heidelberg.de
Pressemitteilung Universität Heidelberg
06. Dezember 2021
In Anerkennung ihrer besonderen Verdienste um die deutsche Wissenschaft ist Prof. Dr. Johanna Stachel, Physikern an der Ruperto Carola, mit dem Verdienstkreuz 1. Klasse des Verdienstordens der Bundesrepublik Deutschland ausgezeichnet worden. Auf Vorschlag des baden-württembergischen Ministerpräsidenten würdigt der Bundespräsident damit das Lebenswerk der Kern- und Teilchenphysikerin. Theresia Bauer, Ministerin für Wissenschaft, Forschung und Kunst des Landes Baden-Württemberg, überreichte den Orden am 3. Dezember 2021 im Rahmen des Physikalischen Kolloquiums an der Universität Heidelberg. Mit dem Verdienstkreuz am Bande war Prof. Stachel bereits 1999 geehrt worden.
„In einer Situation, in der faktenbasierte, wissenschaftliche Erkenntnisse wichtiger denn je sind für die Bewältigung der anstehenden Aufgaben, bedürfen wir ganz besonders des gesellschaftlichen Engagements von Wissenschaftlerinnen und Wissenschaftlern“, sagte Theresia Bauer anlässlich der Überreichung des Ordens. Mit mehr als 600 Publikationen und vielen weiteren fachlichen Beiträgen habe Johanna Stachel die Sichtbarkeit der großen Fakultät für Physik und Astronomie der Universität Heidelberg gemehrt, so die Ministerin, die Prof. Stachel zugleich als „inspirierende wissenschaftliche Lehrerin“ würdigte. Johanna Stachel lebe auch vor, was Frauen in den Naturwissenschaften auf Spitzenniveau leisten, und baue mit ihrer Arbeit „dauerhafte Brücken für internationale Kooperationen, auch jenseits projektbezogener wissenschaftlicher Zusammenarbeit“, sagte Theresia Bauer.
Als eine international ausgewiesene Expertin auf dem Gebiet der Kern- und Teilchenphysik ist Prof. Stachel am europäischen Forschungszentrum CERN in Genf (Schweiz) maßgeblich an den Experimenten mit dem Large Hadron Collider (LHC) im Rahmen der ALICE-Kollaboration beteiligt. Ihr spezielles Interesse gilt dem sogenannten Quark-Gluon Plasma, einem Urzustand von Materie, der heute nur mithilfe von Teilchenbeschleunigern wie dem LHC erzeugt werden kann. Als Leiterin des Projektes „ALICE Transition Radiation Detector“ untersucht sie, wie sich der Materiezustand aus freien Quarks und Gluonen verhält. Insbesondere haben ihre phänomenologischen Arbeiten entscheidend dazu beigetragen, die Phasengrenze zwischen normaler Materie und dem Quark-Gluon Plasma anhand experimenteller Daten zu bestimmen. Hier arbeitet sie eng mit Prof. Dr. Peter Braun-Munzinger vom GSI Helmholtzzentrum für Schwerionenforschung in Darmstadt sowie zwei Kollegen an den Universitäten Münster und Breslau (Polen) zusammen.
Johanna Stachel studierte Physik und Chemie an der Eidgenössischen Technischen Hochschule Zürich (Schweiz) und an der Universität Mainz, an der sie 1982 auch promoviert wurde. Im Anschluss daran war sie dreizehn Jahre als Professorin in den USA an der State University of New York at Stony Brook tätig, ehe sie 1996 auf eine Professur für Experimentalphysik an die Universität Heidelberg berufen wurde. Seit 1996 forscht Prof. Stachel am CERN, seit 2000 als Projektleiterin und Mitglied im Management Board des ALICE-Projekts.
Die Physikerin ist Mitglied in verschiedenen hochrangigen wissenschaftlichen Akademien und Gesellschaften und engagiert sich auch in vielfältiger Weise in Einrichtungen der Wissenschaftsförderung. Als Gutachterin und Beraterin war Prof. Stachel unter anderem für das Bundesministerium für Bildung und Forschung sowie für verschiedene nationale und internationale wissenschaftliche Gesellschaften und Forschungseinrichtungen tätig. Von 2012 bis 2014 stand sie – als erste Frau im Präsidentenamt – an der Spitze der Deutschen Physikalischen Gesellschaft (DPG), gefolgt von einer zweijährigen Amtszeit als Vizepräsidentin.
Johanna Stachels wissenschaftliche Arbeiten wurden unter anderem mit dem Lise-Meitner-Preis der Europäischen Physikalischen Gesellschaft und zuletzt mit der Stern-Gerlach-Medaille der Deutschen Physikalischen Gesellschaft gewürdigt – der höchsten Auszeichnung, die die DPG auf dem Gebiet der experimentellen Physik vergibt. Zudem erhielt sie 2001 den zum ersten Mal vergebenen Lautenschläger-Forschungspreis, mit dem besondere Leistungen in der Spitzenforschung ausgezeichnet werden.
Kontakt
Prof. Dr. Johanna Stachel
Physikalisches Institut
Im Neuenheimer Feld 226
69120 Heidelberg
Tel.: +49 6221- 54 19 501
Email: stachel@physi.uni-heidelberg.de
Abstract:
We develop a variational approach to simulating the dynamics of open quantum many-body systems using deep autoregressive neural networks. The parameters of a compressed representation of a mixed quantum state are adapted dynamically according to the Lindblad master equation by employing a time-dependent variational principle. We illustrate our approach by solving the dissipative quantum Heisenberg model in one and two dimensions for up to 40 spins and by applying it to the simulation of confinement dynamics in the presence of dissipation.
M. Reh, M. Schmitt, M. Gärttner, “Time-dependent variational principle for open quantum systems with artificial neural networks”, Phys. Rev. Lett. 127, 230501 (2021).
https://journals.aps.org/prl/abstract/10.1103/PhysRevLett.127.230501
Related to Project A05, A06
Abstract:
We study excitation transport in a two-dimensional system of randomly assembled spins with power-law hopping in two dimensions. This model can be realized in cold atom quantum simulators with Rydberg atoms. In these experiments, due to the Rydberg blockade effect, the degree of disorder in the system is effectively tunable by varying the spin density. We study dynamics and eigenstate properties of the model as a function of disorder strength and system size and discuss potential limitations for experiments. At strong disorder we predominantly observe localized eigenstates with power-law tails. In this regime the spectral and eigenstate properties can be understood in a perturbative picture of states localized on small clusters of spins. As the disorder strength is weakened eigenstates become increasingly delocalized and a set of seemingly multifractal states appears in the low-energy tail of the spectrum. A detailed study of the system-size scaling of the eigenstate properties indicates that in the infinite-size limit all states eventually become localized. We discuss the feasibility of observing localization effects experimentally in the spatial spreading of an initially localized excitation and identify limited system sizes and finite decoherence rates as major challenges. Our study paves the way towards an experimental observation of localization effects in Rydberg spin systems with tunable disorder.
J. P. Klinger, M. Gärttner, “Single-particle localization in a two-dimensional Rydberg spin system”, Phys. Rev. A 104, 063301 (2021).
https://journals.aps.org/pra/abstract/10.1103/PhysRevA.104.063301
Related to Project A05
Abstract:
Fluctuations of conserved charges are sensitive to the QCD phase transition and a possible critical endpoint in the phase diagram at finite density. In this work, we compute the baryon number fluctuations up to tenth order at finite temperature and density. This is done in a QCD-assisted effective theory that accurately captures the quantum- and in-medium effects of QCD at low energies. A direct computation at finite density allows us to assess the applicability of expansions around vanishing density. By using different freeze-out scenarios in heavy-ion collisions, we translate these results into baryon number fluctuations as a function of collision energy. We show that a non-monotonic energy dependence of baryon number fluctuations can arise in the non-critical crossover region of the phase diagram. Our results compare well with recent experimental measurements of the kurtosis and the sixth-order cumulant of the net-proton distribution from the STAR collaboration. They indicate that the experimentally observed non-monotonic energy dependence of fourth-order net-proton fluctuations is highly non-trivial. It could be an experimental signature of an increasingly sharp chiral crossover and may indicate a QCD critical point. The physics implications and necessary upgrades of our analysis are discussed in detail.
W.-J. Fu, X. Luo, J. M. Pawlowski, F. Rennecke, R. Wen, S. Yin, “Hyper-order baryon number fluctuations at finite temperature and density”, Phys. Rev. D 104, 094047 (2021).
https://journals.aps.org/prd/abstract/10.1103/PhysRevD.104.094047
Related to Project A02
Pressemitteilung Nr. 121/2021
26. November 2021
Die Kräfte zwischen Teilchen, Atomen, Molekülen oder sogar makroskopischen Objekten wie Magneten sind durch die Wechselwirkungen der Natur festgelegt. So richten sich zum Beispiel zwei nahe beieinanderliegende Stabmagnete unter der Wirkung magnetischer Kräfte neu aus. Einem Team um Prof. Dr. Matthias Weidemüller und Dr. Gerhard Zürn am Zentrum für Quantendynamik der Universität Heidelberg ist es nun gelungen, nicht nur die Stärke, sondern auch die Art der Wechselwirkung zwischen mikroskopischen Quanten-Magneten – den sogenannten Spins – gezielt zu verändern. Anstatt in einen Zustand vollständiger Unordnung zu verfallen, können die speziell präparierten Magnete ihre ursprüngliche Ausrichtung über einen langen Zeitraum aufrechterhalten. Damit haben die Heidelberger Physiker erfolgreich eine programmierbare Kontrolle der Wechselwirkung in isolierten Quantensystemen demonstriert.
Magnetische Systeme können überraschendes Verhalten zeigen, wenn sie in einer instabilen Konfiguration präpariert werden. Wird beispielsweise eine Ansammlung räumlich ungeordneter magnetischer Dipole, wie etwa Stabmagnete, in dieselbe Richtung eingestellt, führt dies zu einer anschließenden Neuausrichtung der Magnete. Diese Dynamik mündet schließlich in eine Gleichgewichtskonfiguration, bei der alle Magnete zufällig ausgerichtet sind. Während sich in der Vergangenheit die meisten Untersuchungen auf klassische magnetische Dipole beschränkten, ist es seit kurzem möglich, die Ansätze mit Hilfe sogenannter Quantensimulatoren auf Quanten-Magnete auszuweiten: Synthetische atomare Systeme ahmen die grundlegende Physik magnetischer Phänomene in einer extrem gut kontrollierten Umgebung nach, in der alle relevanten Parameter nahezu nach Belieben eingestellt werden können.
Für ihre Quantensimulationsexperimente verwendeten die Forscher ein Gas aus Atomen, das auf eine Temperatur nahe dem absoluten Nullpunkt abgekühlt wurde. Mithilfe von Laserlicht wurden die Atome in extrem hohe elektronische Zustände angeregt, die das Elektron um nahezu makroskopische Abstände vom Atomkern trennen. Diese „atomaren Riesen“, auch Rydberg-Atome genannt, stehen in Wechselwirkung über Distanzen von fast Haaresbreite miteinander. „Ein Ensemble von Rydberg-Atomen weist genau die gleichen Eigenschaften auf wie wechselwirkende ungeordnete Quanten-Magnete, was es zu einer idealen Plattform für die Simulation und Erforschung des Quantenmagnetismus macht“, sagt Dr. Nithiwadee Thaicharoen, die als Postdoktorandin in Prof. Weidemüllers Team am Physikalischen Institut tätig war und mittlerweile als Professorin in Thailand forscht.
Der wesentliche „Trick“ der Heidelberger Physiker bestand darin, die Dynamik der Quanten-Magnete zu steuern, indem sie Methoden aus dem Feld der Kernspinresonanz übernahmen. In ihren Experimenten nutzen die Wissenschaftler speziell entworfene periodische Mikrowellenpulse, um den atomaren Spin zu verändern. Eine große Herausforderung bestand darin, die Wechselwirkung der Spins mithilfe dieser Technik des sogenannten Floquet-Engineering präzise zu kontrollieren. „Die Mikrowellenpulse mussten auf Zeitskalen von einer Milliardstel Sekunde auf die Rydberg-Atome angewendet werden, wobei diese Atome zugleich sehr empfindlich auf jede noch so kleine äußere Störung wie winzige elektrische Felder reagieren“, so Postdoktorand Dr. Clément Hainaut, der kürzlich an die Universität Lille (Frankreich) gewechselt ist. „Dennoch gelang es auf diesem Weg, die scheinbar unausweichliche Neuausrichtung des Spins zum Stillstand zu bringen und eine makroskopische Magnetisierung unter der Wirkung unseres Kontrollprotokolls aufrecht zu erhalten“, erläutert Doktorand Sebastian Geier. „Mit unserem Ansatz des Floquet-Engineering sollte es nun möglich sein, die zeitliche Entwicklung des Spinsystems umzukehren, nachdem es eine sehr komplexe Dynamik durchlaufen hat. Das wäre so, als würde sich ein zerbrochenes Glas auf magische Weise wieder zusammensetzen, nachdem es auf den Boden gefallen ist.“
Die Arbeiten sind ein wichtiger Schritt, um grundlegende Prozesse in komplexen Quantensystemen besser zu verstehen. „Nach der ersten und zweiten Quantenrevolution, die zum Verständnis der Systeme und zur präzisen Kontrolle einzelner Objekte geführt haben, sind wir zuversichtlich, dass unsere Technik der dynamischen, programmierbaren Einstellung von Wechselwirkungen einen Beitrag auf dem Weg zu Quantentechnologien 3.0 leistet“, betont Matthias Weidemüller, Professor am Physikalischen Institut und Leiter des Zentrums für Quantendynamik der Universität Heidelberg.
Die Untersuchungen wurden im Rahmen des Exzellenzclusters STRUCTURES und des Sonderforschungsbereichs „Isolierte Quantensysteme und Universalität unter extremen Bedingungen“ (ISOQUANT) der Universität Heidelberg durchgeführt. Eingebunden sind die Forschungen zudem in PASQuans, die „Programmable Atomic Large-Scale Quantum Simulation“-Kollaboration innerhalb des europäischen Quantum Technologies Flagship.
Die aktuellen Forschungsergebnisse wurden in der Zeitschrift „Science“ veröffentlicht.
Originalpublikation:
S. Geier, N. Thaicharoen, C. Hainaut, T. Franz, A. Salzinger, A. Tebben, D. Grimshandl, G. Zürn, M. Weidemüller: Floquet Hamiltonian Engineering of an Isolated Many-Body Spin System, Science, Vol 374, Issue 6571, pp. 1149-1152 (25 November 2021)
https://www.science.org/doi/10.1126/science.abd9547
Kontakt
Prof. Dr. M. Weidemüller
Physikalisches Institut
Im Neuenheimer Feld 226
69120 Heidelberg
Tel.: +49 6221-5419470
E-mail: sekretariat-weidemueller@physi.uni-heidelberg.de
Press Release Heidelberg University No. 121/2021
26 November 2021
The forces between particles, atoms, molecules, or even macroscopic objects like magnets are determined by the interactions of nature. For example, two closely lying bar magnets realign themselves under the influence of magnetic forces. A team led by Prof. Dr Matthias Weidemüller and Dr Gerhard Zürn at the Center for Quantum Dynamics of Heidelberg University has now succeeded in its aim to change not only the strength but also the nature of the interaction between microscopic quantum magnets, known as spins. Instead of falling into a state of complete disorder, the especially prepared magnets can maintain their original orientation for a long period. With these findings, the Heidelberg physicists have successfully demonstrated a programmable control of spin interactions in isolated quantum systems.
Magnetic systems can exhibit surprising behaviour when they are prepared in an unstable configuration. For example, constraining a collection of spatially disordered magnetic dipoles, such as bar magnets, to be aligned in the same direction, will lead to a subsequent reorientation of the magnets. This ultimately results in an equilibrium in which all magnets are randomly oriented. While the majority of investigations used to be limited to classical magnetic dipoles, it has recently become possible to expand the approaches to quantum magnets using what are called quantum simulators. Synthetic atomic systems mimic the fundamental physics of magnetic phenomena in an extremely well-controlled environment where all relevant parameters can be adjusted almost at will.
In their quantum simulation experiments, the researchers used a gas of atoms that was cooled down to a temperature near absolute zero. Using laser light, the atoms were excited to extremely high electronic states, separating the electron by almost macroscopic distances from the atomic nucleus. These “atomic giants”, also known as Rydberg atoms, interact with each other over distances of almost a hair’s breadth. “An ensemble of Rydberg atoms exhibits exactly the same characteristics as interacting disordered quantum magnets, making it an ideal platform to simulate and explore quantum magnetism,” states Dr Nithiwadee Thaicharoen, who was a postdoc on Prof. Weidemüller’s team at the Institute for Physics and now continues her research as a professor in Thailand.
The essential trick of the Heidelberg physicists was to steer the dynamics of the quantum magnets by adopting methods from the field of nuclear magnetic resonance. In their experiments, the researchers apply especially designed periodic microwave pulses to modify the atomic spin. A major challenge was to precisely control the interaction between the atomic spins using this technique, known as Floquet engineering. “The microwave pulses had to be applied to the Rydberg atoms at timescales of a billionth of a second, with these atoms being super-sensitive at the same time to any external perturbation, however tiny, like minute electric fields,” says Dr Clément Hainaut, a postdoc on the team who recently moved to the University of Lille (France).
“We nonetheless succeeded in stalling the spin’s seemingly inevitable reorientation and maintaining a macroscopic magnetisation through our control protocol,” explains doctoral student Sebastian Geier. “Using our Floquet engineering approach, it should now be possible to reverse the timeline such that the spin system inverts its evolution after having gone through a very complex dynamic. It would be like a broken glass magically reassembling itself after it has crashed onto the floor.”
The studies are an important step towards a better understanding of basic processes in complex quantum systems. “After the first and second quantum revolution, which led to the understanding of the systems and the precise control of single objects, we are confident that our technique of dynamically adjusting interactions in a programmable fashion opens a path to Quantum Technologies 3.0,” concludes Matthias Weidemüller, professor at the Institute for Physics and Director of Heidelberg University’s Center for Quantum Dynamics.
The experiments were conducted in the framework of the STRUCTURES Cluster of Excellence and the “Isolated quantum systems and universality under extreme conditions” Collaborative Research Centre (ISOQUANT) of Heidelberg University. The activities are also part of PASQuans, the “Programmable Atomic Large-Scale Quantum Simulation” collaboration, within the European Quantum Technologies Flagship.
The research results were published in the journal “Science”.
Original publication:
S. Geier, N. Thaicharoen, C. Hainaut, T. Franz, A. Salzinger, A. Tebben, D. Grimshandl, G. Zürn, M. Weidemüller: Floquet Hamiltonian Engineering of an Isolated Many-Body Spin System, Science, Vol 374, Issue 6571, pp. 1149-1152 (25 November 2021)
https://www.science.org/doi/10.1126/science.abd9547
Contact
Prof. Dr. M. Weidemüller
Physikalisches Institut
Im Neuenheimer Feld 226
69120 Heidelberg
Tel.: +49 6221-5419470
Email: sekretariat-weidemueller@physi.uni-heidelberg.de
Press Release Heidelberg University No. 121/2021
26 November 2021
The forces between particles, atoms, molecules, or even macroscopic objects like magnets are determined by the interactions of nature. For example, two closely lying bar magnets realign themselves under the influence of magnetic forces. A team led by Prof. Dr Matthias Weidemüller and Dr Gerhard Zürn at the Center for Quantum Dynamics of Heidelberg University has now succeeded in its aim to change not only the strength but also the nature of the interaction between microscopic quantum magnets, known as spins. Instead of falling into a state of complete disorder, the especially prepared magnets can maintain their original orientation for a long period. With these findings, the Heidelberg physicists have successfully demonstrated a programmable control of spin interactions in isolated quantum systems.
Magnetic systems can exhibit surprising behaviour when they are prepared in an unstable configuration. For example, constraining a collection of spatially disordered magnetic dipoles, such as bar magnets, to be aligned in the same direction, will lead to a subsequent reorientation of the magnets. This ultimately results in an equilibrium in which all magnets are randomly oriented. While the majority of investigations used to be limited to classical magnetic dipoles, it has recently become possible to expand the approaches to quantum magnets using what are called quantum simulators. Synthetic atomic systems mimic the fundamental physics of magnetic phenomena in an extremely well-controlled environment where all relevant parameters can be adjusted almost at will.
In their quantum simulation experiments, the researchers used a gas of atoms that was cooled down to a temperature near absolute zero. Using laser light, the atoms were excited to extremely high electronic states, separating the electron by almost macroscopic distances from the atomic nucleus. These “atomic giants”, also known as Rydberg atoms, interact with each other over distances of almost a hair’s breadth. “An ensemble of Rydberg atoms exhibits exactly the same characteristics as interacting disordered quantum magnets, making it an ideal platform to simulate and explore quantum magnetism,” states Dr Nithiwadee Thaicharoen, who was a postdoc on Prof. Weidemüller’s team at the Institute for Physics and now continues her research as a professor in Thailand.
The essential trick of the Heidelberg physicists was to steer the dynamics of the quantum magnets by adopting methods from the field of nuclear magnetic resonance. In their experiments, the researchers apply especially designed periodic microwave pulses to modify the atomic spin. A major challenge was to precisely control the interaction between the atomic spins using this technique, known as Floquet engineering. “The microwave pulses had to be applied to the Rydberg atoms at timescales of a billionth of a second, with these atoms being super-sensitive at the same time to any external perturbation, however tiny, like minute electric fields,” says Dr Clément Hainaut, a postdoc on the team who recently moved to the University of Lille (France).
“We nonetheless succeeded in stalling the spin’s seemingly inevitable reorientation and maintaining a macroscopic magnetisation through our control protocol,” explains doctoral student Sebastian Geier. “Using our Floquet engineering approach, it should now be possible to reverse the timeline such that the spin system inverts its evolution after having gone through a very complex dynamic. It would be like a broken glass magically reassembling itself after it has crashed onto the floor.”
The studies are an important step towards a better understanding of basic processes in complex quantum systems. “After the first and second quantum revolution, which led to the understanding of the systems and the precise control of single objects, we are confident that our technique of dynamically adjusting interactions in a programmable fashion opens a path to Quantum Technologies 3.0,” concludes Matthias Weidemüller, professor at the Institute for Physics and Director of Heidelberg University’s Center for Quantum Dynamics.
The experiments were conducted in the framework of the STRUCTURES Cluster of Excellence and the “Isolated quantum systems and universality under extreme conditions” Collaborative Research Centre (ISOQUANT) of Heidelberg University. The activities are also part of PASQuans, the “Programmable Atomic Large-Scale Quantum Simulation” collaboration, within the European Quantum Technologies Flagship.
The research results were published in the journal “Science”.
Original publication:
S. Geier, N. Thaicharoen, C. Hainaut, T. Franz, A. Salzinger, A. Tebben, D. Grimshandl, G. Zürn, M. Weidemüller: Floquet Hamiltonian Engineering of an Isolated Many-Body Spin System, Science, Vol 374, Issue 6571, pp. 1149-1152 (25 November 2021)
https://www.science.org/doi/10.1126/science.abd9547
Contact
Prof. Dr. M. Weidemüller
Physikalisches Institut
Im Neuenheimer Feld 226
69120 Heidelberg
Tel.: +49 6221-5419470
Email: sekretariat-weidemueller@physi.uni-heidelberg.de
Abstract:
Controlling interactions is the key element for quantum engineering of many-body systems. Using time-periodic driving, a naturally given many-body Hamiltonian of a closed quantum system can be transformed into an effective target Hamiltonian exhibiting vastly different dynamics. We demonstrate such Floquet engineering with a system of spins represented by Rydberg states in an ultracold atomic gas. Applying a sequence of spin manipulations, we change the symmetry properties of the effective Heisenberg XYZ Hamiltonian. As a consequence, the relaxation behavior of the total spin is drastically modified. The observed dynamics can be qualitatively captured by a semi-classical simulation. Synthesising a wide range of Hamiltonians opens vast opportunities for implementing quantum simulation of non-equilibrium dynamics in a single experimental setting.
S. Geier, N. Thaicharoen, C. Hainaut, T. Franz, A. Salzinger, A. Tebben, D. Grimshandl, G. Zürn, M. Weidemüller, “Floquet Hamiltonian Engineering of an Isolated Many-Body Spin System”, Science 374, 1149 (2021).
https://www.science.org/doi/10.1126/science.abd9547
Related to Project A05
Pressemitteilung Universität Heidelberg
16. November 2021
Wie passen analoge Fotografie und innovative Forschung im Bereich der Quantenphysik zusammen? Diese Frage steht im Mittelpunkt einer Veranstaltung mit dem Fotografen Philip Kanwischer, zu der der Sonderforschungsbereich (SFB) „IsoQuant“ der Universität Heidelberg gemeinsam mit dem Kalamari Klub einlädt. Derzeit ist der Leipziger Künstler zu Gast an dem in der Physik angesiedelten SFB, um den Dialog zwischen Kunst und Wissenschaft zu fördern und für die interessierte Öffentlichkeit sichtbar zu machen. Der Artist Talk „New Links Between Different Systems“ findet am 18. November 2021 in englischer Sprache im Heidelberger Café Leitstelle statt und beginnt um 19 Uhr, parallel kann auch ein Livestream abgerufen werden. Für die Teilnahme ist eine Anmeldung erforderlich.
Philip Kanwischer konnte sich in einem Auswahlverfahren für die Besetzung der erstmals ausgeschriebenen SciArt Residency des in der Physik angesiedelten SFB 1225 „Isolierte Quantensysteme und Universalität unter extremen Bedingungen“ (IsoQuant) durchsetzen – eine Initiative zur Förderung der Kooperation zwischen Wissenschaft und Kunst. In diesem Rahmen arbeitet er seit Oktober mit den Wissenschaftlerinnen und Wissenschaftlern des SFB zusammen. Neben dem Artist Talk sind weitere Aktionen sowie eine Ausstellung der künstlerischen Arbeiten geplant. Kooperationspartner bei diesem Projekt ist der in Heidelberg ansässige „Kalamari Klub – Freiraum für analoge Fotografie“.
Der 1991 in Bamberg geborene Philip Kanwischer hat Fotografie an der Hochschule für Grafik und Buchkunst in Leipzig und an der Accademia di Belle Arti in Neapel (Italien) studiert. In seinen Arbeiten verknüpft er Bild und Textmaterial zu narrativen Raumsituationen und thematisiert dabei unter anderem die Wahrnehmung von Katastrophen sowie das Spannungsverhältnis zwischen Mensch und Natur. Seine Werke wurden in zahlreichen Einzel- und Gruppenausstellungen gezeigt. Er lebt und arbeitet in Leipzig.
Im Rahmen des Artist Talk wird Philip Kanwischer im Café Leitstelle, Emil-Maier-Straße 16, über seine künstlerische Arbeit Auskunft geben, im Anschluss daran folgt eine Diskussion mit Wissenschaftlern und Publikum. Für den Besuch der Veranstaltung vor Ort gilt die 2G-Regel (geimpft oder genesen mit Maskenpflicht). Eine Registrierung ist unter info@kalamariklub.org notwendig, das gilt auch für die Teilnahme am Livestream.
Kontakt
Dennis Schulz
SFB1225 ISOQUANT
Wissenschaftskommunikation
Philosophenweg 12
69120 Heidelberg
Tel.: +49 6221- 54 5058
Email: d.schulz@thphys.uni-heidelberg.de
Abstract:
Quantum information platforms made great progress in the control of many-body entanglement and the implementation of quantum error correction, but it remains a challenge to realize both in the same setup. Here, we propose a mixture of two ultracold atomic species as a platform for universal quantum computation with long-range entangling gates, while providing a natural candidate for quantum error-correction. In this proposed setup, one atomic species realizes localized collective spins of tunable length, which form the fundamental unit of information. The second atomic species yields phononic excitations, which are used to entangle collective spins. Finally, we discuss a finite-dimensional version of the Gottesman–Kitaev–Preskill code to protect quantum information encoded in the collective spins, opening up the possibility to universal fault-tolerant quantum computation in ultracold atom systems.
V. Kasper, D. González-Cuadra, A. Hegde, A. Xia, A. Dauphin, F. Huber, E. Tiemann, M. Lewenstein, F. Jendrzejewski, P. Hauke, “Universal quantum computation and quantum error correction with ultracold atomic mixtures”, Quantum Sci. Technol. 7, 015008 (2021).
https://iopscience.iop.org/article/10.1088/2058-9565/ac2d39
Related to Project B04
Abstract:
A precise knowledge of nuclear parton distribution functions (nPDFs) is—among other things—important for the unambiguous interpretation of hard process data taken in pA and AA collisions at the Relativistic Heavy Ion Collider (RHIC) and the LHC. The available fixed target data for deep inelastic scattering (DIS) and Drell-Yan (DY) lepton pair production mainly constrain the light quark distributions. It is hence crucial to include more and more collider data in global analyses of nPDFs in order to better pin down the different parton flavors, in particular the gluon distribution at small x. To help constrain the nuclear gluon PDF, we extend the nCTEQ15 analysis by including single inclusive hadron (SIH) production data from RHIC (PHENIX and STAR) and LHC (ALICE). In addition to the DIS, DY, and SIH datasets, we will also include LHC W/Z production data. As the SIH calculation is dependent on hadronic fragmentation functions (FFs), we use a variety of FFs available in the literature to properly estimate this source of uncertainty. We study the impact of these data on the PDFs and compare with both the nCTEQ15 and nCTEQ15WZ sets. The calculations are performed using a new implementation of the nCTEQ code (ncteq++) including a modified version of incnlo, which allows faster calculations using precomputed grids. The extension of the nCTEQ15 analysis to include the SIH data represents an important step toward the next generation of PDFs.
nCTEQ Collaboration: P. Duwentäster, L. A. Husová, T. Ježo, M. Klasen, Kovařík,, A. Kusina, K. F. Muzakka, F. I. Olness, I. Schienbein, J. Y. Yu, “Impact of inclusive hadron production data on nuclear gluon PDFs”, Phys.Rev.D 104 (2021) 9, 094005.
https://journals.aps.org/prd/abstract/10.1103/PhysRevD.104.094005
Related to Project C05
This talk is meant to introduce the artist Philip Kanwischer who has been selected by a jury to inhabit a 2-month SciArt-Residency in Heidelberg. We will shortly introduce the collaboration of Kalamari Klub and the CRC1225 IsoQuant within the framework of our public relations project and then Philip Kanwischer will present himself and his art work. We will have time for all curious questions and lively discussion after the talk. The talk is open to everyone interested.
https://zoom.us/j/92122638521?pwd=T2tma1dHWE1ydlNjQkJ4Z3Jvc2RJZz09
Abstract:
AWe compute the ghost spectral function in Yang-Mills theory by solving the corresponding Dyson-Schwinger equation for a given input gluon spectral function. The results encompass both scaling and decoupling solutions for the gluon propagator input. The resulting ghost spectral function displays a particle peak at vanishing momentum and a negative scattering spectrum, whose infrared and ultraviolet tails are obtained analytically. The ghost dressing function is computed in the entire complex plane, and its salient features are identified and discussed.
J. Horak, J. Papavassiliou, J. M. Pawlowski, N. Wink, “Ghost spectral function from the spectral Dyson-Schwinger equation”, Phys. Rev. D 104, 074017 (2021).
https://journals.aps.org/prd/abstract/10.1103/PhysRevD.104.074017
Related to Project A02
Abstract:
Quantum-simulator hardware promises new insights into problems from particle and nuclear physics. A major challenge is to reproduce gauge invariance, as violations of this quintessential property of lattice gauge theories can have dramatic consequences, e.g., the generation of a photon mass in quantum electrodynamics. Here, we introduce an experimentally friendly method to protect gauge invariance in U(1) lattice gauge theories against coherent errors in a controllable way. Our method employs only single-body energy-penalty terms, thus enabling practical implementations. As we derive analytically, some sets of penalty coefficients render undesired gauge sectors inaccessible by unitary dynamics for exponentially long times. Further, for few-body error terms, we show numerically that this is achieved with resources exhibiting little dependence on system size. These findings constitute an exponential improvement over previously known results from energy-gap protection or perturbative treatments. In our method, the gauge-invariant subspace is protected by an emergent global symmetry, meaning it can be immediately applied to other symmetries. In our numerical benchmarks for continuous-time and digital quantum simulations, gauge protection holds for all calculated evolution times (up to t>1010/J for continuous time, with J the relevant energy scale). Crucially, our gauge-protection technique is simpler to realize than the associated ideal gauge theory, and can thus be readily implemented in current ultracold-atom analog simulators as well as digital noisy intermediate-scale quantum devices.
J. C. Halimeh, H. Lang, J. Mildenberger, Z. Jiang, P. Hauke, “Gauge-Symmetry Protection Using Single-Body Terms”, PRX Quantum 2, 040311 (2021).
https://journals.aps.org/prxquantum/abstract/10.1103/PRXQuantum.2.040311
Related to Project B04
Why is it that in our minds it is often still “the male scientist” when we think about who actually does science? In the recently launched documentary PICTURE A SCIENTIST, geologist Jane Willenbring, chemist Raychelle Burks, and biologist Nancy Hopkins, as well as key social scientists take on these questions and lead the audience on a journey through the experiences of their academic careers – as women of science. Working to understand and reduce gender bias in the sciences, PICTURE A SCIENTIST brings diversity in science into sharp view at a critical time. The film challenges audiences of all backgrounds and genders to question their own implicit biases and move toward change.
Tuesday, 26 October 2021 at 7pm
KAMERA KINO HEIDELBERG, Brückenstraße 26, 69120 Heidelberg
Film tickets are free of charge for all interested students and employees of the Faculty for Physics and Astronomy. As tickets are limited, prior registration is required:
https://forms.gle/nHk1Dhdo6uT8Bpko6
Following the screening of the film, we invite you to stay for a glass of champagne and a joint discussion/exchange about the film.
The film is going to be in English with German subtitles. We have to obey the current 3G-regulations.
PICTURE A SCIENTIST
Director/Producer: Sharon Shattuck & Ian Cheney; Producer: Manette Pottle
Trailer: https://vimeo.com/ondemand/pictureascientist
Website: https://www.pictureascientist.com
The Boston Globe: “Quietly devastating.”
Science: “Sweeping in scope yet intimately compelling.” The Upcoming: “Sweeping in scope yet intimately compelling.”
WGBH: “The film documents a reckoning both within the field and in our own brains with its fascinating and frightening examination of bias.”
Abstract:
Thermometry of one-dimensional Bose gases can be achieved in various ways, which all revolve around comparing measurement statistics to theoretical prediction. As a result, many repetitions of the measurement are required before an accurate comparison can be made. In this work, we train a neural network to estimate the temperature of a one-dimensional Bose gas in the quasi-condensate regime from a single absorption image. We benchmark our model on both simulated and experimentally measured data. Comparing with our standard method of density ripple thermometry based on fitting two-point correlation function shows that the network can achieve the same precision needing only half the amount of images. Our findings highlight the gain in efficiency when incorporating neural networks into analysis of data from cold gas experiments. Further, the neural network architecture presented can easily be reconfigured to extract other parameters from the images.
F. Møller, T. Schweigler, M. Tajik, J. Sabino, F. Cataldini, S.-C. Ji, J. Schmiedmayer, “Thermometry of one-dimensional Bose gases with neural networks”, Phys. Rev. A 104, 043305 (2021).
https://journals.aps.org/prl/abstract/10.1103/PhysRevLett.128.020402
Related to Project A03
Recently, our former doctoral student and postdoctoral researcher Maximilian Prüfer (Twitter: @max_pruefer) wrote his first science communication article. He describes the general ideas behind quantum simulators from an experimentalist´s point-of-view. It is published in the Many Body Physics-Blog with whom the CRC1225 ISOQUANT collaborates on science communication. The article aims at being accessible to non-physicists and even non-scientists. “My collaboration with Many Body Physics started after an exciting and insightful seminar about science communication from NAWIK which was organized by the CRC1225 ISOQUANT in February 2021. I wanted to write a piece like that already for a long time. The workshop provided me with new skills and the momentum for turning the idea into an article.“
Article: https://manybodyphysics.com/2021/10/03/learning-about-quantum-phenomena-with-quantum-machines/
We have successfully finished our 3rd Internal CRC1225 ISOQUANT workshop starting on Wednesday, October 06 at 12:30hrs with a Welcome Lunch, ending on Friday, October 08 at 16:15 with a Summary Discussion.
The workshop was held in presence at the Institute for Theoretical Physics, Philosophenweg 12 (gHS/ SR105/SR106), respecting the current COVID19 regulations for on-site events (3G obligation, mandatory medical masks in building and on open grounds, if it is not certain that a minimum distance of 1.5 meters from others can be kept). All talks were also streamed online via Zoom as well.
The main aim of the workshop was getting together with all members of the collaboration, discuss and inform each other about the status of the individual part projects, what milestones have been achieved and what still needs to be done to have a second successful 2nd funding period.
You can find the slides of all project talks as well as the presented posters here:
http://indico.physi.uni-heidelberg.de/event/398
password: IsoQ21
Abstract:
Upcoming experiments on the interaction of electrons with intense laser fields are envisaged to become more and more accurate, which calls for theoretical computations of rates and probabilities with correspondingly higher precision. In strong-field QED this requires the knowledge of radiative corrections to be added to leading-order results. Here, we first derive the mass operator in momentum space of an off-shell electron in the presence of an arbitrary plane wave. By taking the average of the mass operator in momentum space over an on-shell electron state, we obtain a new representation, equivalent to but more compact than the known one computed in [V. N. Baier et al., Sov. Phys. JETP 42, 400 (1976).]. Moreover, we use the obtained mass operator to determine the electron mass shift in an arbitrary plane wave, which generalizes the already known expression in a constant crossed field. The spin-dependent part of the electron mass shift can be related to the anomalous magnetic moment of the electron in the plane wave. We show that within the locally constant field approximation it is possible to conveniently define a local expression of the electron anomalous magnetic moment, which reduces to the known expression in a constant crossed field. Beyond the locally constant field approximation, however, the interaction between the electron and the plane wave is nonlocal such that it is not possible to conveniently introduce an electron anomalous magnetic moment.
A. Di Piazza and T. Pătuleanu, “Electron mass shift in an intense plane wave”, Phys. Rev. D 104, 076003 (2021).
https://journals.aps.org/prd/abstract/10.1103/PhysRevD.104.076003
Related to Project B02
Abstract:
he possibility of applying active feedback to a single ion in a Penning trap using a fully digital system is demonstrated. Previously realized feedback systems rely on analog circuits that are susceptible to environmental fluctuations and long term drifts, as well as being limited to the specific task they were designed for. The presented system is implemented using a field-programmable gate array (FPGA)-based platform (STEMlab), offering greater flexibility, higher temporal stability, and the possibility for highly dynamic variation of feedback parameters. The system’s capabilities were demonstrated by applying feedback to the ion detection system primarily consisting of a resonant circuit. This allowed shifts in its resonance frequency of up to several kHz and free modification of its quality factor within two orders of magnitude, which reduces the temperature of a single ion by a factor of 6. Furthermore, a phase-sensitive detection technique for the axial ion oscillation was implemented, which reduces the current measurement time by two orders of magnitude, while simultaneously eliminating model-related systematic uncertainties. The use of FPGA technology allowed the implementation of a fully-featured data acquisition system, making it possible to realize feedback techniques that require constant monitoring of the ion signal. This was successfully used to implement a single-ion self-excited oscillator.
J. Herkenhoff, M. Door, P. Filianin, W. Huang, K. Kromer, D. Lange, R. X. Schüssler, C. Schweiger, S. Eliseev and K. Blaum, “A digital feedback system for advanced ion manipulation techniques in Penning traps”, Rev. Sci. Instrum. 92, 103201 (2021).
https://aip.scitation.org/doi/10.1063/5.0064369
Related to Project B01
Press Release Max-Planck-Institut für Kernphysik Heidelberg
30.09.2021
The Otto Hahn Prize 2021 goes to Klaus Blaum of the Max Planck Institute for Nuclear Physics in Heidelberg. The award is endowed with 50,000 euros and is jointly sponsored by the City of Frankfurt am Main, the German Chemical Society (GDCh) and the German Physical Society (DPG). The award ceremony will take place on 5 November in the festive setting of Frankfurt’s Paulskirche.
“A passion for precision” succinctly characterises the research of physicist Klaus Blaum, who will be awarded the Otto Hahn Prize 2021 this year. His work is pioneering for broad areas of atomic, nuclear and particle physics, especially for the test of the fundamental forces of nature in the microcosm.
“The questions Klaus Blaum deals with are only at first glance far removed from the reality of our lives,” says Lord Mayor Peter Feldmann, describing the award winner’s work. “He is, as a layman might say, the cartographer of the microcosm. With meticulousness and precision, he surveys what forces are at work there. Through him we understand the mechanisms of action of our environment. He proves that working on a small scale, understood in this way, is not small-small – but, on the contrary, virtually challenges our understanding of the world.”
“With his research, Blaum is expanding our knowledge of the fundamental properties of the constituents of the matter that surrounds us,” adds Lutz Schröter, President of the German Physical Society. Blaum’s research activities are wide-ranging and can best be summarised as the “study of exotic particles and states”. This includes studies of highly charged ions, short-lived atomic nuclei, antimatter and the heaviest artificial elements.
“With Klaus Blaum, an exceptional scientist receives the Otto Hahn Prize,” says Peter R. Schreiner, President of the German Chemical Society. “The findings from his work also create important foundations for chemical research.”
Today, the properties of elementary particles and the forces acting between them are often studied at the highest energies. However, a number of fundamental questions in particle physics and cosmology can be pursued particularly well at low energies.
Since the effects here are usually extraordinarily tiny, the highest precision is required. To this end, Blaum and his group developed a large number of sophisticated techniques and often carried out the experiments on individual particles at the lowest temperatures. Using a series of brilliant ideas and exceptional experimental skills, he combined sophisticated techniques from atomic, nuclear and accelerator physics.
Blaum published his scientific results in more than 450 scientific articles in the leading and most internationally recognised physics journals. Although he is considered young in scientific circles at the age of 49, he is already one of the world’s most productive and cited researchers in the field of precision physics and metrology.
Klaus Blaum was born on 27 December 1971 in Bad Sobernheim in Rheinland-Pfalz. He studied physics at the Johannes Gutenberg University in Mainz, where, after receiving his diploma in 1997 and several research stays at the Pacific Northwest National Laboratory (PNNL) in Richland, USA, he received his doctorate in 2000 under Ernst-Wilhelm Otten (1934 – 2019). Subsequently, he was a research associate at the GSI Helmholtz Centre for Heavy Ion Research in Darmstadt until 2002 and worked at the European Nuclear Research Centre CERN near Geneva. There he was project leader for “Mass Spectrometry of Exotic Nuclei with ISOLTRAP at ISOLDE” until 2004. In October 2004, Blaum took over the position of project leader of the Helmholtz-University Young Investigators Group “Experiments with Stored and Cooled Ions” at the Johannes Gutenberg University Mainz for four years. In 2006 he habilitated there on high-precision mass spectrometry with Penning traps for charged particles and storage rings.
Blaum taught at the University of Mainz from 2004 to 2008. He was awarded the 2006 teaching prize of the state of Rheinland-Pfalz for his teaching activities. In October 2007, at the age of only 35, he was appointed Director and Scientific Member of the Max Planck Institute for Nuclear Physics in Heidelberg. This was followed in April 2008 by his appointment as Honorary Professor (W3) of the Ruprecht Karls University in Heidelberg. Since July 2020, Blaum has been Vice President of the Max Planck Society, responsible for the institutes of the Chemical-Physical-Technical Section.
At a young age, Blaum was awarded a number of highly prestigious prizes, including the Gustav Hertz Prize of the German Physical Society in 2004 for his outstanding work on the mass determination of unstable atomic nuclei, as well as the Helmholtz Prize of the Physikalisch-Technische Bundesanstalt (PTB) in 2012 and the Lise Meitner Prize of the European Physical Society (EPS) in 2020. In 2019, he was admitted to the physics class of the “Royal Swedish Academy of Sciences” as a foreign member.
The Otto Hahn Prize is awarded jointly by the City of Frankfurt am Main, the German Physical Society (DPG) and the German Chemical Society (GDCh). It serves to promote science, especially in the fields of chemistry, physics and applied engineering sciences, by recognising outstanding scientific achievements. It is endowed with 50,000 euros and is awarded every two years with a ceremony in Frankfurt’s Paulskirche.
[DPG/GDCh/Stadt Frankfurt]
Contact
Prof. Dr. Klaus Blaum
Phone: +49 6221 516-859
Email: klaus.blaum@mpi-hd.mpg.de
Der Otto-Hahn-Preis 2021 geht an den Kernphysiker Klaus Blaum vom Max-Planck-Institut für Kernphysik in Heidelberg. Die Auszeichnung ist mit 50 000 Euro dotiert und wird gemeinsam von der Stadt Frankfurt am Main, der Gesellschaft Deutscher Chemiker (GDCh) und der Deutschen Physikalischen Gesellschaft (DPG) getragen. Die Verleihung erfolgt am 5. November im festlichen Rahmen der Frankfurter Paulskirche.
Frankfurt am Main / Bad Honnef, 30. September 2021 – „Eine Passion für Präzision“ charakterisiert in prägnanter Form die Forschung des Physikers Klaus Blaum, der in diesem Jahr mit dem Otto-Hahn-Preis 2021 ausgezeichnet wird. Seine Arbeiten sind wegbereitend für weite Bereiche der Atom-, Kern- und Teilchenphysik, insbesondere für den Test der fundamentalen Kräfte der Natur im Mikrokosmos.
„Die Fragen, mit denen sich Klaus Blaum auseinandersetzt, sind nur auf den ersten Blick weit weg von unserer Lebenswirklichkeit“, beschreibt Oberbürgermeister Peter Feldmann die Arbeiten des Preisträgers. „Er ist, wie man als Laie vielleicht sagen könnte, der Kartograph des Mikrokosmos. Mit Akribie und Genauigkeit vermisst er, welche Kräfte dort walten. Durch ihn begreifen wir die Wirkmechanismen unserer Umwelt. Er beweist, dass die so verstandene Arbeit im Kleinen eben nicht klein-klein ist – sondern, ganz im Gegenteil, unser Verständnis von Welt geradezu herausfordert.“
„Mit seiner Forschung erweitert Blaum unsere Erkenntnisse von den grundlegenden Eigenschaften der Bestandteile der uns umgebenden Materie“, ergänzt Lutz Schröter, Präsident der Deutschen Physikalischen Gesellschaft. Die Forschungsaktivitäten von Blaum sind weit gefächert und lassen sich am besten mit dem „Studium exotischer Teilchen und Zustände“ zusammenfassen. Dazu gehören Untersuchungen an hochgeladenen Ionen, an kurzlebigen Atomkernen, an Antimaterie sowie an schwersten, künstlichen Elementen.
„Mit Klaus Blaum erhält ein Ausnahmewissenschaftler den Otto-Hahn-Preis”, sagt Peter R. Schreiner, Präsident der Gesellschaft Deutscher Chemiker. „Die Erkenntnisse aus seinen Arbeiten schaffen auch für die chemische Forschung wichtige Grundlagen.“
Heute werden die Eigenschaften elementarer Teilchen und die zwischen ihnen wirkenden Kräfte oft bei höchsten Energien untersucht. Eine Reihe grundlegender Fragen der Teilchenphysik und der Kosmologie lassen sich aber besonders gut bei niedrigen Energien verfolgen.
Da hier die Effekte in der Regel außerordentlich winzig sind, ist höchste Präzision gefordert. Dazu entwickelte Blaum mit seiner Gruppe eine große Anzahl ausgeklügelter Techniken und führte die Experimente oft nur an einzelnen Teilchen bei tiefsten Temperaturen durch. Mittels einer Reihe brillanter Ideen und durch außergewöhnliches Experimentiergeschick kombinierte er anspruchsvolle Techniken der Atom-, Kern- und Beschleunigerphysik.
Blaum hat seine wissenschaftlichen Ergebnisse in mehr als 450 wissenschaftlichen Artikeln in den führenden und international höchst anerkannten Physikzeitschriften veröffentlicht. Obgleich er mit 49 Jahren in Wissenschaftskreisen als jung gilt, gehört er bereits zu den weltweit produktivsten und meistzitierten Forscherinnen und Forschern auf dem Gebiet der Präzisionsphysik und Messtechnik.
Klaus Blaum wurde am 27. Dezember 1971 in Bad Sobernheim in Rheinland-Pfalz geboren. Er studierte Physik an der Johannes Gutenberg-Universität Mainz, wo er nach dem Diplom 1997 und mehreren Forschungsaufenthalten am Pacific Northwest National Laboratory (PNNL) in Richland, USA, im Jahr 2000 bei Ernst-Wilhelm Otten (1934 – 2019) promoviert wurde. Im Anschluss war er bis 2002 wissenschaftlicher Mitarbeiter am GSI Helmholtzzentrum für Schwerionenforschung in Darmstadt und arbeitete am Europäischen Kernforschungszentrum CERN bei Genf. Dort war er bis 2004 Projektleiter für „Massenspektrometrie exotischer Kerne mit ISOLTRAP an ISOLDE“. Im Oktober 2004 übernahm Blaum für vier Jahre die Position des Projektleiters der Helmholtz-Hochschul-Nachwuchsgruppe „Experimente mit gespeicherten und gekühlten Ionen“ an der Johannes-Gutenberg-Universität Mainz. 2006 habilitierte er sich dort über Hochpräzisionsmassenspektrometrie mit Penningfallen für geladene Teilchen und Speicherringen.
Blaum lehrte von 2004 bis 2008 an der Universität Mainz. Für seine Lehrtätigkeit wurde ihm der Lehrpreis des Landes Rheinland-Pfalz 2006 verliehen. Im Oktober 2007 erhielt er im Alter von nur 35 Jahren die Berufung zum Direktor und wissenschaftlichen Mitglied des Max-Planck-Instituts für Kernphysik in Heidelberg. Im April 2008 folgte seine Berufung zum Honorarprofessor (W3) der Ruprecht-Karls-Universität Heidelberg. Seit Juli 2020 ist Blaum als Vizepräsident der Max-Planck-Gesellschaft zuständig für die Institute der Chemisch-Physikalisch-Technischen Sektion.
Schon in jungen Jahren wurde Blaum mit einer Reihe von hoch angesehenen Preisen ausgezeichnet, darunter 2004 mit dem Gustav-Hertz-Preis der Deutschen Physikalischen Gesellschaft für seine hervorragenden Arbeiten über die Massenbestimmung instabiler Atomkerne sowie 2012 mit dem Helmholtz-Preis der Physikalisch-Technischen Bundesanstalt (PTB) und 2020 mit dem Lise-Meitner-Preis der Europäischen Physikalischen Gesellschaft (EPS). 2019 wurde er als Auswärtiges Mitglied in die Physikklasse der “Royal Swedish Academy of Sciences” aufgenommen.
Der Otto-Hahn-Preis wird gemeinsam von der Stadt Frankfurt am Main, der Deutschen Physikalischen Gesellschaft (DPG) und der Gesellschaft Deutscher Chemiker (GDCh) verliehen. Er dient der Förderung der Wissenschaft insbesondere auf den Gebieten der Chemie, Physik und der angewandten Ingenieurwissenschaften durch die Anerkennung herausragender wissenschaftlicher Leistungen. Er ist mit 50 000 Euro dotiert und wird alle zwei Jahre mit einem Festakt in der Frankfurter Paulskirche verliehen.
Gemeinsame Pressemitteilung der Stadt Frankfurt am Main der Gesellschaft Deutscher Chemiker e. V. (GDCh) sowie der Deutschen Physikalischen Gesellschaft e. V. (DPG) vom 30.09.2021
https://www.dpg-physik.de/veroeffentlichungen/aktuell/2021/eine-passion-fuer-praezision
Die Deutsche Physikalische Gesellschaft e. V. (DPG), deren Tradition bis in das Jahr 1845 zurückreicht, ist die älteste nationale und mit rund 55.000 Mitgliedern auch mitgliederstärkste physikalische Fachgesellschaft der Welt. Als gemeinnütziger Verein verfolgt sie keine wirtschaftlichen Interessen. Die DPG fördert mit Tagungen, Veranstaltungen und Publikationen den Wissenstransfer innerhalb der wissenschaftlichen Gemeinschaft und möchte allen Neugierigen ein Fenster zur Physik öffnen. Besondere Schwerpunkte sind die Förderung des naturwissenschaftlichen Nachwuchses und der Chancengleichheit. Sitz der DPG ist Bad Honnef am Rhein. Hauptstadtrepräsentanz ist das Magnus-Haus Berlin. Website: www.dpg-physik.de
Der Otto-Hahn-Preis 2021 geht an den Kernphysiker Klaus Blaum vom Max-Planck-Institut für Kernphysik in Heidelberg. Die Auszeichnung ist mit 50 000 Euro dotiert und wird gemeinsam von der Stadt Frankfurt am Main, der Gesellschaft Deutscher Chemiker (GDCh) und der Deutschen Physikalischen Gesellschaft (DPG) getragen. Die Verleihung erfolgt am 5. November im festlichen Rahmen der Frankfurter Paulskirche.
Frankfurt am Main / Bad Honnef, 30. September 2021 – „Eine Passion für Präzision“ charakterisiert in prägnanter Form die Forschung des Physikers Klaus Blaum, der in diesem Jahr mit dem Otto-Hahn-Preis 2021 ausgezeichnet wird. Seine Arbeiten sind wegbereitend für weite Bereiche der Atom-, Kern- und Teilchenphysik, insbesondere für den Test der fundamentalen Kräfte der Natur im Mikrokosmos.
„Die Fragen, mit denen sich Klaus Blaum auseinandersetzt, sind nur auf den ersten Blick weit weg von unserer Lebenswirklichkeit“, beschreibt Oberbürgermeister Peter Feldmann die Arbeiten des Preisträgers. „Er ist, wie man als Laie vielleicht sagen könnte, der Kartograph des Mikrokosmos. Mit Akribie und Genauigkeit vermisst er, welche Kräfte dort walten. Durch ihn begreifen wir die Wirkmechanismen unserer Umwelt. Er beweist, dass die so verstandene Arbeit im Kleinen eben nicht klein-klein ist – sondern, ganz im Gegenteil, unser Verständnis von Welt geradezu herausfordert.“
„Mit seiner Forschung erweitert Blaum unsere Erkenntnisse von den grundlegenden Eigenschaften der Bestandteile der uns umgebenden Materie“, ergänzt Lutz Schröter, Präsident der Deutschen Physikalischen Gesellschaft. Die Forschungsaktivitäten von Blaum sind weit gefächert und lassen sich am besten mit dem „Studium exotischer Teilchen und Zustände“ zusammenfassen. Dazu gehören Untersuchungen an hochgeladenen Ionen, an kurzlebigen Atomkernen, an Antimaterie sowie an schwersten, künstlichen Elementen.
„Mit Klaus Blaum erhält ein Ausnahmewissenschaftler den Otto-Hahn-Preis”, sagt Peter R. Schreiner, Präsident der Gesellschaft Deutscher Chemiker. „Die Erkenntnisse aus seinen Arbeiten schaffen auch für die chemische Forschung wichtige Grundlagen.“
Heute werden die Eigenschaften elementarer Teilchen und die zwischen ihnen wirkenden Kräfte oft bei höchsten Energien untersucht. Eine Reihe grundlegender Fragen der Teilchenphysik und der Kosmologie lassen sich aber besonders gut bei niedrigen Energien verfolgen.
Da hier die Effekte in der Regel außerordentlich winzig sind, ist höchste Präzision gefordert. Dazu entwickelte Blaum mit seiner Gruppe eine große Anzahl ausgeklügelter Techniken und führte die Experimente oft nur an einzelnen Teilchen bei tiefsten Temperaturen durch. Mittels einer Reihe brillanter Ideen und durch außergewöhnliches Experimentiergeschick kombinierte er anspruchsvolle Techniken der Atom-, Kern- und Beschleunigerphysik.
Blaum hat seine wissenschaftlichen Ergebnisse in mehr als 450 wissenschaftlichen Artikeln in den führenden und international höchst anerkannten Physikzeitschriften veröffentlicht. Obgleich er mit 49 Jahren in Wissenschaftskreisen als jung gilt, gehört er bereits zu den weltweit produktivsten und meistzitierten Forscherinnen und Forschern auf dem Gebiet der Präzisionsphysik und Messtechnik.
Klaus Blaum wurde am 27. Dezember 1971 in Bad Sobernheim in Rheinland-Pfalz geboren. Er studierte Physik an der Johannes Gutenberg-Universität Mainz, wo er nach dem Diplom 1997 und mehreren Forschungsaufenthalten am Pacific Northwest National Laboratory (PNNL) in Richland, USA, im Jahr 2000 bei Ernst-Wilhelm Otten (1934 – 2019) promoviert wurde. Im Anschluss war er bis 2002 wissenschaftlicher Mitarbeiter am GSI Helmholtzzentrum für Schwerionenforschung in Darmstadt und arbeitete am Europäischen Kernforschungszentrum CERN bei Genf. Dort war er bis 2004 Projektleiter für „Massenspektrometrie exotischer Kerne mit ISOLTRAP an ISOLDE“. Im Oktober 2004 übernahm Blaum für vier Jahre die Position des Projektleiters der Helmholtz-Hochschul-Nachwuchsgruppe „Experimente mit gespeicherten und gekühlten Ionen“ an der Johannes-Gutenberg-Universität Mainz. 2006 habilitierte er sich dort über Hochpräzisionsmassenspektrometrie mit Penningfallen für geladene Teilchen und Speicherringen.
Blaum lehrte von 2004 bis 2008 an der Universität Mainz. Für seine Lehrtätigkeit wurde ihm der Lehrpreis des Landes Rheinland-Pfalz 2006 verliehen. Im Oktober 2007 erhielt er im Alter von nur 35 Jahren die Berufung zum Direktor und wissenschaftlichen Mitglied des Max-Planck-Instituts für Kernphysik in Heidelberg. Im April 2008 folgte seine Berufung zum Honorarprofessor (W3) der Ruprecht-Karls-Universität Heidelberg. Seit Juli 2020 ist Blaum als Vizepräsident der Max-Planck-Gesellschaft zuständig für die Institute der Chemisch-Physikalisch-Technischen Sektion.
Schon in jungen Jahren wurde Blaum mit einer Reihe von hoch angesehenen Preisen ausgezeichnet, darunter 2004 mit dem Gustav-Hertz-Preis der Deutschen Physikalischen Gesellschaft für seine hervorragenden Arbeiten über die Massenbestimmung instabiler Atomkerne sowie 2012 mit dem Helmholtz-Preis der Physikalisch-Technischen Bundesanstalt (PTB) und 2020 mit dem Lise-Meitner-Preis der Europäischen Physikalischen Gesellschaft (EPS). 2019 wurde er als Auswärtiges Mitglied in die Physikklasse der “Royal Swedish Academy of Sciences” aufgenommen.
Der Otto-Hahn-Preis wird gemeinsam von der Stadt Frankfurt am Main, der Deutschen Physikalischen Gesellschaft (DPG) und der Gesellschaft Deutscher Chemiker (GDCh) verliehen. Er dient der Förderung der Wissenschaft insbesondere auf den Gebieten der Chemie, Physik und der angewandten Ingenieurwissenschaften durch die Anerkennung herausragender wissenschaftlicher Leistungen. Er ist mit 50 000 Euro dotiert und wird alle zwei Jahre mit einem Festakt in der Frankfurter Paulskirche verliehen.
Gemeinsame Pressemitteilung der Stadt Frankfurt am Main der Gesellschaft Deutscher Chemiker e. V. (GDCh) sowie der Deutschen Physikalischen Gesellschaft e. V. (DPG) vom 30.09.2021
https://www.dpg-physik.de/veroeffentlichungen/aktuell/2021/eine-passion-fuer-praezision
Die Deutsche Physikalische Gesellschaft e. V. (DPG), deren Tradition bis in das Jahr 1845 zurückreicht, ist die älteste nationale und mit rund 55.000 Mitgliedern auch mitgliederstärkste physikalische Fachgesellschaft der Welt. Als gemeinnütziger Verein verfolgt sie keine wirtschaftlichen Interessen. Die DPG fördert mit Tagungen, Veranstaltungen und Publikationen den Wissenstransfer innerhalb der wissenschaftlichen Gemeinschaft und möchte allen Neugierigen ein Fenster zur Physik öffnen. Besondere Schwerpunkte sind die Förderung des naturwissenschaftlichen Nachwuchses und der Chancengleichheit. Sitz der DPG ist Bad Honnef am Rhein. Hauptstadtrepräsentanz ist das Magnus-Haus Berlin. Website: www.dpg-physik.de
Abstract:
Dynamical quantum phase transitions (DQPTs) feature singular temporal behavior in transient quantum states during nonequilibrium real-time evolution. In this work we show that DQPTs in random Ising chains exhibit critical behavior with nontrivial exponents that are not integer valued and not of mean-field type. By means of an exact renormalization group transformation we estimate the exponents with high accuracy eliminating largely any finite-size effects. We further discuss how the considered dynamical phenomena can be made accessible in current Rydberg atom platforms. In this context we explore signatures of the DQPTs in the statistics of spin configuration measurements available in such architectures. Specifically, we study the statistics of clusters of consecutively aligned spins and observe a marked influence of the DQPT on the corresponding distribution.
https://journals.aps.org/prb/abstract/10.1103/PhysRevB.104.115159
Related to Project: B04
Abstract:
We propose a scalable analog quantum simulator for quantum electrodynamics (QED) in two spatial dimensions. The setup for the U(1) lattice gauge field theory employs inter-species spin-changing collisions in an ultra-cold atomic mixture trapped in an optical lattice. Building on the previous one-dimensional implementation scheme of Ref. [1] we engineer spatial plaquette terms for magnetic fields, thus solving a major obstacle towards experimental realizations of realistic gauge theories in higher dimensions. We apply our approach to pure gauge theory and discuss how the phenomenon of confinement of electric charges can be described by the quantum simulator.
R. Ott, T. V. Zache, F. Jendrzejewski, J. Berges, “Scalable cold-atom quantum simulator for two-dimensional QED”, Phys. Rev. Lett. 127, 130504 (2021).
https://journals.aps.org/prl/abstract/10.1103/PhysRevLett.127.130504
Related to Project B03, B04
Abstract:
Inspired by topological data analysis techniques, we introduce persistent homology observables and apply them in a geometric analysis of the dynamics of quantum field theories. As a prototype application, we consider data from a classical-statistical simulation of a two-dimensional Bose gas far from equilibrium. We discover a continuous spectrum of dynamical scaling exponents, which provides a refined classification of nonequilibrium self-similar phenomena. A possible explanation of the underlying processes is provided in terms of mixing strong wave turbulence and anomalous vortex kinetics components in point clouds. We find that the persistent homology scaling exponents are inherently linked to the geometry of the system, as the derivation of a packing relation reveals. The approach opens new ways of analyzing quantum many-body dynamics in terms of robust topological structures beyond standard field theoretic techniques.
D. Spitz and J. Berges and M. K. Oberthaler and A. Wienhard, “Finding self-similar behavior in quantum many-body dynamics via persistent homology”, SciPost Phys. 11, 60 (2021).
https://scipost.org/10.21468/SciPostPhys.11.3.060
Related to Project B04, B03, A01, A03
Abstract:
n local scalar quantum field theories at finite temperature correlation functions are known to satisfy certain nonperturbative constraints, which for two-point functions in particular implies the existence of a generalization of the standard Källén-Lehmann representation. In this work, we use these constraints in order to derive a spectral representation for the shear viscosity arising from the thermal asymptotic states, η0. As an example, we calculate η0 in ϕ4 theory, establishing its leading behavior in the small and large coupling regimes.
P. Lowdon, R.-A. Tripolt, J. M. Pawlowski, D. H. Rischke, “Spectral representation of the shear viscosity for local scalar QFTs at finite temperature”, Phys. Rev. D 104, 065010 (2021).
https://journals.aps.org/prd/abstract/10.1103/PhysRevD.104.065010
Related to Project A02
Abstract:
We map out the QCD phase structure at finite temperature and chemical potential for 2-flavour and 2+1-flavour QCD. This is done within a generalised functional approach to QCD put forward in [1]. Specifically we compute the quark propagator and the finite-temperature and density fluctuations of the gluon propagator and the quark-gluon vertex on the basis of precision data for vacuum correlation functions. The novel ingredient is the direct self-consistent computation of the DSEs for the three dominant dressings of the quark-gluon vertex, in contrast to the common use of STI-inspired vertices.
For small densities the results for the chiral order parameter agree with the respective lattice and functional renormalisation group results, for large densities the present results are in a quantitative agreement with the latter, including the location of the critical end point.
F. Gao, J. M. Pawlowski, “Chiral phase structure and critical end point in QCD”, Phys.
Lett. B 820, 136584 (2021).
https://www.sciencedirect.com/science/article/pii/S0370269321005244?via%3Dihub
Related to Project A02
Abstract:
Recently, two scenarios have been proposed to resolve the discrepancy between photon yield and the flow coefficients measured in nuclear collisions at RHIC and the LHC. In the first, additional photons are produced from the early pre-equilibrium stage computed from the “bottom-up” thermalization scenario. In the second, the thermal rates are enhanced close to the pseudo-critical temperature Tc∼ 155MeV using a phenomenological ansatz. We investigate the measurement of Hanbury Brown-Twiss (HBT) photon correlations as an experimental tool to discriminate between such scenarios. By enhancing standard hydrodynamical simulations with these scenarios, we compute the correlators in terms of the relative momenta of the pair for different values of its transverse momenta, K⊥ We find that the longitudinal correlation is the most sensitive to different photon sources.
O. Garcia Montero, N. Löher, A. Mazeliauskas, K. Reygers and J. Berges, “Untangling the evolution of heavy ion collisions using direct photon interferometry”, PoS HardProbes2020, 033 (2021).
Related to Project A01, A02
Abstract:
Multipartite entanglement, such as witnessed through the quantum Fisher information (QFI), is a crucial resource for quantum technologies, but its experimental certification is highly challenging. Here, we propose an experimentally friendly protocol to measure the QFI. It relies on recording the short-time dynamics of simple observables after a quench from a thermal state, works for spins, bosons, and fermions, and can be implemented in standard cold-atom experiments and other platforms with temporal control over the system Hamiltonian. To showcase the protocol, we simulate it for the one-dimensional Fermi-Hubbard model. Further, we establish a family of bounds connecting the QFI to multipartite mode entanglement for fermionic systems, which enable the detection of multipartite entanglement at sizable temperatures. Our work paves a way to experimentally accessing entanglement for quantum enhanced metrology.
R. Costa de Almeida, P. Hauke, “From entanglement certification with quench dynamics to multipartite entanglement of interacting fermions”, Phys. Rev. Res. 3, L032051 (2021).
https://journals.aps.org/prresearch/abstract/10.1103/PhysRevResearch.3.L032051
Related to Project: A06*
Abstract:
Starting from nonequilibrium quantum field theory on a closed time path, we derive kinetic equations for the strong-field regime of quantum electrodynamics (QED) using a systematic expansion in the gauge coupling e. The strong field regime is characterized by a large photon field of order O(1/e), which is relevant for the description of, e.g., intense laser fields, the initial stages of off-central heavy ion collisions, and condensed matter systems with net fermion number. The strong field enters the dynamical equations via both quantum Vlasov and collision terms, which we derive to order O(e2). The kinetic equations feature generalized scattering amplitudes that have their own equation of motion in terms of the fermion spectral function. The description includes single photon emission, electron-positron pair photoproduction, vacuum (Schwinger) pair production, their inverse processes, medium effects and contributions from the field, which are not restricted to the so-called locally-constant crossed field approximation. This extends known kinetic equations commonly used in strong-field QED of intense laser fields. In particular, we derive an expression for the asymptotic fermion pair number that includes leading-order collisions and remains valid for strongly inhomogeneous fields. For the purpose of analytically highlighting limiting cases, we also consider plane-wave fields for which it is shown how to recover Furry-picture scattering amplitudes by further assuming negligible occupations. Known on-shell descriptions are recovered in the case of simply peaked ultrarelativistic fermion occupations. Collisional strong-field equations are necessary to describe the dynamics to thermal equilibrium starting from strong-field initial conditions.
G. Fauth, J. Berges, A. Di Piazza, “Collisional strong-field QED kinetic equations from first principles”, Phys. Rev. D 104, 036007 (2021).
https://journals.aps.org/prd/abstract/10.1103/PhysRevD.104.036007
Related to Project B03, B02, A01
Abstract:
We present a novel ultrastable superconducting radio-frequency (RF) ion trap realized as a combination of an RF cavity and a linear Paul trap. Its RF quadrupole mode at 34.52 MHz reaches a quality factor of Q≈2.3×105 at a temperature of 4.1 K and is used to radially confine ions in an ultralow-noise pseudopotential. This concept is expected to strongly suppress motional heating rates and related frequency shifts which limit the ultimate accuracy achieved in advanced ion traps for frequency metrology. Running with its low-vibration cryogenic cooling system, electron beam ion trap and deceleration beamline supplying highly charged ions (HCI), the superconducting trap offers ideal conditions for optical frequency metrology with ionic species. We report its proof-of-principle operation as a quadrupole mass filter with HCI, and trapping of Doppler-cooled 9Be+ Coulomb crystals.
J. Stark, C. Warnecke, S. Bogen, S. Chen, E. A. Dijck, S. Kühn, M. K. Rosner, A. Graf, J. Nauta, J.-H. Oelmann, L. Schmöger, M. Schwarz, D. Liebert, L. J. Spieß, S. A. King, T. Leopold, P. Micke, P. O. Schmidt, T. Pfeifer, and J. R. Crespo López-Urrutia, “An ultralownoise superconducting radio-frequency ion trap for frequency metrology with highly charged ions”, Rev.Sci. Instrum. 92, 083203 (2021).
https://aip.scitation.org/doi/10.1063/5.0046569
Related to Project B01
Abstract:
The forthcoming generation of multi-petawatt lasers opens the way to abundant pair production by the nonlinear Breit–Wheeler process, i.e. the decay of a photon into an electron–positron pair inside an intense laser field. In this paper we explore the optimal conditions for Breit–Wheeler pair production in the head-on collision of a laser pulse with gamma photons. The role of the laser peak intensity versus the focal spot size and shape is examined keeping a constant laser energy to match experimental constraints. A simple model for the soft-shower case, where most pairs originate from the decay of the initial gamma photons, is derived. This approach provides us with a semi-analytical model for more complex situations involving either Gaussian or Laguerre–Gauss (LG) laser beams. We then explore the influence of the order of the LG beams on pair creation. Finally we obtain the result that, above a given threshold, a larger spot size (or a higher order in the case of LG laser beams) is more favorable than a higher peak intensity. Our results match very well with three-dimensional particle-in-cell simulations and can be used to guide upcoming experimental campaigns.
A. Mercuri-Baron, M. Grech, F. Niel, A. Grassi, M. Lobet, A. Di Piazza, C. Riconda, “Impact of the laser spatio-temporal shape on Breit-Wheeler pair production”, New J. of Phys. 23, 085006 (2021).
https://iopscience.iop.org/article/10.1088/1367-2630/ac1975
Related to Project B02
In recent years, dynamical quantum phase transitions (DQPTs) have emerged as a useful theoretical concept to characterize nonequilibrium states of quantum matter. DQPTs are marked by singular behavior in an effective free energy λ(t), which, however, is a global measure, making its experimental or theoretical detection challenging in general. We introduce two local measures for the detection of DQPTs with the advantage of requiring fewer resources than the full effective free energy. The first, called the real-local effective free energy λM(t), is defined in real space and is therefore suitable for systems where locally resolved measurements are directly accessible such as in quantum-simulator experiments involving Rydberg atoms or trapped ions. We test λM(t) in Ising chains with nearest-neighbor and power-law interactions, and find that this measure allows extraction of the universal critical behavior of DQPTs. The second measure we introduce is the momentum-local effective free energy λk(t), which is targeted at systems where momentum-resolved quantities are more naturally accessible, such as through time-of-flight measurements in ultracold atoms. We benchmark λk(t) for the Kitaev chain, a paradigmatic system for topological quantum matter, in the presence of weak interactions. Our introduced local measures for effective free energies can further facilitate the detection of DQPTs in modern quantum-simulator experiments.
J. C. Halimeh, D. Trapin, M. Van Damme, M. Heyl, “Local measures of dynamical quantum phase transitions”, Phys. Rev. B 104, 075130 (2021).
https://journals.aps.org/prb/abstract/10.1103/PhysRevB.104.075130
Related to Project B04
Abstract:
We report calculations of QED corrections to the g factor of Li-like ions induced by the exchange of two virtual photons between the electrons. The calculations are performed within QED theory to all orders of the nuclear binding strength parameter Zα, where Z is the nuclear charge number and α is the fine-structure constant. In the region of low nuclear charges we compare results from three different methods: QED, relativistic many-body perturbation theory, and nonrelativistic QED. All three methods are shown to yield consistent results. With our calculations we improve the accuracy of theoretical predictions of the g factor of the ground state of Li-like carbon and oxygen by about an order of magnitude. Our theoretical results agree with those from previous calculations but differ by three to four standard deviations from the experimental results available for silicon and calcium.
V. A. Yerokhin, C. H. Keitel, and Z. Harman, “Two-photon-exchange corrections to the g factor of Li-like ions”, Phys. Rev. A 104, 022814 (2021).
https://journals.aps.org/pra/abstract/10.1103/PhysRevA.104.022814
Related to Project B02
Abstract:
The cyclotron frequency ratio of 187Os29+ to 187Re29+ ions was measured with the Penning-trap mass spectrometer PENTATRAP. The achieved result of R=1.000 000 013 882(5) is to date the most precise such measurement performed on ions. Furthermore, the total binding-energy difference of the 29 missing electrons in Re and Os was calculated by relativistic multiconfiguration methods, yielding the value of ΔE=53.5(10) eV. Finally, using the achieved results, the mass difference between neutral 187Re and 187Os, i.e., the Q value of the β− decay of 187Re, is determined to be 2470.9(13) eV.
P. Filianin, C. Lyu, M. Door, K. Blaum, W. J. Huang, M. Haverkort, P. Indelicato, C. H. Keitel, K. Kromer, D. Lange, Y. N. Novikov, A. Rischka, R. X. Schüssler, Ch. Schweiger, S. Sturm, S. Ulmer, Z. Harman, and S. Eliseev, “Direct Q-Value Determination of the β− Decay of 187Re”, Phys. Rev. Lett. 127, 072502 (2021).
https://journals.aps.org/prl/abstract/10.1103/PhysRevLett.127.072502
Related to Project B01, B02
Abstract:
Heavy-ion collisions at BNL’s Relativistic Heavy Ion Collider and CERN’s Large Hadron Collider provide strong evidence for the formation of a quark-gluon plasma, with temperatures extracted from relativistic viscous hydrodynamic simulations shown to be well above the transition temperature from hadron matter. Outstanding problems in QCD include how the strongly correlated quark-gluon matter forms in a heavy-ion collision, its properties off equilibrium, and the thermalization process in the plasma. The theoretical progress in this field in weak-coupling QCD effective field theories and in strong-coupling holographic approaches based on gauge-gravity duality is reviewed. The interdisciplinary connections of different stages of the thermalization process to nonequilibrium dynamics in other systems across energy scales ranging from inflationary cosmology to strong-field QED to ultracold atomic gases are outlined, with emphasis placed on the universal dynamics of nonthermal and hydrodynamic attractors. Measurements in heavy-ion collisions are surveyed that are sensitive to the early nonequilibrium stages of the collision and the potential for future measurements is discussed. The current state of the art in thermalization studies is summarized and promising avenues for further progress are identified.
https://journals.aps.org/rmp/abstract/10.1103/RevModPhys.93.035003
Related to Project: A01, A02, C06, B04, A03, A04
Abstract:
Cosmological reheating describes the transition of the postinflationary universe to a hot and thermal state. In order to shed light on the underlying dynamics of this process, we propose to quantum-simulate the reheating-like dynamics of a generic cosmological single-field model in an ultracold Bose gas. In our setup, the excitations on top of an atomic Bose-Einstein condensate play the role of the particles produced by the decaying inflaton field after inflation. Expanding spacetime as well as the background oscillating inflaton field are mimicked in the nonrelativistic limit by a time dependence of the atomic interactions, which can be tuned experimentally via Feshbach resonances. As we illustrate by means of classical-statistical simulations for the case of two spatial dimensions, the dynamics of the atomic system exhibits the characteristic stages of far-from-equilibrium reheating, including the amplification of fluctuations via parametric instabilities and the subsequent turbulent transport of energy towards higher momenta. The transport is governed by a nonthermal fixed point showing universal self-similar time evolution as well as a transient regime of prescaling with time-dependent scaling exponents. While the classical-statistical simulations can capture only the earlier stages of the dynamics for weak couplings, the proposed experiment has the potential of exploring the evolution up to late times even beyond the weak coupling regime.
A. Chatrchyan, K. Geier, M. K. Oberthaler, J. Berges, and P. Hauke, “Analog cosmological reheating in an ultracold Bose gas”, Phys. Rev. A 104, 023302 (2021).
https://journals.aps.org/pra/abstract/10.1103/PhysRevA.104.023302
Related to Project B04, B03, A06
Abstract:
We propose a novel scheme for coupling a Rydberg state to a stationary light polariton, based on a dual-V level scheme. We investigate the properties of the resulting stationary Rydberg polariton, and show that its form and its quadratic dispersion relation closely resemble that of the stationary light polariton of the underlying dual-V scheme. We consider the influence of a Rydberg impurity on the system and find strong interaction-induced absorption of the involved probe field. The proposed scheme for a stationary Rydberg polariton might find applications for realizing interacting polaritons with increased interaction time.
A. Tebben, C. Hainaut, A. Salzinger, T. Franz, S. Geier, G. Zürn, M.Weidemüller, “A stationary Rydberg polariton”, arXiv:2108.00657 (2021).
https://arxiv.org/abs/2108.00657
Related to Project A05
Abstract:
We discuss the phase structure of the two-flavour quark-meson model including quantum, thermal, density and critical fluctuations with the functional renormalisation group. This study combines two technical advances in the literature, that are also chiefly important for the quantitative access of the phase boundary of QCD at large density or baryon chemical potential. Specifically we allow for the formation and propagation of shocks as well as a fully self-consistent computation of the order parameter potential for chiral symmetry breaking.
E. Grossi, F. J. Ihssen, J. M. Pawlowski, N. Wink, “Shocks and quark-meson scatterings at large density”, Phys. Rev. D 104, 016028 (2021).
https://journals.aps.org/prd/abstract/10.1103/PhysRevD.104.016028
Related to Project A02, C01
Abstract:
The solution of the Dirac equation in the presence of an arbitrary plane wave, corresponding to the so-called Volkov states, has provided an enormous insight in strong-field QED. In [Phys. Rev. A \textbf{103}, 076011 (2021)] a new “fully quasiclassical” representation of the Volkov states has been found, which is equivalent to the one known in the literature but which more transparently shows the quasiclassical nature of the quantum dynamics of an electron in a plane-wave field. Here, we derive the corresponding expression of the propagator by constructing it using the fully quasiclassical form of the Volkov states. The found expression allows one, together with the fully quasiclassical expression of the Volkov states, to compute probabilities in strong-field QED in an intense plane wave by manipulating only 2-by-2 rather than 4-by-4 Dirac matrices as in the usual approach. Moreover, apart from the exponential functions featuring the classical action of an electron in a plane wave, the fully quasiclassical Volkov propagator only depends on the electron kinetic four-momentum in the plane wave, which is a gauge-invariant quantity. Finally, we also compute the tadpole diagram in a plane wave starting from the Volkov propagator and we show that, although it is divergent, its contribution can be always absorbed via a renormalization of the external field.
K. F. Muzakka, P. Duwentäster, T. J. Hobbs, T. Ježo, M. Klasen, K. Kovařík, A. Kusina, J. G. Morfín, F. I. Olness, R. Ruiz, I. Schienbein, J. Y. Yu, “Impact of W and Z Production Data and Compatibility of Neutrino DIS Data in Nuclear Parton Distribution Functions”, arXiv:2107.13235 (2021).
https://arxiv.org/abs/2107.13235
Related to Project C05
Abstract:
The coupling of the motion of two ion species in separate Penning traps via a common tank circuit is discussed. The enhancement of the coupling assisted by the tank circuit is demonstrated by an avoided crossing behavior measurement of the motional modes of two coupled ions. An intermittent laser cooling method for sympathetic cooling is proposed and a theoretical description is provided. The technique enables tuning of the coupling strength between two ion species in separate traps and thus allows for efficient sympathetic cooling of an arbitrary type of single ion for high-precision Penning-trap experiments.
A. Weigel, R. Wolf, S. Sturm, “Tank-Circuit Assisted Coupling Method for Sympathetic Laser Cooling”, Adv. Quantum Technol. 4, (2021).
https://onlinelibrary.wiley.com/doi/full/10.1002/qute.202100029
Related to Project B01
Abstract:
Volkov states are exact solutions of the Dirac equation in the presence of an arbitrary plane wave. Accounting for the interaction between the Dirac field and the electromagnetic field, however, Volkov states, as well as free photon states, are not stable in the presence of the background plane-wave field but “decay” as electrons/positrons can emit photons and photons can transform into electron-positron pairs. By using the solutions of the corresponding Schwinger-Dyson equations within the locally constant field approximation, we compute the probabilities of nonlinear single Compton scattering and nonlinear Breit-Wheeler pair production by including the effects of the decay of electron, positron, and photon states. As a result, we find that the probabilities of these processes can be expressed as the integral over the light-cone time of the known probabilities valid for stable states per unit of light-cone time times a light-cone time-dependent exponential damping function for each interacting particle. The exponential function for an incoming (outgoing) either electron/positron or photon at each light-cone time corresponds to the total probability that either the electron/positron emits a photon via nonlinear Compton scattering or the photon transforms into an electron-positron pair via nonlinear Breit-Wheeler pair production until that light-cone time (from that light-cone time on). It is interesting that the exponential damping terms depend not only on the particles momentum but also on their spin (for electrons/positrons) and polarization (for photons). This additional dependence on the discrete quantum numbers prevents the application of the standard electron/positron spin and photon polarization sum rules in computing, for example, total probabilities.
T. Podszus and A. Di Piazza, “First-order strong-field QED processes including the damping of particles states”, Phys. Rev. D 104, 016014 (2021)
https://journals.aps.org/prd/abstract/10.1103/PhysRevD.104.016014
Related to Project B02
Abstract:
Entropic uncertainty is a well-known concept to formulate uncertainty relations for continuous variable quantum systems with finitely many degrees of freedom. Typically, the bounds of such relations scale with the number of oscillator modes, preventing a straight-forward generalization to quantum field theories. In this work, we overcome this difficulty by introducing the notion of a functional relative entropy and show that it has a meaningful field theory limit. We present the first entropic uncertainty relation for a scalar quantum field theory and exemplify its behavior by considering few particle excitations and the thermal state. Also, we show that the relation implies the Robertson-Schrödinger uncertainty relation.
S. Floerchinger, T. Haas, M. Schröfl, “Relative entropic uncertainty relation for scalar quantum fields”, July 16, 2021, arXiv:2107.07824 (2021).
https://arxiv.org/abs/2107.07824
Related to Project A06
Abstract:
We show that an event-shape engineering based on the mean transverse momentum of charged hadrons, [pt], provides an optimal handle on the strength of the magnetic field created in central heavy-ion collisions at high energy. This is established through quantitative evaluations of the correlation existing between the event-by-event magnetic field produced by the spectator protons in 5.02 TeV Pb+Pb collisions and the event-by-event [pt] at a given collision centrality. We argue that the event selection based on [pt] provides a better handle on the magnetic field than the more traditional selection based on the event ellipticities. Advantages brought by this new method for the experimental search of the chiral magnetic effect are discussed.
G. Giacalone, C. Shen, “Manipulating strong electromagnetic fields with the average transverse momentum of relativistic nuclear collisions”, Eur. Phys. J. A 57, 230 (2021).
https://link.springer.com/article/10.1140%2Fepja%2Fs10050-021-00545-1
Related to Project C06
Abstract:
We compute the proper real-time interaction potential between a static quark and antiquark in classical lattice gauge theory at finite temperature. Our central result is the determination of the screened real-part of this potential, and we reconfirm the presence of an imaginary part. The real part is intimately related to the back-reaction of the static sources onto the gauge fields, incorporated via Gauss’s law. Differences in the treatment of static sources in quantum and classical lattice gauge theory are discussed.
A. Lehmann, A. Rothkopf, “Proper static potential in classical lattice gauge theory at finite T”, JHEP 07, 067 (2021).
https://link.springer.com/article/10.1007%2FJHEP07%282021%29067
Related to Project C05
Abstract:
In relativistic nuclear collisions the production of hadrons with light (u,d,s) quarks is quantitatively described in the framework of the Statistical Hadronization Model (SHM). Charm quarks are dominantly produced in initial hard collisions but interact strongly in the hot fireball and thermalize. Therefore charmed hadrons can be incorporated into the SHM by treating charm quarks as ‘impurities’ with thermal distributions, while the total charm content of the fireball is fixed by the measured open charm cross section. We call this model SHMc and demonstrate that with SHMc the measured multiplicities of single charm hadrons in lead-lead collisions at LHC energies can be well described with the same thermal parameters as for (u,d,s) hadrons. Furthermore, transverse momentum distributions are computed in a blast-wave model, which includes the resonance decay kinematics. SHMc is extended to lighter collision systems down to oxygen-oxygen and includes doubly- and triply-charmed hadrons. We show predictions for production probabilities of such states exhibiting a characteristic and quite spectacular enhancement hierarchy.
A. Andronic, P. Braun-Munzinger, M. K. Köhler, A. Mazeliauskas, K. Redlich, J. Stachel, V. Vislavicius, “The multiple-charm hierarchy in the statistical hadronization model”, JHEP 07, 035 (2021).
https://link.springer.com/article/10.1007%2FJHEP07%282021%29035
Related to Project A01, C05
Abstract:
Wehrl entropy is an entropy associated with the Husimi quasiprobability distribution. We discuss how it can be used to formulate entropic uncertainty relations and for a quantification of entanglement in continuous variables. We show that the Wehrl-Lieb inequality is closer to equality than the usual Białynicki-Birula–Mycielski entropic uncertainty relation almost everywhere. Furthermore, we show how Wehrl mutual information can be used to obtain a measurable perfect witness for pure state bipartite entanglement, which additionally provides a lower bound on the entanglement entropy.
S. Floerchinger, T. Haas, H. Müller-Groeling, “Wehrl entropy, entropic uncertainty relations, and entanglement”, Phys. Rev. A 103, 062222 (2021).
https://journals.aps.org/pra/abstract/10.1103/PhysRevA.103.062222
Related to Project A06
Abstract:
We experimentally investigate the nonlinear transmission spectrum of coherent light fields propagating through a Rydberg EIT medium with strong atomic interactions. In contrast to previous investigations, which have largely focused on resonant control fields, we explore here the full two-dimensional spectral response of the Rydberg gas. Our measurements confirm previously observed spectral features for a vanishing control-field detuning, but also reveal significant differences on two-photon resonance. In particular, we find qualitative deficiencies of mean-field models and rate-equation simulations as well as a third-order nonlinear susceptibility that accounts for pairwise interaction effects at low probe-field intensities in describing the nonlinear probe-field response under EIT conditions. Our results suggest that a more complete understanding of Rydberg EIT and emerging photon interactions requires to go beyond existing simplified models as well as few-photon theories.
A. Tebben, C. Hainaut, A. Salzinger, S. Geier, T. Franz, T. Pohl, M. Gärttner, G. Zürn, M. Weidemüller, “Nonlinear absorption in interacting Rydberg electromagnetically-inducedtransparency spectra on two-photon resonance”, Phys. Rev. A 103, 063710 (2021).
https://journals.aps.org/pra/abstract/10.1103/PhysRevA.103.063710
Related to Project A05
Abstract:
We use the nCTEQ analysis framework to investigate nuclear Parton Distribution Functions (nPDFs) in the region of large x and intermediate-to-low Q, with special attention to recent JLab Deep Inelastic Scattering data on nuclear targets. This data lies in a region which is often excluded by W and Q cuts in global nPDF analyses. As we relax these cuts, we enter a new kinematic region, which requires new phenomenology. In particular, we study the impact of i) target mass corrections, ii) higher twist corrections, iii) deuteron corrections, and iv) the shape of the nuclear PDF parametrization at large-x close to one. Using the above tools, we produce a new nPDF set (named nCTEQ15HIX) which yields a good description of the new JLab data in this challenging kinematic region, and displays reduced uncertainties at large x, in particular for up and down quark flavors.
E.P. Segarra, T. Ježo, A. Accardi, P. Duwentäster, O. Hen, T.J. Hobbs, C. Keppel, M. Klasen, K. Kovarík, A. Kusina, J.G. Morfín, K.F. Muzakka, F.I. Olness, I. Schienbein, and J.Y. Yu, “Extending nuclear PDF analyses into the high-x, low-Q2 region”, Phys. Rev. D 103, 114015 (2021).
https://journals.aps.org/prd/abstract/10.1103/PhysRevD.103.114015
Related to Project C05
SciArt Residency 2021 – Philip Kanwischer
Quantum physics meets analogue photography – Philip Kanwischer invited for two-month Artist Residency in Heidelberg
How can analogue photography and innovative research in quantum physics fit together? A groundbreaking collaboration in science communication between the international artist
collective Kalamari Klub and the Collaborative Research Centre IsoQuant at the University of Heidelberg will seek to find commonalities between these seemingly disparate fields. In the
fall of 2021, the artist Philip Kanwischer is invited for a two-month SciArt Residency in Heidelberg, where he will have the opportunity to produce a body of work in collaboration
with scientists from IsoQuant.
Philip Kanwischer, born 1991 in Bamberg, Germany, studied photography at the Academy of Fine Arts in Leipzig, Germany and at the Accademia di Belle Arti in Napoli, Italy. In his work
he combines images and text into narrative installations, studying the perception of catastrophic events and the tense relation between human and nature. His works have been
included in numerous solo and group shows. He lives and works in Leipzig, Germany. More than 170 artists from 41 countries answered the Open Call for the SciArt Residency. In
a multi-level application process, Philip Kanwischer convinced the jury consisting of experts from physics, arts, and science communication.
“The quality and number of international applications we have received is truly outstanding, and we are delighted to have been able to invite Philip Kanwischer for this project”, says
Prof. Dr. Jürgen Berges, spokesperson of the Collaborative Research Centre IsoQuant. “This underlines the high value that lies within the collaboration between art and science, and
Heidelberg as a location offers ideal conditions for a project like this.”
Members of the jury included Prof. Dr. Jürgen Berges (Spokesperson & Board member, IsoQuant), Prof. Dr. Markus Oberthaler (Board member, IsoQuant), Tina Kuka (Coordinator &
Board member, IsoQuant), Eleonora Lippi (Researcher & Board member, IsoQuant), Jasmin Meinold (Curator, Art historian), Max P. Martin (Creative Director, Kalamari Klub), Nicolas
Reinhart (Artistic Director, Kalamari Klub,) and Dennis Schulz (Science Communication, Kalamari Klub and IsoQuant).
There are more events to come within the context of the SciArt Residency: among others, an artist talk and exhibition with Philip Kanwischer are scheduled for 2021 and 2022. In addition,
the Collaborative Research Centre and Kalamari Klub are planning on publishing a magazine for photography, hosting collaborative workshops, and organizing other events,
such as photo walks.
Kalamari Klub is an international artist collective for the celebration of analogue photography. The collective runs a community darkroom in Heidelberg and has been
promoting and supporting local and international artists through exhibitions, workshops, publications and residency programmes since 2016.
IsoQuant is a Collaborative Research Centre (CRC) at the University of Heidelberg focusing on Isolated Quantum Systems and Universality in Extreme Conditions. Collaborative
Research Centres are long-term research institutions in which researchers are tackling innovative, challenging and complex research questions within a cross- disciplinary research
program. The scientific aim of the CRC IsoQuant is to explore and gain better understanding of the question why many physical quantum systems exhibit characteristic common
properties despite fundamental differences in key parameters such as temperature, density, or field strength. The cross-disciplinary approach to this research question within the
IsoQuant collaboration brings together theoretical and experimental physicists from different fields and allows for a broad bandwidth of experimental and theoretical methods to solve
overarching issues in physics.
Further Information
https://www.kalamariklub.org
https://www.isoquant-heidelberg.de
https://philipkanwischer.de
Contact
Tina Kuka & Dennis Schulz, Wissenschaftskommunikation IsoQuant
scicom-isoquant@thphys.uni-heidelberg.de
Nicolas Reinhart, Künstlerische Leitung Kalamari Klub
nico@kalamariklub.org
SciArt Residency 2021 – Philip Kanwischer
Quantenphysik trifft analoge Fotografie – Philip Kanwischer für zweimonatige Artist Residency nach Heidelberg eingeladen
Wie können analoge Fotografie und innovative Forschung im Bereich der Quantenphysik zusammenpassen? Diese spannende Frage stellt sich das internationale Künstler*innen-Kollektiv Kalamari Klub gemeinsam mit Wissenschaftler*innen des Sonderforschungsbereichs IsoQuant an der Universität Heidelberg. Eingebettet in die Strategie für kreative Ansätze zur Wissenschaftskommunikation entstand erstmalig die Idee für eine Kooperation von Kunst und Wissenschaft. Im Herbst 2021 wird im Rahmen dieser Kooperation der Künstler Philip Kanwischer für eine zweimonatige Künstler*innenresidenz nach Heidelberg eingeladen, um eine künstlerische Arbeit in Zusammenarbeit mit den Wissenschaftler*innen des Sonderforschungsbereiches IsoQuant zu entwickeln.
Philip Kanwischer, geboren 1991 in Bamberg, studierte Fotografie an der Hochschule für Grafik und Buchkunst in Leipzig und an der Accademia di Belle Arti di Napoli, Italien. In seinen Arbeiten verknüpft Kanwischer Bild und Textmaterial zu narrativen Raumsituationen und thematisiert dabei u.a. die Wahrnehmung von Katastrophen und das Spannungsverhältnis zwischen Mensch und Natur. Seine Werke wurden in zahlreichen Einzel- und Gruppenausstellungen gezeigt, er lebt und arbeitet in Leipzig. Auf den Open Call der ausgeschriebenen SciArt Residency bewarben sich mehr als 170 Künstler*innen aus 41 Ländern. In einem mehrstufigen Auswahlverfahren überzeugte Philip Kanwischer die Jury, bestehend aus Expert*innen der Physik, der Kunst und der Wissenschaftskommunikation.
„Die Qualität und Anzahl der internationalen Bewerbungen, die wir erhalten haben, ist wirklich herausragend und wir freuen uns sehr, dass wir Philip Kanwischer für dieses Projekt gewinnen konnten“, sagt Prof. Dr. Jürgen Berges, Sprecher des Sonderforschungsbereichs IsoQuant. „Das unterstreicht den hohen Stellenwert der Kollaboration von Kunst und Wissenschaft am Standort Heidelberg, der ideale Voraussetzungen bietet.“
Mitglieder der Jury waren Prof. Dr. Jürgen Berges (Sprecher & Vorstandsmitglied, IsoQuant), Prof. Dr. Markus Oberthaler (Vorstandsmitglied, IsoQuant), Tina Kuka (Koordinatorin & Vorstandsmitglied, IsoQuant), Eleonora Lippi (Wissenschaftlerin & Vorstandsmitglied, IsoQuant), Jasmin Meinold (Kuratorin und Kunstwissenschaftlerin), Max P. Martin (Kreative Leitung, Kalamari Klub), Nicolas Reinhart (Künstlerische Leitung, Kalamari Klub) und Dennis Schulz (Wissenschaftskommunikation, Kalamari Klub und IsoQuant).
Im Rahmen der SciArt Resideny wird es weitere Veranstaltungen mit Philip Kanwischer geben, u.a. sind ein Artist Talk und eine Ausstellung der künstlerischen Arbeiten für 2021 und 2022 geplant. Darüber hinaus plant der Sonderforschungsbereich IsoQuant mit Kalamari Klub die Veröffentlichung eines Magazins für Fotografie, gemeinsame Workshops und weitere Aktionen wie z.B. Photo Walks.
Kalamari Klub ist ein internationales Künstler*innen-Kollektiv für analoge Fotografie. Das Kollektiv betreibt eine öffentliche Dunkelkammer in Heidelberg und unterstützt seit 2016 lokal und international Künstler*innen durch Ausstellungen, Workshops, Publikationen und Artist Residencies.
IsoQuant ist ein Sonderforschungsbereich an der Universität Heidelberg zum Thema „Isolierte Quantensysteme und Universalität unter extremen Bedingungen“. Sonderforschungsbereiche sind langfristige Projekte zur Grundlagenforschung, in denen Wissenschaftler einer oder mehrerer Universitäten interdisziplinär an komplexen neuen Fragestellungen zusammenarbeiten. Im Mittelpunkt des SFB 1225 IsoQuant steht ein Forschungsthema, das für eine Vielzahl von Anwendungen in der Physik von großer Bedeutung ist. Viele Quantensysteme weisen trotz grundlegender Unterschiede bei Schlüsselparametern wie Temperatur, Dichte oder Feldstärke charakteristische gemeinsame
Eigenschaften auf. Diese universellen Eigenschaften besser zu verstehen, erfordert übergreifende Herangehensweisen in der Forschung über traditionelle Spezialisierungen hinweg. Die Zusammenarbeit von ca. 20 Arbeitsgruppen aus theoretischer und experimenteller Physik innerhalb des SFB1225 IsoQuant erlaubt die Verwendung einer großen Bandbreite an Methoden zur Lösung übergreifender Fragestellungen in der Physik.
Weitere Informationen
https://www.kalamariklub.org
https://www.isoquant-heidelberg.de
https://philipkanwischer.de
Kontakt
Tina Kuka & Dennis Schulz, Wissenschaftskommunikation IsoQuant
scicom-isoquant@thphys.uni-heidelberg.de
Nicolas Reinhart, Künstlerische Leitung Kalamari Klub
nico@kalamariklub.org
Quantum physics meets analogue photography – Philip Kanwischer invited for two-month Artist Residency in Heidelberg
How can analogue photography and innovative research in quantum physics fit together? A groundbreaking collaboration in science communication between the international artist
collective Kalamari Klub and the Collaborative Research Centre IsoQuant at the University of Heidelberg will seek to find commonalities between these seemingly disparate fields. In the
fall of 2021, the artist Philip Kanwischer is invited for a two-month SciArt Residency in Heidelberg, where he will have the opportunity to produce a body of work in collaboration
with scientists from IsoQuant.
Philip Kanwischer, born 1991 in Bamberg, Germany, studied photography at the Academy of Fine Arts in Leipzig, Germany and at the Accademia di Belle Arti in Napoli, Italy. In his work
he combines images and text into narrative installations, studying the perception of catastrophic events and the tense relation between human and nature. His works have been
included in numerous solo and group shows. He lives and works in Leipzig, Germany. More than 170 artists from 41 countries answered the Open Call for the SciArt Residency. In
a multi-level application process, Philip Kanwischer convinced the jury consisting of experts from physics, arts, and science communication.
“The quality and number of international applications we have received is truly outstanding, and we are delighted to have been able to invite Philip Kanwischer for this project”, says
Prof. Dr. Jürgen Berges, spokesperson of the Collaborative Research Centre IsoQuant. “This underlines the high value that lies within the collaboration between art and science, and
Heidelberg as a location offers ideal conditions for a project like this.”
Members of the jury included Prof. Dr. Jürgen Berges (Spokesperson & Board member, IsoQuant), Prof. Dr. Markus Oberthaler (Board member, IsoQuant), Tina Kuka (Coordinator &
Board member, IsoQuant), Eleonora Lippi (Researcher & Board member, IsoQuant), Jasmin Meinold (Curator, Art historian), Max P. Martin (Creative Director, Kalamari Klub), Nicolas
Reinhart (Artistic Director, Kalamari Klub,) and Dennis Schulz (Science Communication, Kalamari Klub and IsoQuant).
There are more events to come within the context of the SciArt Residency: among others, an artist talk and exhibition with Philip Kanwischer are scheduled for 2021 and 2022. In addition,
the Collaborative Research Centre and Kalamari Klub are planning on publishing a magazine for photography, hosting collaborative workshops, and organizing other events,
such as photo walks.
Kalamari Klub is an international artist collective for the celebration of analogue photography. The collective runs a community darkroom in Heidelberg and has been
promoting and supporting local and international artists through exhibitions, workshops, publications and residency programmes since 2016.
IsoQuant is a Collaborative Research Centre (CRC) at the University of Heidelberg focusing on Isolated Quantum Systems and Universality in Extreme Conditions. Collaborative
Research Centres are long-term research institutions in which researchers are tackling innovative, challenging and complex research questions within a cross- disciplinary research
program. The scientific aim of the CRC IsoQuant is to explore and gain better understanding of the question why many physical quantum systems exhibit characteristic common
properties despite fundamental differences in key parameters such as temperature, density, or field strength. The cross-disciplinary approach to this research question within the
IsoQuant collaboration brings together theoretical and experimental physicists from different fields and allows for a broad bandwidth of experimental and theoretical methods to solve
overarching issues in physics.
Further Information
https://www.kalamariklub.org
https://www.isoquant-heidelberg.de
https://philipkanwischer.de
Contact
Tina Kuka & Dennis Schulz, Wissenschaftskommunikation IsoQuant
scicom-isoquant@thphys.uni-heidelberg.de
Nicolas Reinhart, Künstlerische Leitung Kalamari Klub
nico@kalamariklub.org
Abstract:
We present a detailed analysis of the relaxation dynamics in an extended bosonic Josephson junction. We show that stochastic classical field simulations in three spatial dimensions reproduce the main experimental findings of M. Pigneur et al., Phys. Rev. Lett. 120, 173601 (2018). We give an analytic solution describing the short time evolution through multimode dephasing. For longer times, the observed relaxation to a phase locked state is caused by nonlinear dynamics beyond the sine-Gordon model, persisting even at zero temperature. Finally, we analyze different experimentally relevant trapping geometries and their potential for analogue simulation of the sine-Gordon model out of equilibrium. Our results provide the basis for future experimental implementations aiming to study quantum effects of extended bosonic Josephson junctions.
J.-F. Mennemann, I. E. Mazets, M. Pigneur, H. P. Stimming, N. J. Mauser, J. Schmiedmayer, S. Erne, “Relaxation in an Extended Bosonic Josephson Junction”, Phys. Rev. Research 3, 023197 (2021).
https://journals.aps.org/prresearch/abstract/10.1103/PhysRevResearch.3.023197
Related to Project A03
Abstract:
Quantum uncertainty relations are formulated in terms of relative entropy between distributions of measurement outcomes and suitable reference distributions with maximum entropy. This type of entropic uncertainty relation can be applied directly to observables with either discrete or continuous spectra. We find that a sum of relative entropies is bounded from above in a nontrivial way, which we illustrate with some examples.
S. Floerchinger, T. Haas, B. Hoeber, “Relative entropic uncertainty relation”, Phys. Rev. A 103, 062209 (2021).
https://journals.aps.org/pra/abstract/10.1103/PhysRevA.103.062209
Related to Project A06
We invite all female students to join our next Thesis@IsoQuant Meeting. Due to the current COVID-19 meeting restrictions, the meeting will be held online via Zoom. Prior registration is needed at https://uebungen.physik.uni-heidelberg.de/uebungen/liste.php?vorl=1329
Motivation
Our Thesis@Isoquant-Meetings are a networking and mentoring platform for female students in physics addressing 3 main objectives:
(1) getting to know the working groups within the Collaborative Research Centre 1225 IsoQuant
(2) find possible topics and supervisors for bachelor, master or PhD thesis
(3) discuss & exchange about general topics related to university / career & family planning / work-life balance/ etc.
Next meeting: Wednesday, 09 June 2021, 17:15 -18:45
Invited guest: Dr. Kira Rehfeld (Institute for Environmental Physics) and her working group
Zoom-Link:https://cern.zoom.us/j/61148677066?pwd=NjAvM3phdksvUnNPaHh1aW42OXFEUT09
Meeting ID: 611 4867 7066
Passcode: 436979
We look forward to seeing many of you there!
If you have any questions, do not hesitate to contact us via sfb1225@thphys.uni-heidelberg.de.
We invite all female students to join our next Thesis@IsoQuant Meeting. Due to the current COVID-19 meeting restrictions, the meeting will be held online via Zoom. Prior registration is needed at https://uebungen.physik.uni-heidelberg.de/uebungen/liste.php?vorl=1329
Motivation
Our Thesis@Isoquant-Meetings are a networking and mentoring platform for female students in physics addressing 3 main objectives:
(1) getting to know the working groups within the Collaborative Research Centre 1225 IsoQuant
(2) find possible topics and supervisors for bachelor, master or PhD thesis
(3) discuss & exchange about general topics related to university / career & family planning / work-life balance/ etc.
Next meeting: Wednesday, May 19
Invited CRC1225 member: Prof. Dr. Selim Jochim (PI) and his woking group
16:00-17:30
Zoom-Link: https://zoom.us/j/99114904473?pwd=OWZKd0lmVUVCbkdtbVAwOVRKaXdrdz09
Meeting-ID: 991 1490 4473
Kenncode: 524216
We look forward to seeing many of you there!
If you have any questions, do not hesitate to contact us via sfb1225@thphys.uni-heidelberg.de.
Abstract:
We study anomalous chiral symmetry breaking in two-flavor QCD induced by gravitational and QCD-instantons within asymptotically safe gravity within the functional renormalization group approach. Similarly to QCD-instantons, gravitational ones, associated to a K3-surface connected by a wormhole-like throat in flat spacetime, generate contributions to the ’t Hooft coupling proportional to exp(−1/gN) with the dimensionless Newton coupling gN. Hence, in the asymptotically safe gravity scenario with a nonvanishing fixed point coupling g∗N, the induced ’t Hooft coupling is finite at the Planck scale, and its size depends on the chosen UV-completion. Within this scenario the gravitational effects on anomalous U(1)A-breaking at the Planck scale may survive at low energy scales. In turn, fermion masses of the order of the Planck scale cannot be present. This constrains the allowed asymptotically safe UV-completion of the gravity-QCD system. We map out the parameter regime that is compatible with the existence of light fermions in the low-energy regime.
Y. Hamada, J. M. Pawlowski , M. Yamada, “Gravitational instantons and anomalous chiral symmetry breaking”, Phys. Rev. D 103, 106016 (2021).
https://journals.aps.org/prd/abstract/10.1103/PhysRevD.103.106016
Related to Project: A02, B03
Abstract:
Entanglement is not only the resource that fuels many quantum technologies but also plays a key role for some of the most profound open questions of fundamental physics. Experiments controlling quantum systems at the single quantum level may shed light on these puzzles. However, measuring, or even bounding, entanglement experimentally has proven to be an outstanding challenge, especially when the prepared quantum states are mixed. We use entropic uncertainty relations for bipartite systems to derive measurable lower bounds on distillable entanglement. We showcase these bounds by applying them to physical models realizable in cold-atom experiments. The derived entanglement bounds rely on measurements in only two different bases and are generically applicable to any quantum simulation platform.
B. Bergh and M. Gärttner, “Experimentally Accessible Bounds on Distillable Entanglement from Entropic Uncertainty Relations”, Phys. Rev. Lett. 126, 190503 (2021).
https://journals.aps.org/prl/abstract/10.1103/PhysRevLett.126.190503
Related to Project A06
Abstract:
We study experimentally accessible lower bounds on entanglement measures based on entropic uncertainty relations. Experimentally quantifying entanglement is highly desired for applications of quantum simulation experiments to fundamental questions, e.g., in quantum statistical mechanics and condensed-matter physics. At the same time it poses a significant challenge because the evaluation of entanglement measures typically requires the full reconstruction of the quantum state, which is extremely costly in terms of measurement statistics. We derive an improved entanglement bound for bipartite systems, which requires measuring joint probability distributions in only two different measurement settings per subsystem, and demonstrate its power by applying it to currently operational experimental setups for quantum simulation with cold atoms. Examining the tightness of the derived entanglement bound, we find that the set of pure states for which our relation is tight is strongly restricted. We show that, for measurements in mutually unbiased bases, the only pure states that saturate the bound are maximally entangled states on a subspace of the bipartite Hilbert space (this includes product states). We further show that our relation can also be employed for entanglement detection using generalized measurements, i.e., when not all measurement outcomes can be resolved individually by the detector. In addition, the impact of local conserved quantities on the detectable entanglement is discussed.
B. Bergh and M. Gärttner, “Entanglement detection in quantum many-body systems using entropic uncertainty relations”, Phys. Rev. A 103, 052412 (2021).
https://journals.aps.org/pra/abstract/10.1103/PhysRevA.103.052412
Related to Project A06
Abstract:
The renormalization group plays an essential role in many areas of physics, both conceptually and as a practical tool to determine the long-distance low-energy properties of many systems on the one hand and on the other hand search for viable ultraviolet completions in fundamental physics. It provides us with a natural framework to study theoretical models where degrees of freedom are correlated over long distances and that may exhibit very distinct behavior on different energy scales. The nonperturbative functional renormalization-group (FRG) approach is a modern implementation of Wilson’s RG, which allows one to set up nonperturbative approximation schemes that go beyond the standard perturbative RG approaches. The FRG is based on an exact functional flow equation of a coarse-grained effective action (or Gibbs free energy in the language of statistical mechanics). We review the main approximation schemes that are commonly used to solve this flow equation and discuss applications in equilibrium and out-of-equilibrium statistical physics, quantum many-particle systems, high-energy physics and quantum gravity.
N. Dupuis, L. Canet, A. Eichhorn, W. Metzner, J. M. Pawlowski, M. Tissier, N. Wschebor, “The nonperturbative functional renormalization group and its applications”, Phys. Rept. 910, 1 (2021).
https://www.sciencedirect.com/science/article/abs/pii/S0370157321000156?via%3Dihub
Related to Project
Abstract:
We discuss the coupling of the motion of two ion species in separate Penning traps via a common tank circuit. The enhancement of the coupling assisted by the tank circuit is demonstrated by an avoided crossing behavior measurement of the motional modes of two coupled ions. We propose an intermittent laser cooling method for sympathetic cooling and provide a theoretical description. The technique enables tuning of the coupling strength between two ion species in separate traps and thus allows for efficient sympathetic cooling of an arbitrary type of single ion for high-precision Penning-trap experiments.
B. Tu, F. Hahne, I. Arapoglou, A. Egl, F. Heiße, M. Höcker, C. König, J. Morgner, T. Sailer, A. Weigel, R. Wolf, S. Sturm, “Tank-Circuit Assisted Coupling Method for Sympathetic Laser Cooling”, Adv. Quantum Technol. (2021)
https://onlinelibrary.wiley.com/doi/10.1002/qute.202100029
Related to Project B01
Abstract:
We calculate the gravitational-wave spectra produced by the electroweak phase transition with TeV-scale Beyond-Standard-Model physics in the early universe. Our study captures the effect of quantum and thermal fluctuations within a non-perturbative framework. We discover a universal relation between the mean bubble separation and the strength parameter of the phase transition, which holds for a wide range of new-physics contributions. The ramifications of this result are three-fold: first, they constrain the gravitational-wave spectra resulting from heavy (TeV-scale) new physics. Second, they contribute to distinguishing heavy from light new physics directly from the gravitational-wave signature. Third, they suggest that a concerted effort of gravitational-wave observations together with collider experiments could be required to distinguish between different models of heavy new physics.
A. Eichhorn, J. Lumma, J. M. Pawlowski, M. Reichert, M. Yamada, “Universal gravitationalwave signatures from heavy new physics in the electroweak sector”, JCAP 05, 006 (2021).
https://iopscience.iop.org/article/10.1088/1475-7516/2021/05/006
Related to Project: A02
Abstract:
The interaction between medium-weight nuclei and a strong zeptosecond laser pulse of MeV photons is investigated theoretically. Multiple absorption of photons competes with nuclear equilibration. We investigate the sudden regime. Here the rate of photon absorption is so strong that there is no time for the nucleus to fully equilibrate after each photon absorption process. We follow the temporal evolution of the system in terms of a set of rate equations. These account for dipole absorption and induced dipole emission, equilibration (modeled in terms of particle-hole states coupled by the residual nuclear interaction), and neutron decay (populating a chain of proton-rich nuclei). Our results are compared with earlier work addressing the adiabatic regime where equilibration is instantaneous. We predict the degree of excitation and the range of nuclei reached by neutron evaporation. These findings are relevant for planning future experiments.
S. Kobzak, H. A. Weidenmüller, A. Pàlffy, “Laser-nucleus interactions in the sudden regime”, Phys. Rev. C 103, 044616 (2021).
https://journals.aps.org/prc/abstract/10.1103/PhysRevC.103.044616
Related to Project: B02
We consider the non-equilibrium dynamics of a weakly interacting Bose gas tightly confined to a highly elongated double well potential. We use a self-consistent time-dependent Hartree–Fock approximation in combination with a projection of the full three-dimensional theory to several coupled one-dimensional channels. This allows us to model the time-dependent splitting and phase imprinting of a gas initially confined to a single quasi one-dimensional potential well and obtain a microscopic description of the ensuing damped Josephson oscillations.
Y. D. van Nieuwkerk, J. Schmiedmayer and F. H.L. Essler, “Josephson oscillations in split
one-dimensional Bose gases”, SciPost Phys. 10, 090 (2021).
https://scipost.org/10.21468/SciPostPhys.10.4.090
Related to Project A03
Abstract:
Promising searches for new physics beyond the current standard model (SM) of particle physics are feasible through isotope-shift spectroscopy, which is sensitive to a hypothetical fifth force between the neutrons of the nucleus and the electrons of the shell. Such an interaction would be mediated by a new particle which could in principle be associated with dark matter. In so-called King plots, the mass-scaled frequency shifts of two optical transitions are plotted against each other for a series of isotopes. Subtle deviations from the expected linearity could reveal such a fifth force. Here, we study experimentally and theoretically six transitions in highly charged ions of Ca, an element with five stable isotopes of zero nuclear spin. Some of the transitions are suitable for upcoming high-precision coherent laser spectroscopy and optical clocks. Our results provide a sufficient number of clock transitions for—in combination with those of singly charged Ca+—application of the generalized King plot method. This will allow future high-precision measurements to remove higher-order SM-related nonlinearities and open a door to yet more sensitive searches for unknown forces and particles.
N.-H. Rehbehn, M. K. Rosner, H. Bekker, J. C. Berengut, P. O. Schmidt, S. A. King, P. Micke, M. F. Gu, R. Müller, A. Surzhykov, and J. R. Crespo López-Urrutia, “Sensitivity to new physics of isotope-shift studies using the coronal lines of highly charged calcium ions”, Phys. Rev. A 103, L040801 (2021).
https://journals.aps.org/pra/abstract/10.1103/PhysRevA.103.L040801
Related to Project B01
Abstract:
We investigate the ground state properties of one-dimensional hard-core bosons interacting via a variable long-range potential using the density matrix renormalization group. We show that restoring energy extensivity in the system, which is done by rescaling the interaction potential with a suitable size-dependent factor known as Kac’s prescription, has a profound influence on the low-energy properties in the thermodynamic limit. While an insulating phase is found in the absence of Kac’s rescaling, the latter leads to a new metallic phase that does not fall into the conventional Luttinger liquid paradigm. We discuss a scheme for the observation of this new phase using cavity-mediated long-range interactions with cold atoms. Our findings raise fundamental questions on how to study the thermodynamics of long-range interacting quantum systems.
T. Botzung, D. Hagenmüller, G. Masella, J. Dubail, N. Defenu, A. Trombettoni, and G. Pupillo, “Effects of energy extensivity on the quantum phases of long-range interacting systems”, Phys. Rev. B 103, 155139 (2021).
https://journals.aps.org/prb/abstract/10.1103/PhysRevB.103.155139
Related to Project C02
We are happy to announce that the registration for the Annual Retreat of the CRC 1225 ISOQUANT Young Researchers’ Convent is now open! Due to the current Covid-19 situation, we decided to turn the retreat into a fully online event which will take place from May 17-19, 2021.
The main intention of this event is to provide a platform for cross-project collaboration and the opportunity to intensify exchanges and networking between young researchers (bachelor, master and PhD students & young postdocs) within the IsoQuant collaboration. This year’s subject will be “Quantum Computation: Solving Problems on Quantum Hardware”.
Prof. Fred Jendrzejewski (IsoQuant), Prof. John Martinis (Silicon Quantum Computing, Sydney, formerly at Google), Dr. Juris Ulmanis (Quantum Alpine Technology, Innsbruck) and Dr. Christian Gogolin (Covestro, Germany) will join this online workshop contributing with lectures, talks and a panel discussion.
The plan is to develop small group projects focussing on current research topics ranging from gauge theories to quantum chemistry. You will get a glimpse at the current state of quantum technologies, run your own algorithm (eg. using Qiskit) on real quantum computers and hear leading experts on the future of quantum computation.
Registration is open now:
https://docs.google.com/forms/d/e/1FAIpQLSfvIB3WYXv0td4xk7rdybp9QaqAGf0oNTxfKe_PMzEd6eEMWg/viewform
Deadline for registration is April 30, 2021!
A preliminary schedule can be downloaded here:
https://heibox.uni-heidelberg.de/f/82d0e4ee178546e2a618/?dl=1
If you have any questions, please do not hesitate to contact us at sfb1225@thphys.uni-heidelberg.de.
Abstract:
We study the influence of global baryon number conservation on the non-critical baseline of net baryon cumulants in heavy-ion collisions in a given acceptance, accounting for the asymmetry between the mean-numbers of baryons and antibaryons. We derive the probability distribution of net baryon number in a restricted phase space from the canonical partition function that incorporates exact conservation of baryon number in the full system. Furthermore, we provide tools to compute cumulants of any order from the generating function of uncorrelated baryons constrained by exact baryon number conservation. The results are applied to quantify the non-critical baseline for cumulants of net proton number fluctuations obtained in heavy-ion collisions by the STAR collaboration at different RHIC energies and by the ALICE collaboration at the LHC. Furthermore, volume fluctuations are added by a Monte Carlo procedure based on the centrality dependence of charged particle production as measured experimentally. Compared to the predictions based on the hadron resonance gas model or Skellam distribution a clear suppression of fluctuations is observed due to exact baryon-number conservation. The suppression increases with the order of the cumulant and towards lower collision energies. Predictions for net proton cumulants up to the eight order in heavy-ion collisions are given for experimentally accessible collision energies.
P. Braun-Munzinger, B. Friman, K. Redlich, A. Rustamov, J. Stachel, “Relativistic nuclear collisions: Establishing the non-critical baseline for fluctuation measurements”, Nucl. Phys. A 1008 (2021).
https://www.sciencedirect.com/science/article/pii/S0375947421000063?via%3Dihub
Related to Project A01, A02, A03, A04, C05, C06
Abstract:
Available laser technology is opening the possibility of testing QED experimentally in the so-called strong-field regime. This calls for developing theoretical tools to investigate strong-field QED processes in electromagnetic fields of complex spacetime structure. Here, we propose a scheme to compute electron wave functions in tightly focused laser beams by taking into account exactly the complex spacetime structure of the fields. The scheme is solely based on the validity of the Wentzel-Kramers-Brillouin (WKB) approximation and the resulting wave functions, unlike previously proposed ones [Phys. Rev. Lett. \textbf{113}, 040402 (2014)], do not directly rely on approximations on the classical electron trajectory. Moreover, a consistent procedure is indicated to take into account higher-order quantum effects within the WKB approach depending on higher-and-higher powers of the Planck constant. In the case of a plane-wave background field we show that the found wave functions exactly reduce to the Volkov states, which are then written in a new and fully quasiclassical form. Finally, by using the leading-order WKB wave functions to compute the probabilities of nonlinear Compton scattering and nonlinear Breit-Wheeler pair production, it is explicitly shown that, if additionally the energies of the charges are sufficiently large that the latter are not significantly deflected by the field, the corresponding Baier’s formulas are exactly reproduced for an otherwise arbitrary classical electron trajectory.
A. Di Piazza, “WKB Electron Wave Functions in a Tightly Focused Laser Beam”, Phys. Rev. D 103, 076011 (2021).
https://journals.aps.org/prd/abstract/10.1103/PhysRevD.103.076011
Related to Project B02
Abstract:
Universality is one of the key concepts in understanding critical phenomena. However, for interacting inhomogeneous systems described by complex networks, a clear understanding of the relevant parameters for universality is still missing. Here we discuss the role of a fundamental network parameter for universality, the spectral dimension. For this purpose, we construct a complex network model where the probability of a bond between two nodes is proportional to a power law of the nodes’ distances. By explicit computation we prove that the spectral dimension for this model can be tuned continuously from 1 to infinity, and we discuss related network connectivity measures. We propose our model as a tool to probe universal behavior on inhomogeneous structures and comment on the possibility that the universal behavior of correlated models on such networks mimics the one of continuous field theories in fractional Euclidean dimensions.
A. P. Millán and G. Gori and F. Battiston and T. Enss and N. Defenu, “Complex networks with tuneable spectral dimension as a universality playground”, Phys. Rev. Research 3, 023015 (2021).
https://journals.aps.org/prresearch/abstract/10.1103/PhysRevResearch.3.023015
Related to Project C02, C03, A05
Abstract:
In this paper we present a study of in-medium jet modifications performed with JEWEL and PYTHIA 6.4, focusing on the uncertainties related to variations of the perturbative scales and nuclear parton distribution functions (PDFs) and on the impact of the initial and crossover temperature variations of the medium. The simulations are compared to LHC data for the jet spectrum and the nuclear modification factor. We assess the interplay between the choice of nuclear PDFs and different medium parameters and study the impact of nuclear PDFs and the medium on the jet structure via the Lund plane.
A. Andronic, J. Honermann, M. Klasen, C. Klein-Bösing, J. Salomon, “Impact of scale, nuclear PDF and temperature variations on the interpretation of medium-modified jet production data from the LHC”, High Energ. Phys. 6 (2021).
https://link.springer.com/article/10.1007/JHEP04(2021)006
Related to Project C05
Abstract:
In this article, we revisit the heteronuclear Efimov effect in a Bose-Fermi mixture with large mass difference in the Born-Oppenheimer picture. As a specific example, we consider the combination of bosonic 133Cs and fermionic 6Li. In a system consisting of two heavy bosons and one light fermion, the fermion-mediated potential between the two heavy bosons gives rise to an infinite series of three-body bound states. The intraspecies scattering length determines the three-body parameter and the scaling factor between consecutive Efimov states. In a second scenario, we replace the single fermion by an entire Fermi Sea at zero temperature. The emerging interaction potential for the two bosons exhibits long-range oscillations leading to a weakening of the binding and a breakup of the infinite series of Efimov states. In this scenario, the binding energies follow a modified Efimov scaling law incorporating the Fermi momentum. The scaling factor between deeply bound states is governed by the intraspecies interaction, analogous to the Efimov states in vacuum.
B. Tran, M. Rautenberg, M. Gerken, E. Lippi, B. Zhu, J. Ulmanis, M. Drescher, M. Salmhofer, T. Enss, M. Weidemüller, “Fermions meet two bosons—the heteronuclear Efimov effect revisited”, Braz. J. Phys. 51 (2021).
https://link.springer.com/article/10.1007%2Fs13538-020-00811-5
Related to Project: C03
Abstract:
We give a detailed account of a stationary impurity in an ideal Bose-Einstein condensate, which we call the ideal Bose polaron, at both zero and nonzero temperatures and arbitrary strength of the impurity-boson coupling. The time evolution is solved exactly and it is found that, surprisingly, many of the features that have been predicted for the real BEC are already present in this simpler setting and can be understood analytically therein. We obtain explicit formulas for the time evolution of the condensate wave function at T=0 and of the one-particle density matrix at T>0. For negative scattering length, the system is found to thermalize even though the dynamics are perfectly coherent. The time evolution and thermal values of the Tan contact are derived and compared to a recent experiment. We find that contrary to the Fermi polaron, the contact is not bounded at unitarity as long as a condensate exists. An explicit formula for the dynamical overlap at T=0 allows us to compute the rf spectrum which can be understood in detail by relating it to the two-body problem of one boson and the impurity.
M. Drescher, M. Salmhofer, T. Enss, “Quench Dynamics of the Ideal Bose Polaron at Zero and Nonzero Temperatures”, Phys. Rev. A 103, 033317 (2021).
https://journals.aps.org/pra/abstract/10.1103/PhysRevA.103.033317
Related to Project: C03, C02
We warmly welcome Jun.-Prof. Dr. Lauriane Chomaz as new principal investigator within the CRC1225 collaboration (C03).
On February 1st, Lauriane Chomaz received her nomination as Junior Professor of Heidelberg University. She is now starting to set up her working group at the Physikalisches Institut, exploring the behavior of quantum fluids using ultracold assemblies of atoms. If you want to find out more about her research work, feel free to visit her website.
The next Science Pub Quiz is coming up on Wednesday, 17 March 2021 as an online edition (via YouTube). As usually, the Science Pub Quiz starts at 7.30 pm. Our guest this time: Franziska Kohlt. She did her PhD at the University of Oxford, writing, among other topics, about the fantastical literature of the 19th century. She works as a postdoc for science communication at the University of York and a translator for Marvel Comics, published papers on the communication of the COVID-19 pandemic and the history and ethics of AI. To find out more about her, just visit her website www.franziskakohlt.com
Wanna join the quiz? Then register at www.scipubquiz.de/register! We will send you the Youtube link one day before the quiz.
For more information on the Science Pub Quiz:
Website: www.scipubquiz.de
Facebook: https://www.facebook.com/scipubquiz
Instagram: @science.quiz.hd
www.instagram.com/science.quiz.hd/
We are really looking forward to see you all again!
Abstract:
A critical set of two-loop quantum electrodynamics corrections to the g factor of hydrogenlike ions is calculated in the Furry picture. These corrections are due to the polarization of the external magnetic field by the quantum vacuum, which is dressed by the binding field. The result obtained for the self-energy–magnetic-loop diagrams is compared with the current state-of-the-art result, derived through a perturbative expansion in the binding strength parameter Zα, with Z the atomic number and α the fine-structure constant. Agreement is found in the Z→0 limit. However, even for very light ions, the perturbative result fails to approximate the magnitude of the corresponding correction to the g factor. The total correction to the g factor coming from all diagrams considered in this work is found to be highly relevant for upcoming experimental tests of fundamental physics with highly charged ions.
V. Debierre, B. Sikora, H. Cakir, N. S. Oreshkina, V. A. Yerokhin, C. H. Keitel, and Z. Harman, “Two-loop virtual light-by-light scattering corrections to the bound-electron g factor”, Phys. Rev. A 103, L030802 (2021).
https://journals.aps.org/pra/abstract/10.1103/PhysRevA.103.L030802
Related to Project B02
Abstract:
In an effort to address integrability breaking in cold gas experiments, we extend the integrable hydrodynamics of the Lieb-Liniger model with two additional components representing the population of atoms in the first and second transverse excited states, thus enabling a description of quasi-1D condensates. Collisions between different components are accounted for through the inclusion of a Boltzmann-type collision integral in the hydrodynamic equation. Contrary to standard generalized hydrodynamics, our extended model captures thermalization of the condensate at a rate consistent with experimental observations from a quantum Newton’s cradle setup.
F. Møller, C. Li, I. Mazets, H.-P. Stimming, T. Zhou, Z. Zhu, X. Chen, J. Schmiedmayer, “Extension of the Generalized Hydrodynamics to Dimensional Crossover Regime”, Phys. Rev. Lett. 126 (2021).
https://journals.aps.org/prl/abstract/10.1103/PhysRevLett.126.090602
Related to Project A03
Abstract:
In ultracold quantum gases, the interactions between the individual atoms can be controlled by applying magnetic bias fields. As magnetic field fluctuations limit the precision here, typically a feedback loop needs to be employed to regulate the current through a pair of Helmholtz coils. No commercially available magnetic field sensor allows to measure large fields directly with high enough precision, leading to many unsatisfactory solutions being used in experiments. Here, we demonstrate a direct magnetic field stabilization in a regime previously not accessible, using NV centers as the magnetic field sensor. This allows us to measure and stabilize fields of 4.66 mT down to 12 nT RMS noise over the course of 24 h, measured on a 1 Hz bandwidth. We achieve a control of better than 1 ppm after 20 min of integration time, ensuring high long-term stability for experiments. This approach extends direct magnetic field control to strong magnetic fields, which could enable new precise quantum simulations in this regime.
A. Hesse, K. Köster, J. Steiner, J. Michl, V. Vorobyov, D. Dasari, J. Wrachtrup, F. Jendrzejewski, “Direct control of high magnetic fields for cold atom experiments based on NV centers”, New J. Phys. 23, 023037 (2021).
https://iopscience.iop.org/article/10.1088/1367-2630/abe1e5
Related to Project B04
Pressemitteilung MPIK, 17. Februar 2021
Erstmals ist einem Forscherteam des Heidelberger Max-Planck-Instituts für Kernphysik die kohärente Kontrolle von Kernanregungen mit geeignet geformten Röntgenlicht gelungen. In dem am Europäischen Synchrotron ESRF durchgeführten Experiment wurde eine zeitliche Stabilität von wenigen Zeptosekunden erreicht. Zukünftige Experimente basierend auf der Kontrolle der Kerndynamik versprechen genauere Zeitstandards und neue Möglichkeiten auf dem Weg zu Kernbatterien. [Nature, 17. Februar 2021]
Moderne Experimente zur Quantendynamik können die Quantenprozesse der Elektronen in Atomen mit Hilfe von Laserfeldern weitreichend steuern. Das Innenleben der Kerne der Atome spielt dabei aber meist keine Rolle, weil die für sie charakteristischen Energie-, Zeit- und Längenskalen so extrem sind, dass sie durch die Laserfelder praktisch nicht beeinflusst werden. Neue Ansätze bringen frischen Wind in die Kernphysik, indem sie diese Unempfindlichkeit gegen äußere Störungen ausnutzen und die extremen Skalen der Atomkerne für besonders genaue Messungen einsetzen. So können Atomkerne auf Röntgenstrahlen mit einer äußerst genau definierten Energie reagieren, wobei – ähnlich wie Elektronen in der Atomhülle – einzelne Nukleonen angeregt werden. Diese Übergänge können als Taktgeber für präzise Kernuhren verwendet werden und hierzu ist die Vermessung der Kerneigenschaften mit höchster Präzision erforderlich.
Ein Forscherteam um Physiker des Max-Planck-Instituts für Kernphysik in Heidelberg ist nun einen Schritt weiter gegangen, indem es die Quantendynamik der Atomkerne nicht nur vermessen, sondern auch durch geeignet geformte Röntgenpulse mit bisher unerreichter zeitlicher Stabilität von wenigen Zeptosekunden – das ist ein Faktor 100 besser als alles bisher Erreichte – kontrolliert hat. Damit wird der in der optischen Spektroskopie erfolgreich etablierte Werkzeugkasten der kohärenten Kontrolle auch für Atomkerne anwendbar, was ganz neue Möglichkeiten und Perspektiven eröffnet.
Die sogenannte kohärente Kontrolle nutzt die Welleneigenschaften von Materie zur Steuerung von Quantenprozessen durch elektromagnetische Felder, z. B. Laserpulse. Jedes Wellenphänomen ist dabei neben der Frequenz bzw. Wellenlänge durch die Amplitude (Wellenhöhe) und Phase (zeitliche Lage von Wellenbergen und -tälern in der Welle) charakterisiert. Eine einfache Analogie ist die Kontrolle einer schwingenden Schaukel durch periodisches, wellenförmiges Anschubsen. Hierfür muss der genaue Zeitpunkt (Phase) des Schubsens relativ zur Schaukelbewegung gesteuert werden. Stößt man die entgegenkommende Schaukel an, wird sie gebremst. Bewegt sie sich hingegen weg, so wird ihre Auslenkung durch das Anschubsen größer.
Analog dazu lassen sich die quantenmechanischen Eigenschaften von Materie durch entsprechend präziser Steuerung der hierfür verwendeten Laserfelder kontrollieren. In den vergangen Jahrzehnten gab es große Fortschritte und Erfolge bei der kohärenten Kontrolle von Atomen und Molekülen, mit einer zeitlichen Präzision des Lichts bis in den Bereich von Attosekunden, dem milliardsten Teil einer milliardstel Sekunde, was der natürlichen Zeitskala von Elektronen in Atomen entspricht. Wichtige Forschungsziele mit möglichen zukünftigen Anwendungen sind z. B. die Kontrolle von chemischen Reaktionen oder die Entwicklung neuer genauerer Zeitstandards.
In den letzten Jahren hat die Verfügbarkeit von neuartigen Strahlungsquellen für Röntgenstrahlung mit Laserqualität (Synchrotronstrahlung und Freie-Elektronen-Laser) ein neues Gebiet eröffnet: Die nukleare Quantenoptik. Physikern der Abteilungen von Christoph Keitel und Thomas Pfeifer vom Heidelberger Max-Planck-Institut für Kernphysik (MPIK) ist nun am Europäischen Synchrotron ESRF (Grenoble, Frankreich) in Kooperation mit Forschern vom DESY (Hamburg) und dem Helmholtz-Institut/Friedrich-Schiller-Universität (Jena) erstmals der Nachweis kohärenter Kontrolle von Kernanregungen durch Röntgenstrahlung gelungen. Hierbei wurde eine Stabilität der kohärenten Kontrolle von wenigen Zeptosekunden (tausendstel Attosekunden) erreicht.
Im Experiment nutzten die Forscher um Projektleiter Jörg Evers (MPIK) zwei mit dem Eisen-Isotop 57Fe angereicherte Proben, die mit kurzen Röntgenpulsen aus dem Synchrotron bestrahlt werden (Abb. 1). Mit der ersten Probe erzeugten sie einen steuerbaren Doppel-Röntgenpuls, der dann verwendet wurde, um die Dynamik der Kerne in der zweiten Probe zu kontrollieren. Die untersuchten Kernanregungen, die sich durch Röntgenemission wieder abregen, sind als sog. Mößbauer-Übergänge durch eine sehr hohe Energieschärfe gekennzeichnet. Die mit einem Nobelpreis ausgezeichnete Entdeckung des zugrundeliegenden Effekts gelang Rudolf Mößbauer 1958 am MPI für Medizinische Forschung, aus welchem im gleichen Jahr das MPIK ausgegründet wurde.
Um den Doppelpuls zu erzeugen, werden die Kerne der ersten Probe durch den kurzen Röntgenpuls angeregt, und geben diese Anregung aufgrund der hohen Energieschärfe vergleichsweise langsam wieder als einen zweiten Röntgenpuls ab. Im Experiment wird die Probe zwischen der Anregung und der Abregung schnell um eine kleine Distanz bewegt, die etwa der halben Röntgenwellenlänge entspricht. Hierdurch ändert sich die Flugzeit des zweiten Pulses zur zweiten Probe, was die Lage der Wellen der beiden Röntgenpulse (relative Phase) gegeneinander verschiebt.
Dieser Doppelpuls steuert nun die Kerne in der zweiten Probe. Der erste Puls regt eine quantenmechanische Dynamik im Kern an, analog zu der schwingenden Schaukel. Der zweite Puls ändert diese Dynamik, in Abhängigkeit von der relativen Phase der beiden Röntgenpulse. Trifft die Welle des zweiten Pulses beispielsweise im Takt mit der Kerndynamik auf die zweite Probe, so werden die Kerne weiter angeregt. Durch die Variation der relativen Phase konnten die Forscher so gezielt zwischen einer weiteren Anregung der Kerne und einer Abregung der Kerne hin- und herschalten, und damit den quantenmechanischen Zustand der Kerne kontrollieren. Dieser lässt sich aus den gemessenen Interferenzstrukturen der Röntgenstrahlung hinter der zweiten Probe rekonstruieren (Abb. 2).
Eine akustische Analogie soll dies verdeutlichen (siehe Abb. 3) Hierbei entsprechen die Mößbauerkerne der Proben Stimmgabeln die durch einen kurzen Knall („Startschuss“, analog zum Synchrotronpuls) angeregt werden und ihrerseits mit ihrer genau festgelegten Frequenz schwach gedämpft tönen. Der Schall der ersten Gabel trifft also nach dem Knall als zusätzliche Anregung auf die zweite Gabel. Im Fall (a) ist diese Schallwelle gegenphasig zur Bewegung der zweiten Gabel, so dass deren Schwingung abgeregt wird. Im Fall (b) wird die erste Gabel schnell so verschoben, dass ihr Schall stattdessen zur Bewegung der zweiten Gabel passt und sie somit stärker anregt.
Angesichts der erforderlichen extremen Anforderungen an die Kontrolle von Atomkernen (die Verrückung der ersten Probe um eine halbe Wellenlänge liegt in der Größenordnung eines Atomradius) überrascht der offenbar geringe Einfluss äußerer Störungen auf die Güte des Experiments. Dass dies dennoch funktioniert, liegt in der kurzen Dauer einer Messsequenz, während derer die wesentlichen störenden Bewegungen quasi eingefroren sind. Diese Stabilität ist eine Voraussetzung für zukünftige neue Anwendungen auf der Basis von Kernübergängen: genauere Zeitstandards, Untersuchung der Variation von Fundamentalkonstanten oder der Suche nach neuer Physik jenseits der akzeptierten Modelle.
Im Bereich der atomaren Dynamik ist die weitreichende Kontrolle der Schlüssel für viele Anwendungen. Die hier demonstrierten Möglichkeiten öffnen das Tor zu neuen experimentellen Ansätzen, die auf einer Kontrolle der Kerndynamik beruhen, wie etwa genauere Messungen durch Präparation der Kerne in bestimmte Zustände. Insofern zukünftige Röntgenquellen eine stärkere Anregung der Kerne ermöglichen würden, wären damit auch Kernbatterien, die ohne Kernspaltung oder -fusion große Mengen Energie in internen Anregungen der Kerne speichern und wieder abgeben können, denkbar.
Link zur Pressemitteilung des MPIK: https://www.mpi-hd.mpg.de/mpi/de/nachrichten/nachricht/default-12aac64c9f
Originalpublikation:
Coherent X-ray-optical control of nuclear excitons
K. P. Heeg, A. Kaldun, C. Strohm, C. Ott, R. Subramanian, D. Lentrodt, J. Haber, H.-C. Wille, S. Goerttler, R. Rüffer, C. H. Keitel, R. Röhlsberger, T. Pfeifer and J. Evers
Nature 590, 401 (2021), DOI: 10.1038/s41586-021-03276-x
Kontakt
apl. Prof. Dr. Jörg Evers
Forschungsgruppenleiter
Tel.: +49 6221 516-177
Email: joerg.evers@mpi-hd.mpg.de
Dr. Christian Ott
Forschungsgruppenleiter
Tel.: +49 6221 516-577
Email: christian.ott@mpi-hd.mpg.de
Prof. Dr. Thomas Pfeifer
Direktor
Tel.: +49 6221 516-380
Email: thomas.pfeifer@mpi-hd.mpg.de
Honorarprof. Dr. Christoph H. Keitel
Direktor
Tel.: +49 6221 516-150
Email: keitel@mpi-hd.mpg.de
Pressemitteilung MPIK, 17. Februar 2021
Erstmals ist einem Forscherteam des Heidelberger Max-Planck-Instituts für Kernphysik die kohärente Kontrolle von Kernanregungen mit geeignet geformten Röntgenlicht gelungen. In dem am Europäischen Synchrotron ESRF durchgeführten Experiment wurde eine zeitliche Stabilität von wenigen Zeptosekunden erreicht. Zukünftige Experimente basierend auf der Kontrolle der Kerndynamik versprechen genauere Zeitstandards und neue Möglichkeiten auf dem Weg zu Kernbatterien. [Nature, 17. Februar 2021]
Moderne Experimente zur Quantendynamik können die Quantenprozesse der Elektronen in Atomen mit Hilfe von Laserfeldern weitreichend steuern. Das Innenleben der Kerne der Atome spielt dabei aber meist keine Rolle, weil die für sie charakteristischen Energie-, Zeit- und Längenskalen so extrem sind, dass sie durch die Laserfelder praktisch nicht beeinflusst werden. Neue Ansätze bringen frischen Wind in die Kernphysik, indem sie diese Unempfindlichkeit gegen äußere Störungen ausnutzen und die extremen Skalen der Atomkerne für besonders genaue Messungen einsetzen. So können Atomkerne auf Röntgenstrahlen mit einer äußerst genau definierten Energie reagieren, wobei – ähnlich wie Elektronen in der Atomhülle – einzelne Nukleonen angeregt werden. Diese Übergänge können als Taktgeber für präzise Kernuhren verwendet werden und hierzu ist die Vermessung der Kerneigenschaften mit höchster Präzision erforderlich.
Ein Forscherteam um Physiker des Max-Planck-Instituts für Kernphysik in Heidelberg ist nun einen Schritt weiter gegangen, indem es die Quantendynamik der Atomkerne nicht nur vermessen, sondern auch durch geeignet geformte Röntgenpulse mit bisher unerreichter zeitlicher Stabilität von wenigen Zeptosekunden – das ist ein Faktor 100 besser als alles bisher Erreichte – kontrolliert hat. Damit wird der in der optischen Spektroskopie erfolgreich etablierte Werkzeugkasten der kohärenten Kontrolle auch für Atomkerne anwendbar, was ganz neue Möglichkeiten und Perspektiven eröffnet.
Die sogenannte kohärente Kontrolle nutzt die Welleneigenschaften von Materie zur Steuerung von Quantenprozessen durch elektromagnetische Felder, z. B. Laserpulse. Jedes Wellenphänomen ist dabei neben der Frequenz bzw. Wellenlänge durch die Amplitude (Wellenhöhe) und Phase (zeitliche Lage von Wellenbergen und -tälern in der Welle) charakterisiert. Eine einfache Analogie ist die Kontrolle einer schwingenden Schaukel durch periodisches, wellenförmiges Anschubsen. Hierfür muss der genaue Zeitpunkt (Phase) des Schubsens relativ zur Schaukelbewegung gesteuert werden. Stößt man die entgegenkommende Schaukel an, wird sie gebremst. Bewegt sie sich hingegen weg, so wird ihre Auslenkung durch das Anschubsen größer.
Analog dazu lassen sich die quantenmechanischen Eigenschaften von Materie durch entsprechend präziser Steuerung der hierfür verwendeten Laserfelder kontrollieren. In den vergangen Jahrzehnten gab es große Fortschritte und Erfolge bei der kohärenten Kontrolle von Atomen und Molekülen, mit einer zeitlichen Präzision des Lichts bis in den Bereich von Attosekunden, dem milliardsten Teil einer milliardstel Sekunde, was der natürlichen Zeitskala von Elektronen in Atomen entspricht. Wichtige Forschungsziele mit möglichen zukünftigen Anwendungen sind z. B. die Kontrolle von chemischen Reaktionen oder die Entwicklung neuer genauerer Zeitstandards.
In den letzten Jahren hat die Verfügbarkeit von neuartigen Strahlungsquellen für Röntgenstrahlung mit Laserqualität (Synchrotronstrahlung und Freie-Elektronen-Laser) ein neues Gebiet eröffnet: Die nukleare Quantenoptik. Physikern der Abteilungen von Christoph Keitel und Thomas Pfeifer vom Heidelberger Max-Planck-Institut für Kernphysik (MPIK) ist nun am Europäischen Synchrotron ESRF (Grenoble, Frankreich) in Kooperation mit Forschern vom DESY (Hamburg) und dem Helmholtz-Institut/Friedrich-Schiller-Universität (Jena) erstmals der Nachweis kohärenter Kontrolle von Kernanregungen durch Röntgenstrahlung gelungen. Hierbei wurde eine Stabilität der kohärenten Kontrolle von wenigen Zeptosekunden (tausendstel Attosekunden) erreicht.
Im Experiment nutzten die Forscher um Projektleiter Jörg Evers (MPIK) zwei mit dem Eisen-Isotop 57Fe angereicherte Proben, die mit kurzen Röntgenpulsen aus dem Synchrotron bestrahlt werden (Abb. 1). Mit der ersten Probe erzeugten sie einen steuerbaren Doppel-Röntgenpuls, der dann verwendet wurde, um die Dynamik der Kerne in der zweiten Probe zu kontrollieren. Die untersuchten Kernanregungen, die sich durch Röntgenemission wieder abregen, sind als sog. Mößbauer-Übergänge durch eine sehr hohe Energieschärfe gekennzeichnet. Die mit einem Nobelpreis ausgezeichnete Entdeckung des zugrundeliegenden Effekts gelang Rudolf Mößbauer 1958 am MPI für Medizinische Forschung, aus welchem im gleichen Jahr das MPIK ausgegründet wurde.
Um den Doppelpuls zu erzeugen, werden die Kerne der ersten Probe durch den kurzen Röntgenpuls angeregt, und geben diese Anregung aufgrund der hohen Energieschärfe vergleichsweise langsam wieder als einen zweiten Röntgenpuls ab. Im Experiment wird die Probe zwischen der Anregung und der Abregung schnell um eine kleine Distanz bewegt, die etwa der halben Röntgenwellenlänge entspricht. Hierdurch ändert sich die Flugzeit des zweiten Pulses zur zweiten Probe, was die Lage der Wellen der beiden Röntgenpulse (relative Phase) gegeneinander verschiebt.
Dieser Doppelpuls steuert nun die Kerne in der zweiten Probe. Der erste Puls regt eine quantenmechanische Dynamik im Kern an, analog zu der schwingenden Schaukel. Der zweite Puls ändert diese Dynamik, in Abhängigkeit von der relativen Phase der beiden Röntgenpulse. Trifft die Welle des zweiten Pulses beispielsweise im Takt mit der Kerndynamik auf die zweite Probe, so werden die Kerne weiter angeregt. Durch die Variation der relativen Phase konnten die Forscher so gezielt zwischen einer weiteren Anregung der Kerne und einer Abregung der Kerne hin- und herschalten, und damit den quantenmechanischen Zustand der Kerne kontrollieren. Dieser lässt sich aus den gemessenen Interferenzstrukturen der Röntgenstrahlung hinter der zweiten Probe rekonstruieren (Abb. 2).
Eine akustische Analogie soll dies verdeutlichen (siehe Abb. 3) Hierbei entsprechen die Mößbauerkerne der Proben Stimmgabeln die durch einen kurzen Knall („Startschuss“, analog zum Synchrotronpuls) angeregt werden und ihrerseits mit ihrer genau festgelegten Frequenz schwach gedämpft tönen. Der Schall der ersten Gabel trifft also nach dem Knall als zusätzliche Anregung auf die zweite Gabel. Im Fall (a) ist diese Schallwelle gegenphasig zur Bewegung der zweiten Gabel, so dass deren Schwingung abgeregt wird. Im Fall (b) wird die erste Gabel schnell so verschoben, dass ihr Schall stattdessen zur Bewegung der zweiten Gabel passt und sie somit stärker anregt.
Angesichts der erforderlichen extremen Anforderungen an die Kontrolle von Atomkernen (die Verrückung der ersten Probe um eine halbe Wellenlänge liegt in der Größenordnung eines Atomradius) überrascht der offenbar geringe Einfluss äußerer Störungen auf die Güte des Experiments. Dass dies dennoch funktioniert, liegt in der kurzen Dauer einer Messsequenz, während derer die wesentlichen störenden Bewegungen quasi eingefroren sind. Diese Stabilität ist eine Voraussetzung für zukünftige neue Anwendungen auf der Basis von Kernübergängen: genauere Zeitstandards, Untersuchung der Variation von Fundamentalkonstanten oder der Suche nach neuer Physik jenseits der akzeptierten Modelle.
Im Bereich der atomaren Dynamik ist die weitreichende Kontrolle der Schlüssel für viele Anwendungen. Die hier demonstrierten Möglichkeiten öffnen das Tor zu neuen experimentellen Ansätzen, die auf einer Kontrolle der Kerndynamik beruhen, wie etwa genauere Messungen durch Präparation der Kerne in bestimmte Zustände. Insofern zukünftige Röntgenquellen eine stärkere Anregung der Kerne ermöglichen würden, wären damit auch Kernbatterien, die ohne Kernspaltung oder -fusion große Mengen Energie in internen Anregungen der Kerne speichern und wieder abgeben können, denkbar.
Link zur Pressemitteilung des MPIK: https://www.mpi-hd.mpg.de/mpi/de/nachrichten/nachricht/default-12aac64c9f
Originalpublikation:
Coherent X-ray-optical control of nuclear excitons
K. P. Heeg, A. Kaldun, C. Strohm, C. Ott, R. Subramanian, D. Lentrodt, J. Haber, H.-C. Wille, S. Goerttler, R. Rüffer, C. H. Keitel, R. Röhlsberger, T. Pfeifer and J. Evers
Nature 590, 401 (2021), DOI: 10.1038/s41586-021-03276-x
Kontakt
apl. Prof. Dr. Jörg Evers
Forschungsgruppenleiter
Tel.: +49 6221 516-177
Email: joerg.evers@mpi-hd.mpg.de
Dr. Christian Ott
Forschungsgruppenleiter
Tel.: +49 6221 516-577
Email: christian.ott@mpi-hd.mpg.de
Prof. Dr. Thomas Pfeifer
Direktor
Tel.: +49 6221 516-380
Email: thomas.pfeifer@mpi-hd.mpg.de
Honorarprof. Dr. Christoph H. Keitel
Direktor
Tel.: +49 6221 516-150
Email: keitel@mpi-hd.mpg.de
Press Release MPIK, 17 December 2021
A team of researchers from the Max Planck Institute for Nuclear Physics in Heidelberg has coherently controlled nuclear excitations using suitably shaped X-ray light for the first time. In the experiment performed at the European Synchrotron ESRF, they achieved a temporal control stability of a few zeptoseconds. This forms the basis for new experimental approaches exploiting the control of nuclear dynamics which could lead to more precise future time standards and open new possibilities on the way to nuclear batteries. [Nature, 17 February 2021]
Modern experiments on quantum dynamics can control the quantum processes of electrons in atoms to a large extent by means of laser fields. However, the inner life of atomic nuclei usually plays no role because their characteristic energy, time and length scales are so extreme that they are practically unaffected by the laser fields. Fresh approaches breathe new life into nuclear physics by exploiting this insensitivity to external disturbances and using the extreme scales of the atomic nuclei for particularly precise measurements. Thus, atomic nuclei can respond to X-rays with an extremely well-defined energy by exciting individual nucleons – similar to electrons in the atomic shell. These transitions can be used as clockworks for precise nuclear clocks, and this requires the measurement of nuclear properties with the highest precision.
A team of researchers around physicists from the Max Planck Institute for Nuclear Physics in Heidelberg has now gone a step forward by not only measuring the quantum dynamics of atomic nuclei, but also controlling them using suitably shaped X-ray pulses with previously unattained temporal stability of a few zeptoseconds – a factor of 100 better than anything previously achieved. This opens the toolbox of coherent control, which has been successfully established in optical spectroscopy, to atomic nuclei – providing completely new possibilities and perspectives.
So-called coherent control uses the wave properties of matter to control quantum processes via electromagnetic fields, e.g. laser pulses. In addition to the frequency or wavelength, each wave phenomenon is characterised by the amplitude (wave height) and phase (temporal position of wave crests and troughs). A simple analogy is the control of an oscillating swing by periodic, wave-like pushing. For this, the exact timing (phase) of the push relative to the swing motion has to be controlled. If the oncoming swing is pushed, it is decelerated. If, on the other hand, it moves away, its deflection is increased by the push.
Analogously, the quantum-mechanical properties of matter can be controlled via correspondingly precise steering of the applied laser fields. In the past decades, there has been great progress and success in the coherent control of atoms and molecules, with a temporal precision of light down to the attosecond range, the billionth part of a billionth of a second, which corresponds to the natural time scale of electrons in atoms. Important research goals with possible future applications are, for example, the control of chemical reactions or the development of new, more precise time standards.
In recent years, the availability of novel radiation sources for X-rays with laser quality (synchrotron radiation and free-electron lasers) has opened up a new field: nuclear quantum optics. Physicists from the departments of Christoph Keitel and Thomas Pfeifer at the Max Planck Institute for Nuclear Physics (MPIK) in Heidelberg have now succeeded for the first time in demonstrating coherent control of nuclear excitations by X-rays at the European Synchrotron ESRF (Grenoble, France) in cooperation with researchers from DESY (Hamburg) and the Helmholtz Institute/Friedrich Schiller University (Jena). A stability of the coherent control of a few zeptoseconds (a thousandth of an attosecond) was achieved.
In the experiment, the researchers around project leader Jörg Evers (MPIK) used two samples enriched with the iron isotope 57Fe, which are irradiated with short X-ray pulses from the synchrotron (Fig. 1). In the first sample, they generated a controllable double X-ray pulse, which was then used to control the dynamics of the nuclei in the second sample. The investigated nuclear excitations – which de-excite again by X-ray emission – are characterised by a very high sharpness in energy: so-called Mössbauer transitions. The discovery of the underlying effect (Nobel Prize 1961) was made by Rudolf Mössbauer in 1958 at the MPI for Medical Research, from which the MPIK spun off in the same year.
To generate the double pulse, the nuclei of the first sample are excited by the short X-ray pulse and, due to the high energy sharpness, release this excitation comparably slow in form of a second X-ray pulse. In the experiment, the sample is shifted quickly between the excitation and the de-excitation by a small distance corresponding to about half the X-ray wavelength. This changes the time of flight of the second pulse to the second sample, and thus shifts the position of the waves of the two X-ray pulses (relative phase) with respect to each other.
This double pulse now allows to control the nuclei in the second sample. The first pulse excites a quantum-mechanical dynamic in the nucleus, analogous to the oscillating swing. The second pulse changes this dynamic, depending on the relative phase of the two X-ray pulses. For example, if the wave of the second pulse hits the second sample in phase with the nuclear dynamics, the nuclei are further excited. By varying the relative phase, the researchers were able to switch between further excitation of the nuclei and de-excitation of the nuclei, and thus control the quantum-mechanical state of the nuclei. This can be reconstructed from the measured interference structures of the X-ray radiation behind the second sample (Fig. 2).
An acoustic analogy is illustrated in Fig. 3: Here, the Mössbauer nuclei of the samples correspond to tuning forks that are excited by a short bang (“starting shot”, analogous to the synchrotron pulse) and in turn sound slightly damped with their precisely defined frequency. The sound of the first fork thus hits the second fork after the bang as an additional excitation. In case (a), this sound wave moves opposite to the second fork, so that its oscillation is de-excited. In case (b), the first fork is quickly shifted so that its sound matches the movement of the second fork instead and thus excites it more.
Given the extreme demands required to control atomic nuclei (the displacement of the first sample by half a wavelength is of the order of an atomic radius), the apparently small influence of external disturbances on the quality of the experiment is surprising. Nevertheless, this works – due to the short duration of a measurement sequence, during which the main disturbing movements are virtually frozen. This stability is a prerequisite for future new applications based on nuclear transitions: more precise time standards, investigation of the variation of fundamental constants or the search for new physics beyond the accepted models.
In the field of atomic dynamics, far-reaching control is the key to many applications. The possibilities demonstrated here open the door to new experimental approaches based on the control of nuclear dynamics, e.g. by preparing nuclei in particular quantum states allowing for more precise measurements. Insofar as future X-ray sources would enable stronger excitation of the nuclei, nuclear batteries that can store and release large amounts of energy in internal excitations of the nuclei without nuclear fission or fusion would also be conceivable.
Original Publication
Coherent X-ray-optical control of nuclear excitons
K. P. Heeg, A. Kaldun, C. Strohm, C. Ott, R. Subramanian, D. Lentrodt, J. Haber, H.-C. Wille, S. Goerttler, R. Rüffer, C. H. Keitel, R. Röhlsberger, T. Pfeifer and J. Evers
Nature 590, 401 (2021), DOI: 10.1038/s41586-021-03276-x
Contact
apl. Prof. Dr. Jörg Evers
Research group leader
Phone: +49 6221 516-177
Email: joerg.evers@mpi-hd.mpg.de
Dr. Christian Ott
Research group leader
Phone: +49 6221 516-577
Email: christian.ott@mpi-hd.mpg.de
Prof. Dr. Thomas Pfeifer
Director
Phone: +49 6221 516-380
Email: thomas.pfeifer@mpi-hd.mpg.de
Honorarprof. Dr. Christoph H. Keitel
Director
Phone: +49 6221 516-150
Email: keitel@mpi-hd.mpg.de
Press Release MPIK, 17 December 2021
A team of researchers from the Max Planck Institute for Nuclear Physics in Heidelberg has coherently controlled nuclear excitations using suitably shaped X-ray light for the first time. In the experiment performed at the European Synchrotron ESRF, they achieved a temporal control stability of a few zeptoseconds. This forms the basis for new experimental approaches exploiting the control of nuclear dynamics which could lead to more precise future time standards and open new possibilities on the way to nuclear batteries. [Nature, 17 February 2021]
Modern experiments on quantum dynamics can control the quantum processes of electrons in atoms to a large extent by means of laser fields. However, the inner life of atomic nuclei usually plays no role because their characteristic energy, time and length scales are so extreme that they are practically unaffected by the laser fields. Fresh approaches breathe new life into nuclear physics by exploiting this insensitivity to external disturbances and using the extreme scales of the atomic nuclei for particularly precise measurements. Thus, atomic nuclei can respond to X-rays with an extremely well-defined energy by exciting individual nucleons – similar to electrons in the atomic shell. These transitions can be used as clockworks for precise nuclear clocks, and this requires the measurement of nuclear properties with the highest precision.
A team of researchers around physicists from the Max Planck Institute for Nuclear Physics in Heidelberg has now gone a step forward by not only measuring the quantum dynamics of atomic nuclei, but also controlling them using suitably shaped X-ray pulses with previously unattained temporal stability of a few zeptoseconds – a factor of 100 better than anything previously achieved. This opens the toolbox of coherent control, which has been successfully established in optical spectroscopy, to atomic nuclei – providing completely new possibilities and perspectives.
So-called coherent control uses the wave properties of matter to control quantum processes via electromagnetic fields, e.g. laser pulses. In addition to the frequency or wavelength, each wave phenomenon is characterised by the amplitude (wave height) and phase (temporal position of wave crests and troughs). A simple analogy is the control of an oscillating swing by periodic, wave-like pushing. For this, the exact timing (phase) of the push relative to the swing motion has to be controlled. If the oncoming swing is pushed, it is decelerated. If, on the other hand, it moves away, its deflection is increased by the push.
Analogously, the quantum-mechanical properties of matter can be controlled via correspondingly precise steering of the applied laser fields. In the past decades, there has been great progress and success in the coherent control of atoms and molecules, with a temporal precision of light down to the attosecond range, the billionth part of a billionth of a second, which corresponds to the natural time scale of electrons in atoms. Important research goals with possible future applications are, for example, the control of chemical reactions or the development of new, more precise time standards.
In recent years, the availability of novel radiation sources for X-rays with laser quality (synchrotron radiation and free-electron lasers) has opened up a new field: nuclear quantum optics. Physicists from the departments of Christoph Keitel and Thomas Pfeifer at the Max Planck Institute for Nuclear Physics (MPIK) in Heidelberg have now succeeded for the first time in demonstrating coherent control of nuclear excitations by X-rays at the European Synchrotron ESRF (Grenoble, France) in cooperation with researchers from DESY (Hamburg) and the Helmholtz Institute/Friedrich Schiller University (Jena). A stability of the coherent control of a few zeptoseconds (a thousandth of an attosecond) was achieved.
In the experiment, the researchers around project leader Jörg Evers (MPIK) used two samples enriched with the iron isotope 57Fe, which are irradiated with short X-ray pulses from the synchrotron (Fig. 1). In the first sample, they generated a controllable double X-ray pulse, which was then used to control the dynamics of the nuclei in the second sample. The investigated nuclear excitations – which de-excite again by X-ray emission – are characterised by a very high sharpness in energy: so-called Mössbauer transitions. The discovery of the underlying effect (Nobel Prize 1961) was made by Rudolf Mössbauer in 1958 at the MPI for Medical Research, from which the MPIK spun off in the same year.
To generate the double pulse, the nuclei of the first sample are excited by the short X-ray pulse and, due to the high energy sharpness, release this excitation comparably slow in form of a second X-ray pulse. In the experiment, the sample is shifted quickly between the excitation and the de-excitation by a small distance corresponding to about half the X-ray wavelength. This changes the time of flight of the second pulse to the second sample, and thus shifts the position of the waves of the two X-ray pulses (relative phase) with respect to each other.
This double pulse now allows to control the nuclei in the second sample. The first pulse excites a quantum-mechanical dynamic in the nucleus, analogous to the oscillating swing. The second pulse changes this dynamic, depending on the relative phase of the two X-ray pulses. For example, if the wave of the second pulse hits the second sample in phase with the nuclear dynamics, the nuclei are further excited. By varying the relative phase, the researchers were able to switch between further excitation of the nuclei and de-excitation of the nuclei, and thus control the quantum-mechanical state of the nuclei. This can be reconstructed from the measured interference structures of the X-ray radiation behind the second sample (Fig. 2).
An acoustic analogy is illustrated in Fig. 3: Here, the Mössbauer nuclei of the samples correspond to tuning forks that are excited by a short bang (“starting shot”, analogous to the synchrotron pulse) and in turn sound slightly damped with their precisely defined frequency. The sound of the first fork thus hits the second fork after the bang as an additional excitation. In case (a), this sound wave moves opposite to the second fork, so that its oscillation is de-excited. In case (b), the first fork is quickly shifted so that its sound matches the movement of the second fork instead and thus excites it more.
Given the extreme demands required to control atomic nuclei (the displacement of the first sample by half a wavelength is of the order of an atomic radius), the apparently small influence of external disturbances on the quality of the experiment is surprising. Nevertheless, this works – due to the short duration of a measurement sequence, during which the main disturbing movements are virtually frozen. This stability is a prerequisite for future new applications based on nuclear transitions: more precise time standards, investigation of the variation of fundamental constants or the search for new physics beyond the accepted models.
In the field of atomic dynamics, far-reaching control is the key to many applications. The possibilities demonstrated here open the door to new experimental approaches based on the control of nuclear dynamics, e.g. by preparing nuclei in particular quantum states allowing for more precise measurements. Insofar as future X-ray sources would enable stronger excitation of the nuclei, nuclear batteries that can store and release large amounts of energy in internal excitations of the nuclei without nuclear fission or fusion would also be conceivable.
Original Publication
Coherent X-ray-optical control of nuclear excitons
K. P. Heeg, A. Kaldun, C. Strohm, C. Ott, R. Subramanian, D. Lentrodt, J. Haber, H.-C. Wille, S. Goerttler, R. Rüffer, C. H. Keitel, R. Röhlsberger, T. Pfeifer and J. Evers
Nature 590, 401 (2021), DOI: 10.1038/s41586-021-03276-x
Contact
apl. Prof. Dr. Jörg Evers
Research group leader
Phone: +49 6221 516-177
Email: joerg.evers@mpi-hd.mpg.de
Dr. Christian Ott
Research group leader
Phone: +49 6221 516-577
Email: christian.ott@mpi-hd.mpg.de
Prof. Dr. Thomas Pfeifer
Director
Phone: +49 6221 516-380
Email: thomas.pfeifer@mpi-hd.mpg.de
Honorarprof. Dr. Christoph H. Keitel
Director
Phone: +49 6221 516-150
Email: keitel@mpi-hd.mpg.de
Abstract:
Coherent control of quantum dynamics is key to a multitude of fundamental studies and applications. In the visible or longer-wavelength domains, near-resonant light fields have become the primary tool with which to control electron dynamics. Recently, coherent control in the extreme-ultraviolet range was demonstrated, with a few-attosecond temporal resolution of the phase control. At hard-X-ray energies (above 5–10 kiloelectronvolts), Mössbauer nuclei feature narrow nuclear resonances due to their recoilless absorption and emission of light, and spectroscopy of these resonances is widely used to study the magnetic, structural and dynamical properties of matter. It has been shown that the power and scope of Mössbauer spectroscopy can be greatly improved using various control techniques. However, coherent control of atomic nuclei using suitably shaped near-resonant X-ray fields remains an open challenge. Here we demonstrate such control, and use the tunable phase between two X-ray pulses to switch the nuclear exciton dynamics between coherent enhanced excitation and coherent enhanced emission. We present a method of shaping single pulses delivered by state-of-the-art X-ray facilities into tunable double pulses, and demonstrate a temporal stability of the phase control on the few-zeptosecond timescale. Our results unlock coherent optical control for nuclei, and pave the way for nuclear Ramsey spectroscopy and spin-echo-like techniques, which should not only advance nuclear quantum optics, but also help to realize X-ray clocks and frequency standards. In the long term, we envision time-resolved studies of nuclear out-of-equilibrium dynamics, which is a long-standing challenge in Mössbauer science.
K. P. Heeg, A. Kaldun, C. Strohm, C. Ott, R. Subramanian, D. Lentrodt, J. Haber, H.-C. Wille, S. Goerttler, R. Rüffer, C. H. Keitel, R. Röhlsberger, T. Pfeifer, and J. Evers, “Coherent X-rayoptical control of nuclear excitons”, Nature 590, 401 (2021).
https://www.nature.com/articles/s41586-021-03276-x
Related to Project B02
Abstract:
We present an improved scheme for absorption imaging of alkali atoms at moderate magnetic fields, where the excited state is well in the Paschen–Back regime, but the ground state hyperfine manifold is not. It utilizes four atomic levels to obtain an approximately closed optical cycle. With the resulting absorption of the corresponding two laser frequencies, we extract the atomic column density of a 39K Bose–Einstein condensate. The scheme can be readily applied to all other alkali-like species.
M. Hans, F. Schmutte, C. Viermann, N. Liebster, M. Sparn, M. K. Oberthaler, H. Strobel, “High signal to noise absorption imaging of alkali atoms at moderate magnetic fields”, Rev. Sci. Instrum. 92, 023203 (2021).
https://aip.scitation.org/doi/10.1063/5.0040677
Related to Project B04
Abstract:
In this paper, we investigate the dynamical quantum phase transitions appearing in the Loschmidt echo and the time-dependent order parameter of a quantum system of harmonically coupled degenerate bosons as a function of the power-law decay σ of long-range interactions. Following a sudden quench, the nonequilibrium dynamics of this system are governed by a set of nonlinear coupled Ermakov equations. To solve them, we develop an analytical approximation valid at late times. Based on this approximation, we show that the emergence of a dynamical quantum phase transition hinges on the generation of a finite mass gap following the quench, starting from a massless initial state. In general, we can define two distinct dynamical phases characterized by the finiteness of the post-quench mass gap. The Loschmidt echo exhibits periodical nonanalytic cusps whenever the initial state has a vanishing mass gap and the final state has a finite mass gap. These cusps are shown to coincide with the maxima of the time-dependent long-range correlations.
M. Syed, T. Enss, and N. Defenu, “Dynamical quantum phase transition in a bosonic system with long-range interactions”, Phys. Rev. B 103, 064306 (2021).
https://journals.aps.org/prb/abstract/10.1103/PhysRevB.103.064306
Related to Project: C02, C03
Abstract:
Promising searches for new physics beyond the current Standard Model (SM) of particle physics are feasible through isotope-shift spectroscopy, which is sensitive to a hypothetical fifth force between the neutrons of the nucleus and the electrons of the shell. Such an interaction would be mediated by a new particle which could in principle be associated with dark matter. In so-called King plots, the mass-scaled frequency shifts of two optical transitions are plotted against each other for a series of isotopes. Subtle deviations from the expected linearity could reveal such a fifth force. Here, we study experimentally and theoretically six transitions in highly charged ions of Ca, an element with five stable isotopes of zero nuclear spin. Some of the transitions are suitable for upcoming high-precision coherent laser spectroscopy. Combining them with clock transitions in singly charged Ca+ ions enables application of a generalized King plot scheme that allows for eliminating higher-order SM-related nonlinearities, thereby increasing the sensitivity of ongoing searches for unknown forces and particles.
N.-H. Rehbehn, M. K. Rosner, J. C. Berengut, P. O. Schmidt, S. A. King, P. Micke, M. F. Gu, R. Müller, A. Surzhykov, and J. R. Crespo López-Urrutia, “Sensitivity to New Physics of Isotope Shift Studies using the Coronal Lines of Highly Charged Calcium Ions”, arXiv:2102.02309 (2021).
https://arxiv.org/abs/2102.02309
Related to Project B01
Abstract:
A general prediction from asymptotically safe quantum gravity is the approximate vanishing of all quartic scalar couplings at the UV fixed point beyond the Planck scale. A vanishing Higgs doublet quartic coupling near the Planck scale translates into a prediction for the ratio between the mass of the Higgs boson MH and the top quark Mt. If only the standard model particles contribute to the running of couplings below the Planck mass, the observed MH ∼ 125 GeV results in the prediction for the top quark mass Mt ∼ 171 GeV, in agreement with recent measurements. In this work, we study how the asymptotic safety prediction for the top quark mass is affected by possible physics at an intermediate scale. We investigate the effect of an SU(2) triplet scalar and right-handed neutrinos, needed to explain the tiny mass of left-handed neutrinos. For pure seesaw II, with no or very heavy right handed neutrinos, the top mass can increase to Mt ∼ 172.5 GeV for a triplet mass of M∆ ∼ 108GeV. Right handed neutrino masses at an intermediate scale increase the uncertainty of the predictions of Mt due to unknown Yukawa couplings of the right-handed neutrinos and a cubic interaction in the scalar potential. For an appropriate range of Yukawa couplings there is no longer an issue of vacuum stability.
G. Domènech, M. Goodsell, C.Wetterich, “Neutrino masses, vacuum stability and quantum gravity prediction for the mass of the top quark”, JHEP 01, 180 (2021).
https://link.springer.com/article/10.1007/JHEP01(2021)180
Related to Project
The CRC1225 ISOQUANT is happy to announce the start of its first SciArt Residency programme by opening the call for applications. The residency programme is jointly established with Kalamari Klub in the framework of an overall SciArt collaboration project and wants to encourage proposals by artists and enthusiasts who are aiming to develop a body of work based on creation, collaboration and experimentation within an artistic research process. The residency is scheduled for September to October 2021 and will be located in Heidelberg, Germany.
With the SciArt Residency programme, we aim to initiate curiosity-driven engagement between artists, scientists and other members of the society, promoting a spirit of co-learning and equality while helping to communicate knowledge through cross-disciplinary collaboration. A central part of the residency will be the active cooperation and exchange with the scientists of the collaborative research centre. Before the beginning of the residency, the selected artist is invited to give a short presentation about their previous projects and research interests in order to initiate a conversation between the involved scientists and the artist. During the SciArt Residency, scientists and members of the Kalamari Klub will be available to support collaboration, and the artist will be given the opportunity to further develop their research project and focus on their work. It is important to note the programme’s approach as „open prospect“: the form, process and outcome of the residency is entirely up to the artist and their collaborators. Additional funding for an exhibition, talk, workshop or other forms of presentation of the work done during the residency is available.
The application is open to all artists and photographers working with analogue and experimental photography, as well as all those who are working within science-influenced art and photography. While affinity for scientific topics is of advantage, no science knowledge is required. The minimum age at date of application is 18 years.
Further information on the SciArt Residency in general and how to apply can be found here: https://www.kalamariklub.org/sciart-residency-2021
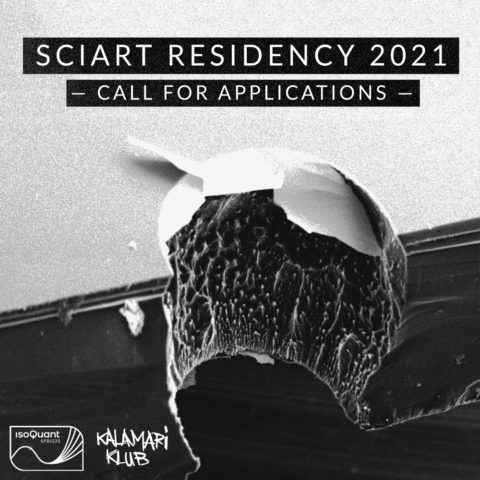
Profile of a plasma etched silicon wafer @Dennis Schulz (Kalamari Klub)
Abstract:
The process of emission of electromagnetic radiation does not occur instantaneously but it is “formed” over a finite time known as radiation formation time. In the ultrarelativistic regime, the corresponding (longitudinal) formation length is given by the formation time times the speed of light and controls several features of radiation. Here, we elucidate the importance of the transverse formation length (TFL) by investigating nonlinear Compton scattering by an electron counterpropagating with respect to a flying focus laser beam. The TFL is related to the transverse size of the radiation formation “volume” and, unlike the longitudinal formation length, has a quantum origin. Being the TFL typically of the order of the Compton wavelength, where any laser field can be assumed to be approximately uniform, related quantum interference effects can be ignored. However, we show analytically that if the focus in a flying focus beam with nL≫1 cycles moves at the speed of light and backwards with respect to the beam propagation direction, the effects of the TFL undergo a large enhancement proportional to nL and can substantially alter the emission spectrum for feasible flying focus pulses.
A. Di Piazza, “Unveiling the transverse formation length of nonlinear Compton scattering”, Phys. Rev. A 103, 012215 (2021).
https://journals.aps.org/pra/abstract/10.1103/PhysRevA.103.012215
Related to Project B02
Abstract:
Understanding the dynamics of strongly interacting disordered quantum systems is one of the most challenging problems in modern science, due to features such as the breakdown of thermalization and the emergence of glassy phases of matter. We report on the observation of anomalous relaxation dynamics in an isolated XXZ quantum spin system realized by an ultracold gas of atoms initially prepared in a superposition of two different Rydberg states. The total magnetization is found to exhibit subexponential relaxation analogous to classical glassy dynamics, but in the quantum case this relaxation originates from the buildup of nonclassical correlations. In both experiment and semiclassical simulations, we find the evolution toward a randomized state is independent of the strength of disorder up to a critical value. This hints toward a unifying description of relaxation dynamics in disordered isolated quantum systems, analogous to the generalization of statistical mechanics to out-of-equilibrium scenarios in classical spin glasses.
A. Signoles, T. Franz, R. F. Alves, M. Gärttner, S. Whitlock, G. Zürn, and M. Weidemüller, “Glassy dynamics in a disordered Heisenberg quantum spin system”, Phys. Rev. X 11, 011011 (2021)
https://journals.aps.org/prx/abstract/10.1103/PhysRevX.11.011011
Related to Project: A05
Abstract:
Gaussian models provide an excellent effective description of many quantum many-body systems ranging from condensed-matter systems all the way to neutron stars. Gaussian states are common at equilibrium when the interactions are weak. Recently it was proposed that they can also emerge dynamically from a non-Gaussian initial state evolving under non-interacting dynamics. Here we present the experimental observation of such a dynamical emergence of Gaussian correlations in a quantum many-body system. This non-equilibrium evolution is triggered by abruptly switching off the effective interaction between the observed collective degrees of freedom, while leaving the interactions between the microscopic constituents unchanged. Starting from highly non-Gaussian correlations, consistent with the sine–Gordon model, we observe a Gaussian state emerging over time as revealed by the decay of the fourth- and sixth-order connected correlations in the quantum field. A description of this dynamics requires a novel mechanism for the emergence of Gaussian correlations, which is relevant for a wide class of quantum many-body systems. In our closed system with non-interacting effective degrees of freedom, we do not expect full thermalization. This memory of the initial state is confirmed by observing recurrences of non-Gaussian correlations.
T. Schweigler, M. Gluza, M. Tajik, S. Sotiriadis, F. Cataldini, S.-C. Ji, F. S. Møller, J. Sabino, B. Rauer, J. Eisert, J. Schmiedmayer, “Decay and recurrence of non-Gaussian correlations in a quantum many-body system”, Nat. Phys. 17 (2021).
https://www.nature.com/articles/s41567-020-01139-2
Related to Project A03
Abstract:
We have developed an extreme ultraviolet (XUV) frequency comb for performing ultra-high precision spectroscopy on the many XUV transitions found in highly charged ions (HCI). Femtosecond pulses from a 100 MHz phase-stabilized near-infrared frequency comb are amplified and then fed into a femtosecond enhancement cavity (fsEC) inside an ultra-high vacuum chamber. The low-dispersion fsEC coherently superposes several hundred incident pulses and, with a single cylindrical optical element, fully compensates astigmatism at the w0 = 15 µm waist cavity focus. With a gas jet installed there, intensities reaching ∼ 1014 W/cm2 generate coherent high harmonics with a comb spectrum at 100 MHz rate. We couple out of the fsEC harmonics from the 7th up to the 35th (42 eV; 30 nm) to be used in upcoming experiments on HCI frequency metrology.
J. Nauta, J.-H. Oelmann, A. Borodin, A. Ackermann, P. Knauer, I. S. Muhammad, R. Pappenberger, T. Pfeifer, and J. R. C. López-Urrutia, “XUV frequency comb production with an astigmatism-compensated enhancement cavity”, Opt. Express 29, 2624 (2021)
https://www.osapublishing.org/oe/fulltext.cfm?uri=oe-29-2-2624&id=446601
Related to Project: B01
Pressemitteilung Universität Heidelberg
16. November 2021
Wie passen analoge Fotografie und innovative Forschung im Bereich der Quantenphysik zusammen? Diese Frage steht im Mittelpunkt einer Veranstaltung mit dem Fotografen Philip Kanwischer, zu der der Sonderforschungsbereich (SFB) „IsoQuant“ der Universität Heidelberg gemeinsam mit dem Kalamari Klub einlädt. Derzeit ist der Leipziger Künstler zu Gast an dem in der Physik angesiedelten SFB, um den Dialog zwischen Kunst und Wissenschaft zu fördern und für die interessierte Öffentlichkeit sichtbar zu machen. Der Artist Talk „New Links Between Different Systems“ findet am 18. November 2021 in englischer Sprache im Heidelberger Café Leitstelle statt und beginnt um 19 Uhr, parallel kann auch ein Livestream abgerufen werden. Für die Teilnahme ist eine Anmeldung erforderlich.
Philip Kanwischer konnte sich in einem Auswahlverfahren für die Besetzung der erstmals ausgeschriebenen SciArt Residency des in der Physik angesiedelten SFB 1225 „Isolierte Quantensysteme und Universalität unter extremen Bedingungen“ (IsoQuant) durchsetzen – eine Initiative zur Förderung der Kooperation zwischen Wissenschaft und Kunst. In diesem Rahmen arbeitet er seit Oktober mit den Wissenschaftlerinnen und Wissenschaftlern des SFB zusammen. Neben dem Artist Talk sind weitere Aktionen sowie eine Ausstellung der künstlerischen Arbeiten geplant. Kooperationspartner bei diesem Projekt ist der in Heidelberg ansässige „Kalamari Klub – Freiraum für analoge Fotografie“.
Der 1991 in Bamberg geborene Philip Kanwischer hat Fotografie an der Hochschule für Grafik und Buchkunst in Leipzig und an der Accademia di Belle Arti in Neapel (Italien) studiert. In seinen Arbeiten verknüpft er Bild und Textmaterial zu narrativen Raumsituationen und thematisiert dabei unter anderem die Wahrnehmung von Katastrophen sowie das Spannungsverhältnis zwischen Mensch und Natur. Seine Werke wurden in zahlreichen Einzel- und Gruppenausstellungen gezeigt. Er lebt und arbeitet in Leipzig.
Im Rahmen des Artist Talk wird Philip Kanwischer im Café Leitstelle, Emil-Maier-Straße 16, über seine künstlerische Arbeit Auskunft geben, im Anschluss daran folgt eine Diskussion mit Wissenschaftlern und Publikum. Für den Besuch der Veranstaltung vor Ort gilt die 2G-Regel (geimpft oder genesen mit Maskenpflicht). Eine Registrierung ist unter info@kalamariklub.org notwendig, das gilt auch für die Teilnahme am Livestream.
Kontakt
Dennis Schulz
SFB1225 ISOQUANT
Wissenschaftskommunikation
Philosophenweg 12
69120 Heidelberg
Tel.: +49 6221- 54 5058
Email: d.schulz@thphys.uni-heidelberg.de
Abstract:
Recent results on the analysis of event-by-event net-baryon number fluctuations in Pb–Pb collisions at sNN−−−√=2.76 and 5.02 TeV are presented. The cumulants of the net-proton distributions, proxies for the net-baryon distributions, up to third order are discussed. The experimental results are compared with HIJING and EPOS model calculations and the dependence of fluctuation measurements on the phase-space coverage is addressed in the context of calculations from Lattice QCD (LQCD) and the Hadron Resonance Gas (HRG) model.
M. Arslandok [ALICE Collaboration], “Recent results on conserved charge fluctuations in ALICE”, Nucl. Phys. A 1005, 121979 (2021).
https://www.sciencedirect.com/science/article/abs/pii/S037594742030289X?via%3Dihub
Related to Project A01, C05
Abstract:
One of the ultimate goals of nuclear collision experiments at high energy is to map the phase diagram of strongly interacting matter. A very challenging task is the determination of the QCD phase structure including the search for critical behavior and verification of the possible existence of a critical end point of a first order phase transition line. A promising tool to probe the presence of critical behavior is the study of fluctuations and correlations of conserved charges since, in a thermal system, these fluctuations are directly related to the equation of state (EoS) of the system under the study. In this report an overview is given of several experimental measurements on net-proton multiplicity distributions such as cumulants and multi-particle correlation functions.
https://www.sciencedirect.com/science/article/abs/pii/S0375947420301688?via%3Dihub
Related to Project: A01
Abstract:
The Pauli exclusion principle is the fundamental law underpinning the structure of matter. Due to their anti-symmetric wave function no two fermions can occupy the same quantum state. It has been predicted that this leads to the formation of striking geometric structures, termed Pauli Crystals, when confining fermions in some potential. In contrast to true crystalline phases, these unique high-order density correlations emerge even without any interactions present. Here, we report the direct observation of Pauli Crystals in a system of ultracold atoms, confined to a two-dimensional harmonic oscillator potential. The measurement relies on a single-atom sensitive imaging scheme in momentum space. Our work lays the foundation for future studies of correlations in strongly interacting systems of many fermions.
M. Holten, L. Bayha, K. Subramanian, C. Heintze, P. M. Preiss and S. Jochim, “Observation of Pauli Crystals”, Phys. Rev. Lett. 126, 020401 (2021)
https://journals.aps.org/prl/abstract/10.1103/PhysRevLett.126.020401
Related to Project: C01, C02
Abstract:
n Penning traps electromagnetic forces are used to confine charged particles under well-controlled conditions for virtually unlimited time. Sensitive detection methods have been developed to allow observation of single stored ions. Various cooling methods can be employed to reduce the energy of the trapped particle to nearly at rest. In this review we summarize how highly charged ions (HCIs) offer unique possibilities for precision measurements in Penning traps. Precision atomic and nuclear masses as well as magnetic moments of bound electrons allow among others to determine fundamental constants like the mass of the electron or to perform stringent tests of fundamental interactions like bound-state quantum electrodynamics. Recent results and future perspectives in high-precision Penning-trap spectroscopy with HCIs will be discussed.
K. Blaum, S. Eliseev, and S. Sturm, “Perspectives on testing fundamental physics with highly charged ions in Penning traps”, Quantum Sci. Technol. 6, 014002 (2021).
https://arxiv.org/abs/2102.06692
Related to Project B01, B02
Abstract:
Whether it be physical, biological or social processes, complex systems exhibit dynamics that are exceedingly difficult to understand or predict from underlying principles. Here we report a striking correspondence between the excitation dynamics of a laser driven gas of Rydberg atoms and the spreading of diseases, which in turn opens up a controllable platform for studying non-equilibrium dynamics on complex networks. The competition between facilitated excitation and spontaneous decay results in sub-exponential growth of the excitation number, which is empirically observed in real epidemics. Based on this we develop a quantitative microscopic susceptible-infected-susceptible model which links the growth and final excitation density to the dynamics of an emergent heterogeneous network and rare active region effects associated to an extended Griffiths phase. This provides physical insights into the nature of non-equilibrium criticality in driven many-body systems and the mechanisms leading to non-universal power-laws in the dynamics of complex systems.
T. M. Wintermantel, M. Buchhold, S. Shevate, M. Morgado, Y. Wang, G. Lochead, S. Diehl, S. Whitlock, “Epidemic growth and Griffiths effects on an emergent network of excited
atoms”, Nature Communications 12 (2021).
https://www.nature.com/articles/s41467-020-20333-7
Related to Project A05
Abstract:
We devise an iterative scheme for numerically calculating dynamical two-point correlation functions in integrable many-body systems, in the Eulerian scaling limit. Expressions for these were originally derived in Ref. [1] by combining the fluctuation-dissipation principle with generalized hydrodynamics. Crucially, the scheme is able to address non-stationarity, inhomogeneous situations, when motion occurs at the Euler scale of hydrodynamics. Using our scheme, we study the spreading of correlations in several integrable models from inhomogeneous initial states. For the classical hard rod model we compare our results with Monte-Carlo simulations and observe excellent agreement at long time scales, thus providing the first demonstration of validity for the expressions derived in Ref. [1]. We also observe the onset of the Euler-scale limit for the dynamical correlations.
F. S. Møller, G. Perfetto, B. Doyon, J. Schmiedmayer, “Euler-scale dynamical correlations in integrable systems with fluid motion”, SciPost Phys. Core 3, 16 (2020).
https://scipost.org/10.21468/SciPostPhysCore.3.2.016
Related to Project A03
Abstract:
Impurities in a Fermi sea, or Fermi polarons, experience a Casimir interaction induced by quantum fluctuations of the medium. When there is short-range attraction between impurities and fermions, also the induced interaction between two impurities is strongly attractive at short distance and oscillates in space for larger distances. We theoretically investigate the scattering properties and compute the scattering phase shifts and scattering lengths between two heavy impurities in an ideal Fermi gas at zero temperature. While the induced interaction between impurities is weakly attractive for weak impurity-medium interactions, we find that impurities strongly and attractively interacting with the medium exhibit resonances in the induced scattering with a sign change of the induced scattering length and even strong repulsion. These resonances occur whenever a three-body Efimov bound state appears at the continuum threshold. At energies above the continuum threshold, we find that the Efimov state in medium can turn into a quasi-bound state with a finite decay width.
T. Enss, B. Tran, M. Rautenberg, M. Gerken, E. Lippi, M. Drescher, B. Zhu, M. Weidemüller, and M. Salmhofer, “Scattering of two heavy Fermi polarons: resonances and quasi-bound states”, Phys. Rev. A 102, 063321 (2020).
https://journals.aps.org/pra/abstract/10.1103/PhysRevA.102.063321
Related to Project C03, C02
Abstract:
The nature and location of the QCD phase transition close to the chiral limit restricts the phase structure of QCD with physical pion masses at non-vanishing density. At small pion masses, explicit U(1)A-breaking, as induced by a non-trivial topological density, is of eminent importance. It triggers the ‘t Hooft interactions and also manifests itself in the interplay of four-quark interactions at low momentum scales. In the present work, we perform a Fierz-complete analysis of the emergence of four-quark interactions from the QCD dynamics at finite temperature, subject to a given ‘t Hooft coupling at large momentum scales. The variation of the latter allows us to test the robustness of our findings. Taking an estimate of the effect of the topological running of the ‘t Hooft coupling into account, our analysis suggests that the chiral transition in QCD with two massless quark flavours falls into the O(4) universality class.
J. Braun, M. Leonhardt, J. M. Pawlowski, D. Rosenblüh, “Chiral and effective U(1)A symmetry restoration in ”, Dec. 11, 2020, arXiv:2012.06231 (2020).
https://arxiv.org/abs/2012.06231
Related to Project A02, B03
Press Release No. 114/2020, 11 December 2020
Phase transitions describe dramatic changes in properties of a macroscopic system – like the transition from a liquid to a gas. Starting from individual ultracold atoms, Heidelberg University physicists were able to observe the emergence of such a transition with an increasing number of particles. The research work was carried out in the field of quantum physics under the direction of Prof. Dr Selim Jochim from the Institute for Physics.
In order to formulate effective theories in physics, microscopic details are set aside in favour of macroscopically observable quantities. A cup of water can be described by properties like pressure, temperature and density of the fluid, whereas the position and velocity of the individual water molecules are irrelevant. A phase transition describes the change of a macroscopic system from one state of matter, like fluid, to a different state of matter, like gaseous. The properties of macroscopic systems – so-called many-body systems – can be described as emergent because they result from the interaction of individual components which themselves do not possess these properties.
I have long been interested in how this dramatic macroscopic change at a phase transition emerges from the microscopic description,” states Selim Jochim. To answer this question, the researchers designed an experiment in which they assembled a system from individual ultracold atoms. Using this quantum simulator, they investigated how collective behaviour arises in a microscopic system. To this end, they trapped up to twelve atoms in a tightly focused laser beam. In this artificial system it is possible to continuously tune the interaction strength between the atoms from non-interacting to being the largest energy scale in the system. “On the one hand, the number of particles in the system is small enough to describe the system microscopically. On the other hand, collective effects are already evident,” explains Luca Bayha, a postdoc in Prof. Jochim’s team.
In their experiment, the Heidelberg physicists configured the quantum simulator such that the atoms attract one another, and if the attraction is strong enough, form pairs. These pairs of atoms are the necessary ingredient for a phase transition to a superfluid – a state in which the particles flow without friction. The current experiments focused on when the pair formation emerges as a function of the interaction strength and the particle number. “The surprising result of our experiment is that only six atoms show all the signatures of a phase transition expected for a many-particle system,” adds Marvin Holten, a doctoral student in Prof. Jochim’s group.
In their work, the researchers have benefitted from the framework of the Cluster of Excellence “STRUCTURES – A Unifying Approach to Emergent Phenomena in the Physical World, Mathematics, and Complex Data” and the Collaborative Research Centre “Isolated Quantum Systems and Universality in Extreme Conditions (ISOQUANT)” of Heidelberg University. Crucial to the success of the experiments was a collaboration with researchers from the universities in Lund (Sweden) and Aarhus (Denmark). The research results were published in “Nature”.
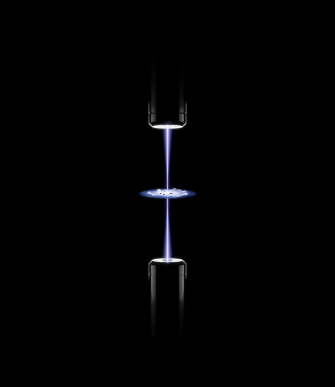
Six pairs of atoms in the focus of a laser beam. © Jonas Ahlstedt / Lund University Bioimaging Centre (LBIC)
Original Publication
L. Bayha, M. Holten, R. Klemt, K. Subramanian, J. Bjerlin, S. M. Reimann, G. M. Bruun, P. M. Preiss, S. Jochim: Observing the emergence of a quantum phase transition shell by shell. Nature 587, 583–587 (2020)
doi:10.1038/s41586-020-2936-y
Contact
Prof. Dr. Selim Jochim
Physikalisches Institut
INF 226, 69120 Heidelberg
Phone: +49 6221 54-19472
jochim@uni-heidelberg.de
Pressemitteilung Nr. 114/2020, 11. Dezember 2020
Phasenübergänge beschreiben dramatische Veränderungen der Eigenschaften eines makroskopischen Systems – zum Beispiel den Wechsel von flüssig zu gasförmig. Ausgehend von einzelnen, ultrakalten Atomen ist es Physikern der Universität Heidelberg in Experimenten gelungen, bei zunehmender Teilchenzahl die Entstehung eines solchen Übergangs zu beobachten. Durchgeführt wurden die Forschungsarbeiten auf dem Gebiet der Quantenphysik unter Leitung von Prof. Dr. Selim Jochim vom Physikalischen Institut.
Um effektive Theorien in der Physik formulieren zu können, werden mikroskopische Details vernachlässigt zugunsten einer Beschreibung makroskopisch beobachtbarer Größen. Der Inhalt einer Tasse Wasser lässt sich etwa durch Eigenschaften wie Druck, Temperatur und Dichte der Flüssigkeit effizient beschreiben; die Position und Geschwindigkeit der einzelnen Wassermoleküle spielen dabei keine Rolle. Ein Phasenübergang beschreibt den Wechsel eines makroskopischen Systems von einem Zustand der Materie, zum Beispiel flüssig, in einen anderen Zustand, zum Beispiel gasförmig. Diese Eigenschaften der makroskopischen Systeme – sogenannte Vielteichensysteme – können als emergent bezeichnet werden: Sie ergeben sich erst aus dem Zusammenwirken der Einzelteile, die selbst diese Eigenschaften nicht aufweisen.
„Seit langem beschäftigt mich die Frage, wie diese dramatische makroskopische Veränderung bei einem Phasenübergang aus der mikroskopischen Beschreibung entsteht“, sagt Selim Jochim. Zur Beantwortung dieser Frage entwarfen die Forscher ein Experiment, bei dem sie ein System aus einzelnen, ultrakalten Atomen zusammengesetzt haben. Mithilfe dieses Quantensimulators untersuchten sie, wie kollektives Verhalten in einem mikroskopischen System entsteht. Zu diesem Zweck wurden bis zu zwölf Atome in einem stark fokussierten Laserstrahl eingefangen. In diesem künstlichen System ist es möglich, die Stärke der Wechselwirkung zwischen den Atomen kontinuierlich über einen so großen Bereich zu verstellen, dass sie entweder komplett zu vernachlässigen oder die größte Energieskala im System ist. „Die Anzahl der Teilchen ist einerseits klein genug für eine mikroskopische Beschreibung des Systems. Andererseits zeigen sich hier schon kollektive Effekte“, erläutert Luca Bayha, Postdoktorand im Team von Prof. Jochim.
Die Heidelberger Physiker haben in ihrem Experiment den Quantensimulator so konfiguriert, dass sich die Atome gegenseitig anziehen und bei starker Anziehung Paare bilden. Diese Atom-Paare sind der notwendige Bestandteil für einen Phasenübergang zu einer Supraflüssigkeit – ein Zustand, bei dem Teilchen ohne Reibung strömen. Die Frage, wann es zu dieser Paarbildung in Abhängigkeit von der Stärke der Wechselwirkung sowie der Anzahl der Teilchen kommt, stand im Mittelpunkt der Untersuchungen. „Das überraschende Ergebnis unseres Experimentes ist, dass sich bereits mit sechs Atomen alle Anzeichen des Phasenübergangs beobachten lassen, die für ein Vielteilchen-System erwartet werden“, so Marvin Holten, Doktorand in der Gruppe von Selim Jochim.
Profitiert haben die Forscher bei ihren Arbeiten vom Exzellenzcluster „STRUCTURES – A Unifying Approach to Emergent Phenomena in the Physical World, Mathematics, and Complex Data“ und dem Sonderforschungsbereich „ Isolierte Quantensysteme und Universalität unter extremen Bedingungen (ISOQUANT)“ der Universität Heidelberg. Entscheidend für das Gelingen der Experimente war die Zusammenarbeit mit Wissenschaftlern von den Universitäten in Lund (Schweden) und Aarhus (Dänemark). Die Forschungsergebnisse wurden in „Nature“ veröffentlicht.
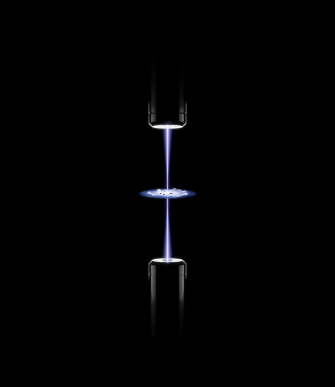
Six pairs of atoms in the focus of a laser beam. © Jonas Ahlstedt / Lund University Bioimaging Centre (LBIC)
Originalpublikation
L. Bayha, M. Holten, R. Klemt, K. Subramanian, J. Bjerlin, S. M. Reimann, G. M. Bruun, P. M. Preiss, S. Jochim: Observing the emergence of a quantum phase transition shell by shell. Nature 587, 583–587 (2020)
doi:10.1038/s41586-020-2936-y
Kontakt
Prof. Dr. Selim Jochim
Physikalisches Institut
INF 226, 69120 Heidelberg
Tel.: +49 6221 54-19472
jochim@uni-heidelberg.de
Press Release No. 114/2020, 11 December 2020
Phase transitions describe dramatic changes in properties of a macroscopic system – like the transition from a liquid to a gas. Starting from individual ultracold atoms, Heidelberg University physicists were able to observe the emergence of such a transition with an increasing number of particles. The research work was carried out in the field of quantum physics under the direction of Prof. Dr Selim Jochim from the Institute for Physics.
In order to formulate effective theories in physics, microscopic details are set aside in favour of macroscopically observable quantities. A cup of water can be described by properties like pressure, temperature and density of the fluid, whereas the position and velocity of the individual water molecules are irrelevant. A phase transition describes the change of a macroscopic system from one state of matter, like fluid, to a different state of matter, like gaseous. The properties of macroscopic systems – so-called many-body systems – can be described as emergent because they result from the interaction of individual components which themselves do not possess these properties.
I have long been interested in how this dramatic macroscopic change at a phase transition emerges from the microscopic description,” states Selim Jochim. To answer this question, the researchers designed an experiment in which they assembled a system from individual ultracold atoms. Using this quantum simulator, they investigated how collective behaviour arises in a microscopic system. To this end, they trapped up to twelve atoms in a tightly focused laser beam. In this artificial system it is possible to continuously tune the interaction strength between the atoms from non-interacting to being the largest energy scale in the system. “On the one hand, the number of particles in the system is small enough to describe the system microscopically. On the other hand, collective effects are already evident,” explains Luca Bayha, a postdoc in Prof. Jochim’s team.
In their experiment, the Heidelberg physicists configured the quantum simulator such that the atoms attract one another, and if the attraction is strong enough, form pairs. These pairs of atoms are the necessary ingredient for a phase transition to a superfluid – a state in which the particles flow without friction. The current experiments focused on when the pair formation emerges as a function of the interaction strength and the particle number. “The surprising result of our experiment is that only six atoms show all the signatures of a phase transition expected for a many-particle system,” adds Marvin Holten, a doctoral student in Prof. Jochim’s group.
In their work, the researchers have benefitted from the framework of the Cluster of Excellence “STRUCTURES – A Unifying Approach to Emergent Phenomena in the Physical World, Mathematics, and Complex Data” and the Collaborative Research Centre “Isolated Quantum Systems and Universality in Extreme Conditions (ISOQUANT)” of Heidelberg University. Crucial to the success of the experiments was a collaboration with researchers from the universities in Lund (Sweden) and Aarhus (Denmark). The research results were published in “Nature”.
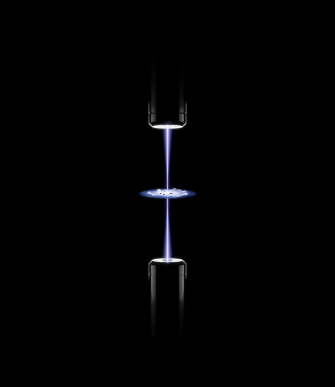
Six pairs of atoms in the focus of a laser beam. © Jonas Ahlstedt / Lund University Bioimaging Centre (LBIC)
Original Publication
L. Bayha, M. Holten, R. Klemt, K. Subramanian, J. Bjerlin, S. M. Reimann, G. M. Bruun, P. M. Preiss, S. Jochim: Observing the emergence of a quantum phase transition shell by shell. Nature 587, 583–587 (2020)
doi:10.1038/s41586-020-2936-y
Contact
Prof. Dr. Selim Jochim
Physikalisches Institut
INF 226, 69120 Heidelberg
Phone: +49 6221 54-19472
jochim@uni-heidelberg.de
Abstract:
Persistent homology provides a robust methodology to infer topological structures from point cloud data. Here we explore the persistent homology of point clouds embedded into a probabilistic setting, exploiting the theory of point processes. We provide variants of notions of ergodicity and investigate measures on the space of persistence diagrams. In particular we introduce the notion of self-similar scaling of persistence diagram expectation measures and prove a packing relation for the occurring dynamical scaling exponents. As a byproduct we generalize the strong law of large numbers for persistent Betti numbers proven in [Hiraoka et al., Ann. Appl. Probab. 28(5), 2018] for averages over cubes to arbitrary convex averaging sequences.
D. Spitz, A. Wienhard, “The self-similar evolution of stationary point processes via persistent homology”, Dec. 10, 2020, arXiv:2012.05751 (2020).
https://arxiv.org/abs/2012.05751
Related to Project B03
Abstract:
We develop a non-perturbative functional framework for computing real-time correlation functionsin strongly correlated systems. The framework is based on the spectral representation of correlationfunctions and dimensional regularisation. Therefore, the non-perturbative spectral renormalisationsetup here respects all symmetries of the theories at hand. In particular this includes space-timesymmetries as well as internal symmetries such as chiral symmetry, and gauge symmetries. Spectralrenormalisation can be applied within general functional approaches such as the functional renor-malisation group, Dyson-Schwinger equations, and two- orn-particle irreducible approaches. As anapplication we compute the full, non-perturbative, spectral function of the scalar field in theφ4-theory in 2+1 dimensions from spectral Dyson-Schwinger equations. We also compute thes-channelspectral function of the fullφ4-vertex in this theory.
J. Horak and J. M. Pawlowski, N. Wink, “Spectral functions in the 4-theory from the spectral Dyson-Schwinger equations”, Phys. Rev. D 102, 125016 (2020).
https://journals.aps.org/prd/abstract/10.1103/PhysRevD.102.125016
Related to Project A02, B03
Abstract:
Four models for the initial conditions of a fluid dynamic description of high energy heavy ion collisions are analysed and compared. We study expectation values and event-by-event fluctuations in the initial transverse energy density profiles from Pb-Pb collisions. Specifically, introducing a Fourier-Bessel mode expansion for fluctuations, we determine expectation values and two-mode correlation functions of the expansion coefficients. The analytically solveable independent point-sources model is compared to an initial state model based on Glauber theory and two models based on the Color Glass Condensate framework. We also discuss to which extent general properties of initial conditions can be understood analytically.
S. Floerchinger, E. Grossi, and K. V. Yousefnia, “Model comparison for initial density fluctuations in high energy heavy ion collisions”, Phys. Rev. C 102, 054914 (2020).
https://journals.aps.org/prc/abstract/10.1103/PhysRevC.102.054914
Related to Project: C06
Abstract:
Holographic duality provides a description of strongly coupled quantum systems in terms of weakly coupled gravitational theories in a higher-dimensional space. It is a challenge, however, to quantitatively determine the physical parameters of the quantum systems corresponding to generic holographic theories. Here, we address this problem for the two-dimensional holographic superfluid, known to exhibit strong dissipation. We numerically simulate the motion of a vortex dipole and perform a high-precision matching of the corresponding dynamics resulting from the dissipative Gross-Pitaevskii equation. Excellent agreement is found for the vortex core shape and the spatiotemporal trajectories. A further comparison to the Hall-Vinen-Iordanskii equations for point vortices interacting with the superfluid allows us to determine the friction parameters of the holographic superfluid. Our results suggest that holographic vortex dynamics can be applied to experimentally accessible superfluids like strongly coupled ultracold Bose gases or thin helium films with temperatures in the Kelvin range. This would make holographic far-from-equilibrium dynamics and turbulence amenable to experimental tests.
P. Wittmer, C.-M. Schmied, T. Gasenzer, C. Ewerz, “Vortex motion quantifies strong dissipation in a holographic superfluid”, Phys. Rev. Lett. 127, 101601 (2021).
https://journals.aps.org/prl/abstract/10.1103/PhysRevLett.127.101601
Related to Project A04
Abstract:
Many-body physics describes phenomena which cannot be understood looking at a systems’ constituents alone. Striking manifestations are broken symmetry, phase transitions, and collective excitations. Understanding how such collective behaviour emerges when assembling a system from individual particles has been a vision in atomic, nuclear, and solid-state physics for decades. Here, we observe the few-body precursor of a quantum phase transition from a normal to a superfluid phase. The transition is signalled by the softening of the mode associated with amplitude vibrations of the order parameter, commonly referred to as a Higgs mode. We achieve exquisite control over ultracold fermions confined to two-dimensional harmonic potentials and prepare closed-shell configurations of 2, 6 and 12 fermionic atoms in the ground state with high fidelity. Spectroscopy is then performed on our mesoscopic system while tuning the pair energy from zero to being larger than the shell spacing. Using full atom counting statistics, we find the lowest resonance to consist of coherently excited pairs only. The distinct non-monotonic interaction dependence of this many-body excitation as well as comparison with numerical calculations allows us to identify it as the precursor of the Higgs mode. Our atomic simulator opens new pathways to systematically unravel the emergence of collective phenomena and the thermodynamic limit particle by particle.
L. Bayha, M. Holten, R. Klemt, K. Subramanian, J. Bjerlin, S. M. Reimann, G. M. Bruun, P. M. Preiss, and S. Jochim, “Observing the emergence of a quantum phase transition – shell by shell”, Nature 587, 583 -587 (2020)
https://www.nature.com/articles/s41586-020-2936-y
Related to Project C01, C02
Abstract:
The modern description of elementary particles is built on gauge theories. Such theories implement fundamental laws of physics by local symmetry constraints, such as Gauss’s law in the interplay of charged matter and electromagnetic fields. Solving gauge theories by classical computers is an extremely arduous task, which has stimulated a vigorous effort to simulate gauge-theory dynamics in microscopically engineered quantum devices. Previous achievements used mappings onto effective models to integrate out either matter or electric fields, or were limited to very small systems. The essential gauge symmetry has not been observed experimentally. Here, we report the quantum simulation of an extended U(1) lattice gauge theory, and experimentally quantify the gauge invariance in a many-body system of 71 sites. Matter and gauge fields are realized in defect-free arrays of bosonic atoms in an optical superlattice. We demonstrate full tunability of the model parameters and benchmark the matter-gauge interactions by sweeping across a quantum phase transition. Enabled by high-fidelity manipulation techniques, we measure Gauss’s law by extracting probabilities of locally gauge-invariant states from correlated atom occupations. Our work provides a way to explore gauge symmetry in the interplay of fundamental particles using controllable large-scale quantum simulators.
B. Yang, H. Sun, R. Ott, H.-Y. Wang, T. V. Zache, J. C. Halimeh, Z.-S. Yuan, P. Hauke, and J.-W. Pan, “Observation of gauge invariance in a 71-site Bose–Hubbard quantum simulator”, Nature 587, 392-396 (2020).
https://www.nature.com/articles/s41586-020-2910-8
Related to Project B04
Abstract:
Thermodynamics is usually developed starting from entropy and the maximum entropy principle. We investigate here to what extent one can replace entropy with relative entropy which has several advantages, for example in the context of local quantum field theory. We find that the principle of maximum entropy can be replaced by a principle of minimum expected relative entropy. Various ensembles and their thermodynamic potentials can be defined through relative entropy. We also show that thermal fluctuations are in fact governed by a relative entropy. Furthermore we reformulate the third law of thermodynamics using relative entropy only.
S. Floerchinger and T. Haas, “Thermodynamics from relative entropy”, Phys. Rev. E 102, 052117 (2020).
https://journals.aps.org/pre/abstract/10.1103/PhysRevE.102.052117
Related to Project C06, A06*
We explore artificial neural networks as a tool for the reconstruction of spectral functions from imaginary time Green’s functions, a classic ill-conditioned inverse problem. Our ansatz is based on a supervised learning framework in which prior knowledge is encoded in the training data and the inverse transformation manifold is explicitly parametrized through a neural network. We systematically investigate this novel reconstruction approach, providing a detailed analysis of its performance on physically motivated mock data, and compare it to established methods of Bayesian inference. The reconstruction accuracy is found to be at least comparable and potentially superior in particular at larger noise levels. We argue that the use of labeled training data in a supervised setting and the freedom in defining an optimization objective are inherent advantages of the present approach and may lead to significant improvements over state-of-the-art methods in the future. Potential directions for further research are discussed in detail.
L. Kades, J. M. Pawlowski, A. Rothkopf, M. Scherzer, J. M. Urban, S. Wetzel, N. Wink, and F. Ziegler, “Spectral reconstruction with deep neural networks”, Phys. Rev. D 102, 096001 (2020).
https://journals.aps.org/prd/abstract/10.1103/PhysRevD.102.096001
Related to Project A02, B03, C05, C06
Abstract:
This work attempts a fundamental formulation of physics based on probabilities. The basic assumptions are simple: One world exists. Humans can understand its properties by formulating laws based on probabilities. Our probabilistic setting only employs the notions of a probability distribution, observables and their expectation values, which are computed according to the classical statistical rule. Time is an ordering structure among observables. Understanding the probabilistic laws enables humans to make predictions for future events. Also space, spacetime and geometry emerge as structures among observables.
Within the classical statistical system the time structure induces the concepts of wave functions, density matrices, non-commuting operators and many other aspects of quantum physics. The classical density matrix encodes the probabilistic information of a time-local subsystem. Subsystems are typically correlated with their environment, offering a much richer structure than discussed commonly. We pay particular attention to subsystems with incomplete statistics and probabilistic observables.
Quantum systems are particular time-local subsystems that follow an unitary evolution law. All laws of quantum mechanics are derived from the basic law for expectation values in classical statistics. In particular, we discuss entangled quantum systems in terms of classical probability distributions. In our approach quantum field theories have to be described by an overall probability distribution for the whole Universe for all times. The fundamental functional integral for quantum field theories should define a probability distribution, underlying the functional integral with Minkowski signature.
While this work remains in the context of theoretical physics, the concepts developed here apply to a wide area of science.
C. Wetterich, “The probabilistic world”, Nov. 4, 2020, arXiv:2011.02867 (2020).
https://arxiv.org/abs/2011.02867
Related to Project
Abstract:
The second law of thermodynamics is discussed and reformulated from a quantum information theoretic perspective for open quantum systems using relative entropy. Specifically, the relative entropy of a quantum state with respect to equilibrium states is considered and its monotonicity property with respect to an open quantum system evolution is used to obtain second law-like inequalities. We discuss this first for generic quantum systems in contact with a thermal bath and subsequently turn to a formulation suitable for the description of local dynamics in a relativistic quantum field theory. A local version of the second law similar to the one used in relativistic fluid dynamics can be formulated with relative entropy or even relative entanglement entropy in a space-time region bounded by two light cones. We also give an outlook towards isolated quantum field theories and discuss the role of entanglement for relativistic fluid dynamics.
N. Dowling, S. Floerchinger, and T. Haas, “Second law of thermodynamics for relativistic fluids formulated with relative entropy”, Phys. Rev. D 102, 105002 (2020).
https://journals.aps.org/prd/abstract/10.1103/PhysRevD.102.105002
Related to Project A06, C06
Abstract:
This report summarizes the presentations and discussions during the Rapid Reaction Task Force “Dynamics of critical fluctuations: Theory — phenomenology — heavy-ion collisions”, which was organized by the ExtreMe Matter Institute EMMI and held at GSI, Darmstadt, Germany in April 2019. We address the current understanding of the dynamics of critical fluctuations in QCD and their measurement in heavy-ion collision experiments. In addition, we outline what might be learned from studying correlations in other physical systems, such as cold atomic gases.
M. Bluhm et al., “Dynamics of critical fluctuations: Theory – phenomenology – heavy-ion collisions”, Nucl. Phys. A 1003 122016 (2020).
https://www.sciencedirect.com/science/article/pii/S0375947420303262?via%3Dihub
Related to Project A02, A01, C05, C06
Abstract:
We explore the relationship between symmetrisation and entanglement through measurements on few-particle systems in a multi-well potential. In particular, considering two or three trapped atoms, we measure and distinguish correlations arising from two different physical origins: antisymmetrisation of the fermionic wavefunction and interaction between particles. We quantify this through the entanglement negativity of states, and the introduction of an antisymmetric negativity, which allows us to understand the role that symmetrisation plays in the measured entanglement properties. We apply this concept both to pure theoretical states and to experimentally reconstructed density matrices of two or three mobile particles in an array of optical tweezers.
J. H. Becher, E. Sindici, R. Klemt, S. Jochim, A. J. Daley, P. M. Preiss, “Measurement of Identical Particle Entanglement and the Influence of Antisymmetrisation”, Phys. Rev. Lett. 125, 180402 (2020)
https://journals.aps.org/prl/abstract/10.1103/PhysRevLett.125.180402
Related to Project C01, C02
We compute the general expression of the one-loop vertex correction in an arbitrary plane-wave background field for the case of two on-shell external electrons and an off-shell external photon. The properties of the vertex corrections under gauge transformations of the plane-wave background field and of the radiation field are studied. Concerning the divergences of the vertex correction, the infrared one is cured by assigning a finite mass to the photon, whereas the ultraviolet one is shown to be renormalized exactly as in vacuum. Finally, the corresponding expression of the vertex correction within the locally constant crossed field approximation is also derived and the high-field asymptotic is shown to scale according to the Ritus-Narozhny conjecture, although the terms featuring the leading-order dependence on the field are found not to contribute to physical transition amplitudes.
A. Di Piazza and M. A. Lopez-Lopez, “One-loop vertex correction in a plane wave”, Phys. Rev. D 102, 076018 (2020).
https://journals.aps.org/prd/abstract/10.1103/PhysRevD.102.076018
Related to Project B02
Abstract:
We study ultra-cold bosons out of equilibrium in a one-dimensional (1D) setting and probe the breaking of integrability and the resulting relaxation at the onset of the crossover from one to three dimensions. In a quantum Newton’s cradle type experiment, we excite the atoms to oscillate and collide in an array of 1D tubes and observe the evolution for up to 4.8 seconds (400 oscillations) with minimal heating and loss. By investigating the dynamics of the longitudinal momentum distribution function and the transverse excitation, we observe and quantify a two-stage relaxation process. In the initial stage single-body dephasing reduces the 1D densities, thus rapidly drives the 1D gas out of the quantum degenerate regime. The momentum distribution function asymptotically approaches the distribution of quasimomenta (rapidities), which are conserved in an integrable system. In the subsequent long time evolution, the 1D gas slowly relaxes towards thermal equilibrium through the collisions with transversely excited atoms. Moreover, we tune the dynamics in the dimensional crossover by initializing the evolution with different imprinted longitudinal momenta (energies). The dynamical evolution towards the relaxed state is quantitatively described by a semiclassical molecular dynamics simulation.
C. Li, T. Zhou, I. Mazets, H.-P. Stimming, F. S. Møller, Z. Zhu, Y. Zhai, W. Xiong, X. Zhou, X. Chen, and J. Schmiedmayer, “Relaxation of Bosons in One Dimension and the Onset of Dimensional Crossover”, SciPost Phys. 9, 58 (2020).
https://scipost.org/SciPostPhys.9.4.058
Related to Project A03
The extraction of the strange quark parton distribution function (PDF) poses a long-standing puzzle. Measurements from neutrino-nucleus deep inelastic scattering (DIS) experiments suggest the strange quark is suppressed compared to the light sea quarks, while recent studies of W±/Z boson production at the LHC imply a larger strange component at small x values. As the parton flavor determination in the proton depends on nuclear corrections, e.g. from heavy-target DIS, LHC heavy ion measurements can provide a distinct perspective to help clarify this situation. In this investigation we extend the nCTEQ15 nPDFs to study the impact of the LHC proton-lead W±/Z production data on both the flavor differentiation and nuclear corrections. This complementary data set provides new insights on both the LHC W±/Z proton analyses and the neutrino-nucleus DIS data. We identify these new nPDFs as nCTEQ15WZ. Our calculations are performed using a new implementation of the nCTEQ code (nCTEQ++) based on C++ which enables us to easily interface to external programs such as HOPPET, APPLgrid and MCFM. Our results indicate that, as suggested by the proton data, the small x nuclear strange sea appears larger than previously expected, even when the normalization of the W±/Z data is accommodated in the fit. Extending the nCTEQ15 analysis to include LHC W±/Z data represents an important step as we advance toward the next generation of nPDFs.
A. Kusina, T. Ježo, D. Clark, P. Duwentäster, E. Godat, T. Hobbs, J. Kent, M. Klasen, K. Kovarík, F. Lyonnet, K. Muzakka, F. Olness, I. Schienbein, and J. Yu, “Impact of LHC vector boson production in heavy ion collisions on strange PDFs”, Eur.Phys.J.C 80 (2020) 10, 968 .
https://link.springer.com/article/10.1140/epjc/s10052-020-08532-4
Related to Project C05
We study the performance of efficient quantum state tomography methods based on neural-network quantum states using measured data from a two-photon experiment. Machine-learning-inspired variational methods provide a promising route towards scalable state characterization for quantum simulators. While the power of these methods has been demonstrated on synthetic data, applications to real experimental data remain scarce. We benchmark and compare several such approaches by applying them to measured data from an experiment producing two-qubit entangled states. We find that in the presence of experimental imperfections and noise, confining the variational manifold to physical states, i.e., to positive semidefinite density matrices, greatly improves the quality of the reconstructed states but renders the learning procedure more demanding. Including additional, possibly unjustified, constraints, such as assuming pure states, facilitates learning, but also biases the estimator.
M. Neugebauer, L. Fischer, A. Jäger, S. Czischek, S. Jochim, M. Weidemüller, and M. Gärttner, “Neural-network quantum state tomography in a two-qubit experiment”, Phys. Rev. A 102, 042604 (2020).
https://journals.aps.org/pra/abstract/10.1103/PhysRevA.102.042604
Related to Project A04, A05
Abstract:
Short autocorrelation times are essential for a reliable error assessment in Monte Carlo simulations of lattice systems. In many interesting scenarios, the decay of autocorrelations in the Markov chain is prohibitively slow. Generative samplers can provide statistically independent field configurations, thereby potentially ameliorating these issues. In this work, the applicability of neural samplers to this problem is investigated. Specifically, we work with a generative adversarial network (GAN). We propose to address difficulties regarding its statistical exactness through the implementation of an overrelaxation step, by searching the latent space of the trained generator network. This procedure can be incorporated into a standard Monte Carlo algorithm, which then permits a sensible assessment of ergodicity and balance based on consistency checks. Numerical results for real, scalar phiv4-theory in two dimensions are presented. We achieve a significant reduction of autocorrelations while accurately reproducing the correct statistics. We discuss possible improvements to the approach as well as potential solutions to persisting issues.
J. M. Urban and J. M. Pawlowski, “Reducing autocorrelation times in lattice simulations with generative adversarial networks”, Machine Learning: Science and Technology 1, 045011 (2020).
https://iopscience.iop.org/article/10.1088/2632-2153/abae73
Related to Project: A01, A02, B03, C01, C02, C03, C05, C06
Press Release Max-Planck-Institut für Kernphysik Heidelberg
08.10.2020
Highest European award in the field of nuclear physics
Klaus Blaum from the MPI for Nuclear Physics is one of the three laureates of the prestigious Lise Meitner Prize 2020 of the European Physical Society. He is awarded it together with Björn Jonson from Chalmers University – member of the scientific advisory board of the MPIK – and Piet van Duppen from KU Leuven “for the development and application of on-line instrumentation and techniques, the precise and systematic investigation of properties of nuclei far from stability, and for shaping the scientific program at the on-line isotope separator facility ISOLDE, CERN.”
The Nuclear Physics Division of the European Physical Society awards the Lise Meitner Prize every two years to one or several individuals from Europe for outstanding work in the fields of experimental, theoretical or applied nuclear science. It represents the highest European distinction in the field of nuclear physics and is connected with a medal showing a portrait of Lise Meitner.
Since 2007, Prof. Dr. Klaus Blaum has been leading the “stored and cooled ions” division as director at the MPI for Nuclear Physics and is a member of the Faculty for Physics and Astronomy of the University of Heidelberg. For his ground-breaking work, he already received numerous prizes and won two times an Advanced Grant of the European Research Council. In 2019, he became a foreign member of the physics class of the Royal Swedish Academy of Sciences.
Contact
Prof. Dr. Klaus Blaum
Phone: +49 6221 516-851
Email: klaus.blaum@mpi-hd.mpg.de
Pressemitteilung Max-Planck-Institut für Kernphysik Heidelberg
08.10.2020
Höchste europäische Auszeichnung auf dem Gebiet der Kernphysik
Klaus Blaum von MPI für Kernphysik ist einer der drei Preisträger des prestigeträchtigen Lise-Meitner-Preises 2020 der Europäischen Physikalischen Gesellschaft. Er erhält ihn zusammen mit Björn Jonson von der Chalmers Universität Göteborg – Mitglied des Fachbeirats des MPIK – und Piet van Duppen von der KU Leuven „für die Entwicklung und Anwendung von on-line Instrumentierung und Techniken, die präzise und systematische Erforschung der Eigenschaften von Kernen weit jenseits der Stabilität und die Gestaltung des wissenschaftlichen Programms am on-line Isotopenseparator ISOLDE des CERN.“
Die Kernphysik-Abteilung der Europäischen Physikalischen Gesellschaft verleiht den Lise-Meitner-Preis alle 2 Jahre an eine oder mehrere Personen aus dem europäischen Raum für herausragende Arbeiten auf den Gebieten der experimentellen, theoretischen oder angewandten Kernphysik. Er ist die höchste europäische Auszeichnung auf dem Gebiet der Kernphysik und mit einer Medaille mit dem Portrait Lise Meitners verbunden.
Prof. Dr. Klaus Blaum leitet seit 2007 als Direktor am MPI für Kernphysik die Abteilung „Gespeicherte und gekühlte Ionen“ und ist Mitglied der Fakultät für Physik und Astronomie der Universität Heidelberg. Für seine bahnbrechenden Arbeiten erhielt er bereits zahlreiche Preise und gewann zweimal einen Advanced Grant des Europäischen Forschungsrats. 2019 wurde er als auswärtiges Mitglied der Physikklasse in die Königlich-Schwedische Akademie der Wissenschaften aufgenommen.
Kontakt
Prof. Dr. Klaus Blaum
Tel: +49 6221 516-851
E-Mail: klaus.blaum@mpi-hd.mpg.de
Abstract:
The smoothing effect of lensing to the CMB temperature power spectrum is, to some extent, degenerate with oscillatory modulations of the primordial power spectrum, in particular if the frequency is close to that of the acoustic peaks. We consider the possibility that the lensing anomaly reported by the latest Planck 2018 results may be hinting at an oscillatory modulation generated by a massive scalar field during an alternative scenario to inflation or by a sharp feature during inflation. We use the full TTTEEE+low E CMB likelihood from Planck to derive constraints on these two types of models. We obtain that in both cases the AL anomaly is mildly reduced to less than 2σ. Moreover, we find that the oscillatory modulation generated during an alternative scenario presents the lowest value of χ2, with Δχ2=−13 compared to ΛCDM. Furthermore, the Akaike Information Criterion suggests that such an oscillation constitutes an attractive candidate since it has a value ΔAIC=−5 with respect to ΛCDM, comparable to the AL parameter. Interestingly, the matter bounce and radiation bounce scenarios are compatible with our results. We discuss how these models of oscillatory features can be tested with future observations.
G. Domènech, X. Chen, M. Kamionkowski, A. Loeb, “Planck residuals anomaly as a fingerprint of alternative scenarios to inflation”, JCAP 10, 005 (2020).
https://iopscience.iop.org/article/10.1088/1475-7516/2020/10/005
Related to Project: B01
Abstract:
The design of quantum many body systems, which have to fulfill an extensive number of constraints, appears as a formidable challenge within the field of quantum simulation. Lattice gauge theories are a particular important class of quantum systems with an extensive number of local constraints and play a central role in high energy physics, condensed matter and quantum information. Whereas recent experimental progress points towards the feasibility of large-scale quantum simulation of Abelian gauge theories, the quantum simulation of non-Abelian gauge theories appears still elusive. In this paper we present minimal non-Abelian lattice gauge theories, whereby we introduce the necessary formalism in well-known Abelian gauge theories, such as the Jaynes-Cumming model. In particular, we show that certain minimal non-Abelian lattice gauge theories can be mapped to three or four level systems, for which the design of a quantum simulator is standard with current technologies. Further we give an upper bound for the Hilbert space dimension of a one dimensional SU(2) lattice gauge theory, and argue that the implementation with current digital quantum computer appears feasible.
V. Kasper, G. Juzeliunas, M. Lewenstein, F. Jendrzejewski, and E. Zohar, “From the Jaynes-Cummings model to non-Abelian gauge theories: a guided tour for the quantum engineer”, New J. Phys. 22, 103027 (2020).
https://iopscience.iop.org/article/10.1088/1367-2630/abb961
Related to Project B04
Abstract:
Conservation laws in a quantum many-body system play a direct role in its dynamic behavior. Understanding the effect of weakly breaking a conservation law due to coherent and incoherent errors is thus crucial, e.g., in the realization of reliable quantum simulators. In this work, we perform exact numerics and time-dependent perturbation theory to study the dynamics of \textit{symmetry violation} in quantum many-body systems with slight coherent (at strength λ) or incoherent (at strength γ) breaking of their local and global symmetries. We rigorously prove the symmetry violation to be a divergence measure in Hilbert space. Based on this, we show that symmetry breaking generically leads to a crossover in the divergence growth from diffusive behavior at onset times to ballistic or hyperballistic scaling at intermediate times, before diffusion dominates at long times. More precisely, we show that for local errors the leading coherent contribution to the symmetry violation cannot be of order lower than ∝λt2 while its leading-order incoherent counterpart is typically of order ∝γt. This remarkable interplay between unitary and incoherent gauge-breaking scalings is also observed at higher orders in projectors onto symmetry (super)sectors. Due to its occurrence at short times, the diffusive-to-ballistic crossover is expected to be readily accessible in modern ultracold-atom and NISQ-device experiments
J. C. Halimeh and P. Hauke, “Diffusive-to-ballistic crossover of symmetry violation in open many-body systems”, arXiv:2010.00009 (2020).
https://arxiv.org/abs/2010.00009
Related to Project: B04
Abstract:
Determining the phase diagram of systems consisting of smaller subsystems ‘connected’ via a tunable coupling is a challenging task relevant for a variety of physical settings. A general question is whether new phases, not present in the uncoupled limit, may arise. We use machine learning and a suitable quasidistance between different points of the phase diagram to study layered spin models, in which the spin variables constituting each of the uncoupled systems (to which we refer as layers) are coupled to each other via an interlayer coupling. In such systems, in general, composite order parameters involving spins of different layers may emerge as a consequence of the interlayer coupling. We focus on the layered Ising and Ashkin–Teller models as a paradigmatic case study, determining their phase diagram via the application of a machine learning algorithm to the Monte Carlo data. Remarkably our technique is able to correctly characterize all the system phases also in the case of hidden order parameters, i.e. order parameters whose expression in terms of the microscopic configurations would require additional preprocessing of the data fed to the algorithm. We correctly retrieve the three known phases of the Ashkin–Teller model with ferromagnetic couplings, including the phase described by a composite order parameter. For the bilayer and trilayer Ising models the phases we find are only the ferromagnetic and the paramagnetic ones. Within the approach we introduce, owing to the construction of convolutional neural networks, naturally suitable for layered image-like data with arbitrary number of layers, no preprocessing of the Monte Carlo data is needed, also with regard to its spatial structure. The physical meaning of our results is discussed and compared with analytical data, where available. Yet, the method can be used without any a priori knowledge of the phases one seeks to find and can be applied to other models and structures.
W. Rzadkowski, N. Defenu, S. Chiacchiera, A. Trombettoni, and G. Bighin, “Detecting composite
orders in layered models via machine learning”, New J. Phys. 22, 093026 (2020).
https://iopscience.iop.org/article/10.1088/1367-2630/abae44
Related to Project: C02
»Eliud Kipchoge was the first man to run 26.2 miles in less than two hours. Maybe Sycamore is the first quantum computer to outperform any supercomputer.« Was haben der berühmte Marathonläufer Eliud Kipchoge und ein Quantencomputer gemeinsam? Wenn Beatrice Ellerhoff Phänomene der Quantenphysik erklärt, greift sie gern auf Analogien und Bilder zurück, die auch den Nicht-Physiker erreichen. Sitzt die 26-jährige Doktorandin nicht am Schreibtisch, ist sie bei Ausdauerläufen in den Heidelberger Wäldern anzutreffen – oder auch am Klavier bei öffentlichen Jamsessions.
Es ist ein frühlingshafter Nachmittag Anfang Mai – gerade warm genug, um ohne Jacke auszukommen. Beatrice Ellerhoff steht vor der Heidelberger Stadtbücherei, hier, wo regelmäßig die »Fridays for Future«-Demonstrationen starteten, bevor die Corona-Pandemie allen Großveranstaltungen einen Strich durch die Rechnung machte. Sie trägt praktische Jeans, Sneakers und einen Rucksack, der alles enthalten könnte, was ihr wichtig ist: von Laufschuhen über Klaviernoten bis hin zu Fachbüchern über theoretische Physik.
Beatrice teilt ihre Hobbys gerne mit vielen anderen. Mit der Physik schien ihr das zunächst nicht möglich zu sein. Was ist es, das die Quantenphysik für den Laien so schwer begreifbar macht? »Quantenphysikalische Systeme sind hochkomplex und zeigen zum Teil bizarre Eigenschaften, die sich unserem intuitiven Verständnis entziehen«, erklärt Beatrice Ellerhoff. Auch den Physikern geben diese Systeme immer wieder Rätsel auf, so zum Beispiel das Phänomen der Supraleitung, die – auf sehr tiefe Temperaturen heruntergekühlt – Strom mit einem Mal widerstandsfrei fließen lässt.
Vor eineinhalb Jahren dann bot sich Beatrice Ellerhoff die Gelegenheit, mit ihrem Fachgebiet auch Nicht-Physiker anzusprechen. Sie schloss sich einem Blog-Projekt an, das Laien verständlich machen möchte, womit sich ein theoretischer Physiker tagtäglich beschäftigt. Zu diesem Zeitpunkt schrieb sie ihre Masterarbeit über Quantensimulationen. »Ich befand mich kurzzeitig in einer Sinnkrise. Jeden Tag errechnete ich mit meinen Simulationen eine Unmenge an Zahlen.« Aber selbst engsten Freunden oder ihrer Familie habe sie kaum begreifbar machen können, was genau sie berechne und wieso das relevant sei.
Bei einem gemeinsamen Mittagessen hatten ihr Oscar Garcia Montero und Alexander Schuckert, Masterstudenten einer benachbarten Arbeitsgruppe, von dem Blog manybody-physics.com erzählt, den die beiden ein halbes Jahr zuvor aufgesetzt hatten. Beatrice war sofort begeistert und stieg ein. Erste Schreiberfahrungen hatte die gebürtige Saarländerin während eines einjährigen Freiwilligendienstes nach dem Abitur gesammelt: In der »Saarbrücker Zeitung« berichtete sie damals über ihre Erlebnisse im ländlichen Paraguay, wo sie Kinder betreute, die ohne diese Unterstützung auf der Straße hätten arbeiten müssen. Noch heute setzt sie dieses Engagement mit dem Verein »Proyecto Tapé« fort, den sie gemeinsam mit einem Freund gegründet hat und der lokale Hilfsprojekte finanziert.
In ihrem jüngsten Artikel auf manybodyphysics.com stellt Beatrice Ellerhoff das Phänomen des Quantencomputers vor und wählt einen überraschenden Einstieg: den Rekord des Kenianers Eliud Kipchoge, der im Oktober vergangenen Jahres als erster Mensch der Geschichte einen Marathon unter zwei Stunden lief. So wie die Läuferwelt über den Wert dieser Zeit und des Laufs an sich diskutierte – der Marathon hatte quasi unter Laborbedingungen stattgefunden und wurde deshalb offiziell nicht als Weltrekord anerkannt –, so debattierten zeitgleich Physiker auf der ganzen Welt über den Wert und die Leistungsfähigkeit von Quantencomputern. Auslöser, so schreibt die Physikdokto-randin, war die Behauptung des Internetgiganten Google, mit seinem Quantencomputer Sycamore einen komplizierten Rechenprozess in unter vier Minuten lösen zu können, für den der beste derzeit verfügbare Computer mehrere Tausend Jahre brauche. Ganz nebenbei klärt Beatrice Ellerhoff ihre Leser dabei über die Funktionsweise von Quantencomputern auf.
Dass sie in ihrem Artikel auf Kipchoges Rekord zurückgreift, ist kein Zufall. Auch sie ist Langstreckenläuferin und kam beim Heidelberger Halbmarathon im letzten Jahr als Siebte aller teilnehmenden Frauen ins Ziel. Ihre Leidenschaft für den Ausdauersport hat sich – ebenso wie die für Mathematik – schon in früher Kindheit entwickelt. Vorbild war dabei immer der Vater: Informatiker, begeisterter Läufer und nicht zuletzt begabter Musiker. Auch diese Passion hat Beatrice geerbt – und führt sie, nachdem ihr Vater vor sieben Jahren früh verstarb, in seinem Gedenken fort. »Ich fühle mich ihm nahe, wenn ich den Interessen nachgehe, die wir geteilt haben.«
Dass sie Jazzmusik so liebt, hat aber noch andere Gründe: Musik ist für mich der perfekte Ausgleich zur kopflastigen Promotion«, erklärt die Doktorandin, die mit einem Stipendium der Heidelberger Graduiertenschule für Physik am Institut für Umweltphysik zum Thema Klimavariabilität forscht. »Hier muss ich nicht nachdenken, sondern kann es einfach fließen lassen – insbesondere beim Jazz, bei dem viel improvisiert wird.« Nur selten spielt sie alleine, sondern fast immer zusammen mit Freunden – so auch bei den Jamsessions in den Heidelberger Clubs Cave54 und Halle02, von denen Beatrice hofft, dass sie bald wieder stattfinden dürfen.
Die Tage der Physikerin, die sich selbst als »Early Bird« bezeichnet, sind gut strukturiert. Da sind nicht nur Dissertation, Hobbys und soziales Engagement – nebenbei schreibt sie derzeit noch an einer 50-seitigen Einführung zu Quantencomputern für die Serie »Essentials« des Springer-Verlags. Und auch für den Blog ist ein nächster Beitrag in Arbeit. Mit ihren zwei Co-Autoren verfasst Beatrice kurze Porträts, um einen Einblick in den Alltag von Physikerinnen und Physikern zu geben – und dabei nicht zuletzt das Stereotyp des Nerds aufzuheben, das Vertreterinnen und Vertretern ihrer Disziplin noch immer anhängt.
Dieser Artikel ist im UNISPIEGEL 1/2020 (Seite 10) erschienen.
https://www.uni-heidelberg.de/de/presse-medien/publikationen/unispiegel/uni-privat/quantensimulation-fuer-laien
»Eliud Kipchoge was the first man to run 26.2 miles in less than two hours. Maybe Sycamore is the first quantum computer to outperform any supercomputer.« Was haben der berühmte Marathonläufer Eliud Kipchoge und ein Quantencomputer gemeinsam? Wenn Beatrice Ellerhoff Phänomene der Quantenphysik erklärt, greift sie gern auf Analogien und Bilder zurück, die auch den Nicht-Physiker erreichen. Sitzt die 26-jährige Doktorandin nicht am Schreibtisch, ist sie bei Ausdauerläufen in den Heidelberger Wäldern anzutreffen – oder auch am Klavier bei öffentlichen Jamsessions.
Es ist ein frühlingshafter Nachmittag Anfang Mai – gerade warm genug, um ohne Jacke auszukommen. Beatrice Ellerhoff steht vor der Heidelberger Stadtbücherei, hier, wo regelmäßig die »Fridays for Future«-Demonstrationen starteten, bevor die Corona-Pandemie allen Großveranstaltungen einen Strich durch die Rechnung machte. Sie trägt praktische Jeans, Sneakers und einen Rucksack, der alles enthalten könnte, was ihr wichtig ist: von Laufschuhen über Klaviernoten bis hin zu Fachbüchern über theoretische Physik.
Beatrice teilt ihre Hobbys gerne mit vielen anderen. Mit der Physik schien ihr das zunächst nicht möglich zu sein. Was ist es, das die Quantenphysik für den Laien so schwer begreifbar macht? »Quantenphysikalische Systeme sind hochkomplex und zeigen zum Teil bizarre Eigenschaften, die sich unserem intuitiven Verständnis entziehen«, erklärt Beatrice Ellerhoff. Auch den Physikern geben diese Systeme immer wieder Rätsel auf, so zum Beispiel das Phänomen der Supraleitung, die – auf sehr tiefe Temperaturen heruntergekühlt – Strom mit einem Mal widerstandsfrei fließen lässt.
Vor eineinhalb Jahren dann bot sich Beatrice Ellerhoff die Gelegenheit, mit ihrem Fachgebiet auch Nicht-Physiker anzusprechen. Sie schloss sich einem Blog-Projekt an, das Laien verständlich machen möchte, womit sich ein theoretischer Physiker tagtäglich beschäftigt. Zu diesem Zeitpunkt schrieb sie ihre Masterarbeit über Quantensimulationen. »Ich befand mich kurzzeitig in einer Sinnkrise. Jeden Tag errechnete ich mit meinen Simulationen eine Unmenge an Zahlen.« Aber selbst engsten Freunden oder ihrer Familie habe sie kaum begreifbar machen können, was genau sie berechne und wieso das relevant sei.
Bei einem gemeinsamen Mittagessen hatten ihr Oscar Garcia Montero und Alexander Schuckert, Masterstudenten einer benachbarten Arbeitsgruppe, von dem Blog manybody-physics.com erzählt, den die beiden ein halbes Jahr zuvor aufgesetzt hatten. Beatrice war sofort begeistert und stieg ein. Erste Schreiberfahrungen hatte die gebürtige Saarländerin während eines einjährigen Freiwilligendienstes nach dem Abitur gesammelt: In der »Saarbrücker Zeitung« berichtete sie damals über ihre Erlebnisse im ländlichen Paraguay, wo sie Kinder betreute, die ohne diese Unterstützung auf der Straße hätten arbeiten müssen. Noch heute setzt sie dieses Engagement mit dem Verein »Proyecto Tapé« fort, den sie gemeinsam mit einem Freund gegründet hat und der lokale Hilfsprojekte finanziert.
In ihrem jüngsten Artikel auf manybodyphysics.com stellt Beatrice Ellerhoff das Phänomen des Quantencomputers vor und wählt einen überraschenden Einstieg: den Rekord des Kenianers Eliud Kipchoge, der im Oktober vergangenen Jahres als erster Mensch der Geschichte einen Marathon unter zwei Stunden lief. So wie die Läuferwelt über den Wert dieser Zeit und des Laufs an sich diskutierte – der Marathon hatte quasi unter Laborbedingungen stattgefunden und wurde deshalb offiziell nicht als Weltrekord anerkannt –, so debattierten zeitgleich Physiker auf der ganzen Welt über den Wert und die Leistungsfähigkeit von Quantencomputern. Auslöser, so schreibt die Physikdokto-randin, war die Behauptung des Internetgiganten Google, mit seinem Quantencomputer Sycamore einen komplizierten Rechenprozess in unter vier Minuten lösen zu können, für den der beste derzeit verfügbare Computer mehrere Tausend Jahre brauche. Ganz nebenbei klärt Beatrice Ellerhoff ihre Leser dabei über die Funktionsweise von Quantencomputern auf.
Dass sie in ihrem Artikel auf Kipchoges Rekord zurückgreift, ist kein Zufall. Auch sie ist Langstreckenläuferin und kam beim Heidelberger Halbmarathon im letzten Jahr als Siebte aller teilnehmenden Frauen ins Ziel. Ihre Leidenschaft für den Ausdauersport hat sich – ebenso wie die für Mathematik – schon in früher Kindheit entwickelt. Vorbild war dabei immer der Vater: Informatiker, begeisterter Läufer und nicht zuletzt begabter Musiker. Auch diese Passion hat Beatrice geerbt – und führt sie, nachdem ihr Vater vor sieben Jahren früh verstarb, in seinem Gedenken fort. »Ich fühle mich ihm nahe, wenn ich den Interessen nachgehe, die wir geteilt haben.«
Dass sie Jazzmusik so liebt, hat aber noch andere Gründe: Musik ist für mich der perfekte Ausgleich zur kopflastigen Promotion«, erklärt die Doktorandin, die mit einem Stipendium der Heidelberger Graduiertenschule für Physik am Institut für Umweltphysik zum Thema Klimavariabilität forscht. »Hier muss ich nicht nachdenken, sondern kann es einfach fließen lassen – insbesondere beim Jazz, bei dem viel improvisiert wird.« Nur selten spielt sie alleine, sondern fast immer zusammen mit Freunden – so auch bei den Jamsessions in den Heidelberger Clubs Cave54 und Halle02, von denen Beatrice hofft, dass sie bald wieder stattfinden dürfen.
Die Tage der Physikerin, die sich selbst als »Early Bird« bezeichnet, sind gut strukturiert. Da sind nicht nur Dissertation, Hobbys und soziales Engagement – nebenbei schreibt sie derzeit noch an einer 50-seitigen Einführung zu Quantencomputern für die Serie »Essentials« des Springer-Verlags. Und auch für den Blog ist ein nächster Beitrag in Arbeit. Mit ihren zwei Co-Autoren verfasst Beatrice kurze Porträts, um einen Einblick in den Alltag von Physikerinnen und Physikern zu geben – und dabei nicht zuletzt das Stereotyp des Nerds aufzuheben, das Vertreterinnen und Vertretern ihrer Disziplin noch immer anhängt.
Dieser Artikel ist im UNISPIEGEL 1/2020 (Seite 10) erschienen.
https://www.uni-heidelberg.de/de/presse-medien/publikationen/unispiegel/uni-privat/quantensimulation-fuer-laien
Abstract:
A major test of the capabilities of modern quantum simulators and NISQ devices is the reliable realization of gauge theories, which constitute a gold standard of implementational efficacy. In addition to unavoidable unitary errors, realistic experiments suffer from decoherence, which compromises gauge invariance and, therefore, the gauge theory itself. Here, we study the effect of decoherence on the quench dynamics of a lattice gauge theory. Rigorously identifying the gauge violation as a divergence measure in the gauge sectors, we find at short times that it first grows diffusively ∼γt due to decoherence at environment-coupling strength γ, before unitary errors at strength λ dominate and the violation grows ballistically ∼λ2t2. We further introduce multiple quantum coherences in the context of gauge theories to quantify decoherence effects. Both experimentally accessible measures will be of independent interest beyond the immediate context of this work.
J. C. Halimeh, V. Kasper, and P. Hauke, “Fate of Lattice Gauge Theories Under Decoherence”, arXiv:2009.07848 (2020).
https://arxiv.org/abs/2009.07848
Related to Project: B04
Abstract:
A new truncation scheme based on the cumulant expansion of the one-particle phase-space distribution function for dark matter particles is developed. Extending the method of moments in relativistic kinetic theory, we derive evolution equations which supplement the covariant conservation of the energy-momentum tensor and particle number current. Truncating the cumulant expansion we obtain a closed, covariant and hyperbolic system of equations which can be used to model the evolution of a general relativistic nonideal fluid. As a working example we consider a Friedmann-Lemaître-Robertson-Walker cosmology with dynamic pressure and solve for the time evolution of the effective equation of state parameter.
A. Erschfeld, S. Floerchinger, and M. Rupprecht, “General relativistic nonideal fluid equations for dark matter from a truncated cumulant expansion”, Phys. Rev. D 102, 063520 (2020).
https://journals.aps.org/prd/abstract/10.1103/PhysRevD.102.063520
Related to Project: C06
Abstract:
The resonant interaction between x-ray photons and nuclei is one of the most exciting subjects in the burgeoning field of x-ray quantum optics. A resourceful platform used to date is thin-film x-ray cavities with embedded layers or Mössbauer nuclei such as 57Fe. A quantum optical model based on the classical electromagnetic Green’s function is developed to investigate theoretically the nuclear response inside the x-ray cavity. The model is versatile and provides an intuitive picture about the influence of the cavity structure on the resulting spectra. We test its predictive powers with the help of the semiclassical coherent scattering formalism simulations and discuss our results on increasing complexity of layer structures.
X. Kong, D. E. Chang, and A. Pálffy, “Green’s-function formalism for resonant interaction of x rays with nuclei in structured media”, Phys. Rev. A 102, 033710 (2020).
https://journals.aps.org/pra/abstract/10.1103/PhysRevA.102.033710
Related to Project: B02
Abstract:
The Berezinskii-Kosterlitz-Thouless (BKT) mechanism describes universal vortex unbinding in many two-dimensional systems, including the paradigmatic XY model. However, most of these systems present a complex interplay between excitations at different length scales that complicates theoretical calculations of nonuniversal thermodynamic quantities. These difficulties may be overcome by suitably modifying the initial conditions of the BKT flow equations to account for noncritical fluctuations at small length scales. In this work, we perform a systematic study of the validity and limits of this two-step approach by constructing optimised initial conditions for the BKT flow. We find that the two-step approach can accurately reproduce the results of Monte Carlo simulations of the traditional XY model. To systematically study the interplay between vortices and spin-wave excitations, we introduce a modified XY model with increased vortex fugacity. We present large-scale Monte Carlo simulations of the spin stiffness and vortex density for this modified XY model and show that even at large vortex fugacity, vortex unbinding is accurately described by the nonperturbative functional renormalization group.
I. Maccari, N. Defenu, L. Benfatto, C. Castellani, and T. Enss, “Interplay of spin waves and vortices in the two-dimensional xy model at small vortex-core energy”, Phys. Rev. B 102, 104505 (2020).
https://journals.aps.org/prb/abstract/10.1103/PhysRevB.102.104505
Related to Project C02, C03, C01
Abstract:
We calculate chiral susceptibilities in (2+1)-flavour QCD for different masses of the light quarks using the functional renormalisation group (fRG) approach to first-principles QCD. We follow the evolution of the chiral susceptibilities with decreasing masses as obtained from both the light-quark and the reduced quark condensate. The latter compares very well with recent results from the HotQCD collaboration for pion masses mπ≳100MeV. For smaller pion masses, the fRG and lattice results are still consistent. In particular, the estimates for the chiral critical temperature are in very good agreement. We close by discussing different extrapolations to the chiral limit.
J. Braun, W.-j. Fu, J. M. Pawlowski, F. Rennecke, D. Rosenblüh, S. Yin: Chiral Susceptibility in (2+1)-flavour QCD, Phys. Rev. D 102, 056010 (2020).
https://journals.aps.org/prd/abstract/10.1103/PhysRevD.102.056010
Related to project A02, C05, C06
Abstract:
Recent years have seen strong progress in quantum simulation of gauge-theory dynamics using ultracold-atom experiments. A principal challenge in these efforts is the certification of gauge invariance, which has recently been realized in [B.~Yang \textit{et al.}, arXiv:2003.08945]. One major but poorly investigated experimental source of gauge-invariance violation is an imperfect preparation of the initial state. Using the time-dependent density-matrix renormalization group, we analyze the robustness of gauge-invariant dynamics against potential preparation defects in the above ultracold-atom implementation of a U(1) gauge theory. We find defects related to an erroneous initialization of matter fields to be innocuous, as the associated gauge-invariance violation remains strongly localized throughout the time evolution. A defect due to faulty initialization of the gauge field leads to a mild proliferation of the associated violation. Furthermore, we characterize the influence of immobile and mobile defects by monitoring the spread of entanglement entropy. Overall, our results indicate that the aforementioned experimental realization exhibits a high level of fidelity in the gauge invariance of its dynamics at all evolution times. Our work provides strong evidence that ultracold-atom setups can serve as an extremely reliable framework for the quantum simulation of gauge-theory dynamics.
J. C. Halimeh, R. Ott, I. P. McCulloch, B. Yang, and P. Hauke, “Robustness of gauge-invariant dynamics in ultracold-atom gauge theories”, Phys. Rev. Research 2, 033361 (2020).
https://journals.aps.org/prresearch/abstract/10.1103/PhysRevResearch.2.033361
Related to Project: B03, B04
Dr Preiss’s ERC project “UniRand – Random Unitaries in a Rapid Optical Lattice Simulator” involves experiments with ultracold quantum gases in optical lattices. The aim is to realise many-body systems with completely new properties through laser cooling and optical trapping. “The point is that strongly correlated systems, which occur for example in the solid state, can be simulated with single-particle resolution. That way, we can reconstruct the quantum state of a system atom-by-atom,” explains the physicist, who since 2016 has worked as a post-doc at the Institute for Physics and at the Heidelberg Center for Quantum Dynamics of Heidelberg University. The researchers hope that this will enable them to find new access to correlators and invariants in many-body systems, which have been investigated in theory but have so far not been measured in any experiment. Approximately 1.5 million euros in funding are available to Philipp Preiss’s team for the project.
The European Research Council awards the starting grant to outstanding young scientists. Criteria for the funding are the scientific excellence of the early-career researchers and the innovative potential of their research ideas.
[…]
Original Press Release
Presse Release No. 75, 03 Septemeber 2020
https://www.uni-heidelberg.de/en/newsroom/erc-starting-grants-three-heidelberg-scientists
Contact
Dr. Philipp Preiss
Physikalisches Institut (Room 00.404)
Im Neuenheimer Feld 226
69120 Heidelberg
Phone: ++49 6221 54 19486
preiss@physi.uni-heidelberg.de
Dr Preiss’s ERC project “UniRand – Random Unitaries in a Rapid Optical Lattice Simulator” involves experiments with ultracold quantum gases in optical lattices. The aim is to realise many-body systems with completely new properties through laser cooling and optical trapping. “The point is that strongly correlated systems, which occur for example in the solid state, can be simulated with single-particle resolution. That way, we can reconstruct the quantum state of a system atom-by-atom,” explains the physicist, who since 2016 has worked as a post-doc at the Institute for Physics and at the Heidelberg Center for Quantum Dynamics of Heidelberg University. The researchers hope that this will enable them to find new access to correlators and invariants in many-body systems, which have been investigated in theory but have so far not been measured in any experiment. Approximately 1.5 million euros in funding are available to Philipp Preiss’s team for the project.
The European Research Council awards the starting grant to outstanding young scientists. Criteria for the funding are the scientific excellence of the early-career researchers and the innovative potential of their research ideas.
[…]
Original Press Release
Presse Release No. 75, 03 Septemeber 2020
https://www.uni-heidelberg.de/en/newsroom/erc-starting-grants-three-heidelberg-scientists
Contact
Dr. Philipp Preiss
Physikalisches Institut (Room 00.404)
Im Neuenheimer Feld 226
69120 Heidelberg
Phone: ++49 6221 54 19486
preiss@physi.uni-heidelberg.de
Abstract:
The existing transformation from a relativistic real scalar field to a complex non-relativistic scalar field by Namjoo, Guth, and Kaiser is generalized from Minkowski space to a more general background metric. In that case the transformation is not purely algebraic any more but determined by a differential equation. We apply the generalized transformation to a real scalar with ϕ4 interaction on an Friedmann-Lemaître-Robertson-Walker cosmologically expanding background and calculate the resulting non-relativistic action up to second order in small parameters. We also show that the transformation can be interpreted as a Bogoliubov transformation between relativistic and non-relativistic creation and annihilation operators and comment on emerging symmetries in the non-relativistic theory.
L. H. Heyen and S. Floerchinger, “Real scalar field, the nonrelativistic limit, and the cosmological
expansion”, Phys. Rev. D 102, 036024 (2020).
https://journals.aps.org/prd/abstract/10.1103/PhysRevD.102.036024
Related to Project: C06
Abstract:
We introduce a novel real-time formulation of lattice NRQCD designed for simulations in the background of an highly occupied gluon field. By evolving quarks in the background of a dynamically evolving gluon field we computed the time-evolution of heavy-quarkonium spectral functions as well as the static and for finitely heavy quarks generalised potential. We conclude that the back reaction of the quarks is necessary for any binding process. Here we discuss the methodology, our results and the origin of the absence of a binding process.
A. Lehmann, A. Rothkopf, “Real-Time-Evolution of Heavy-Quarkonium Bound States”, PoS LATTICE2019, 074 (2020).
Related to Project C05
Abstract:
Lefschetz thimbles have been proposed recently as a possible solution to the complex action problem (sign problem) in Monte Carlo simulations. Here we discuss pure abelian gauge theory with a complex coupling β and apply the concept of Generalized Lefschetz thimbles. We propose to simulate the theory on the union of the tangential manifolds to the thimbles.
We construct a local Metropolis-type algorithm, that is constrained to a specific tangential manifold. We also discuss how, starting from this result, successive subleading tangential manifolds can be taken into account via a reweighting approach. We demonstrate the algorithm on U(1) gauge theory in 1+1 dimensions and investigate the residual sign problem.
M. Pawlowski, M. Scherzer, C. Schmidt, F. P. G. Ziegler, F. Ziesché: Simulating gauge theories on Lefschetz thimbles, PoS LATTICE2019, 223 (2020).
Related to Project B03, A02
Abstract:
We investigate the measurement of Hanbury Brown–Twiss (HBT) photon correlations as an experimental tool to discriminate different sources of photon production. To showcase that HBT correlations can distinguish between such sources, we consider two different scenarios in which we enhance the yields from standard hydrodynamical simulations. In the first, additional photons are produced from the early preequilibrium stage computed from the “bottom-up” thermalization scenario. In the second, the thermal rates are enhanced close to the pseudocritical temperature Tc≈155MeV using a phenomenological ansatz. We compute the correlators for relative momenta qo, qs, and ql for different transverse pair momenta, K⊥, and find that the longitudinal correlation is the most sensitive to different photon sources. Our results also demonstrate that including anisotropic preequilibrium rates enhances non-Gaussianities in the correlators, which can be quantified using the kurtosis of the correlators. Finally, we study the feasibility of measuring a direct photon HBT signal in the upcoming high-luminosity runs at the CERN Large Hadron Collider. Considering only statistical uncertainties, we find that with the projected ≈1010 heavy-ion events a measurement of the HBT correlations for K⊥<1GeV is statistically significant.
O. Garcia-Montero, N. Löher, A. Mazeliauskas, J. Berges, and K. Reygers, “Probing the evolution of heavy-ion collisions using direct photon interferometry”, Phys. Rev. C 102, 024915 (2020).
https://journals.aps.org/prc/abstract/10.1103/PhysRevC.102.024915
Related to Project: A01, A02, C06
Abstract:
We discuss the QCD phase structure at finite temperature and chemical potential for 2-flavor and 2+1-flavor QCD. The results are achieved by computing QCD correlation functions within a generalized functional approach that combines Dyson-Schwinger equations (DSE) and the functional renormalization group (fRG). In this setup fRG precision data from [A. K. Cyrol, M. Mitter, J. M. Pawlowski, and N. Strodthoff, Phys. Rev. D 97, 054006 (2018).] for the vacuum quark-gluon vertex and gluon propagator of 2-flavor QCD used as input, and the respective DSEs are expanded about this input. While the vacuum results for other correlation functions serve as a self-consistency check for functional approaches, the results at finite temperature and density are computed, for the first time, without the need of phenomenological infrared parameters
F. Gao, Jan M. Pawlowski: QCD phase structure from functional methods, Phys. Rev. D 102, 034027 (2020).
https://journals.aps.org/prd/abstract/10.1103/PhysRevD.102.034027
Related to Project A02, C05, C06
Abstract:
If a grand-unified extension of the asymptotically safe Reuter fixed-point for quantum gravity exists, it determines free parameters of the grand-unified scalar potential. All quartic couplings take their fixed-point values in the trans-Planckian regime. They are irrelevant parameters that are, in principle, computable for a given particle content of the grand unified model. In turn, the direction of spontaneous breaking of the grand-unified gauge symmetry becomes predictable. For the flow of the couplings below the Planck mass, gauge and Yukawa interactions compete for the determination of the minimum of the effective potential.
A. Eichhorn, A. Held, and C. Wetterich, “Predictive power of grand unification from quantum gravity”, J. High Energ. Phys. 2020, 111 (2020).
https://link.springer.com/article/10.1007/JHEP08(2020)111
Related to Project:
Abstract:
We show that ultracold fermions in an artificial magnetic field open up a new window to the physics of the spinful fractional quantum Hall effect. We numerically study the lowest energy states of strongly interacting few-fermion systems in rapidly rotating optical microtraps. We find that skyrmion-like ground states with locally ferromagnetic, long-range spin textures emerge. To realize such states experimentally, rotating microtraps with higher-order angular momentum components may be used to prepare fermionic particles in a lowest Landau level. We find parameter regimes in which skyrmion-like ground states should be accessible in current experiments and demonstrate an adiabatic pathway for their preparation in a rapidly rotating harmonic trap. The addition of long range interactions will lead to an even richer interplay between spin textures and fractional quantum Hall physics.
L. Palm, F. Grusdt, and P. M. Preiss, “Skyrmion Ground States of Rapidly Rotating Few-Fermion Systems”, New J. Phys. 22, 083037 (2020).
https://iopscience.iop.org/article/10.1088/1367-2630/aba30e
Related to Project: C01, C02
Abstract:
The large density of gluons, which is present shortly after a nuclear collision at very high energies, can lead to the formation of a condensate. We identify a gauge-invariant order parameter for condensation based on elementary nonperturbative excitations of the plasma, which are described by spatial Wilson loops. Using real-time lattice simulations, we demonstrate that a self-similar transport process towards low momenta builds up a macroscopic zero mode. Our findings reveal intriguing similarities to recent discoveries of condensation phenomena out of equilibrium in table-top experiments with ultracold Bose gases.
J. Berges, K. Boguslavski, M. Mace, and J. M. Pawlowski, “Gauge-invariant condensation in the nonequilibrium quark-gluon plasma”, Phys. Rev. D 102, 034014 (2020).
https://journals.aps.org/prd/abstract/10.1103/PhysRevD.102.034014
Related to Project: B03, A01, A02
Abstract:
The use of high-precision measurements of the g factor of few-electron ions and its isotope shifts is put forward as a probe for physics beyond the Standard Model. The contribution of a hypothetical fifth fundamental force to the g factor is calculated for the ground state of H-like, Li-like and B-like ions, and employed to derive bounds on the parameters of that force. The weighted difference and especially the isotope shift of g factors are used in order to increase the experimental sensitivity to the new physics contribution. It is found that, combining measurements from four different isotopes of H-like, Li-like and B-like calcium ions at accuracy levels projected to be accessible in the near future, experimental results compatible with King planarity would constrain the new physics coupling constant more than one order of magnitude further than the best current atomic data and theory.
V.Debierre, C.H.Keitel and Z.Harman, “Fifth-force search with the bound-electron g factor”, Phys. Lett. B 807, 135527 (2020).
https://www.sciencedirect.com/science/article/pii/S0370269320303312
Related to Project B02
Abstract:
The scalar perturbation induced gravitational waves are a probe of the primordial density perturbation spectrum on small scales. In this paper, we show that they can also probe the thermal history of the universe. We assume the universe underwent a stage with a constant equation of state parameter w, followed by the radiation-dominated stage of the conventional bigbang universe. We find that the infrared slope of the power spectrum of the induced stochastic gravitational wave background for decelerating cosmologies is related to the equation of state of the universe. Furthermore, the induced gravitational wave spectrum has in general a broken power-law shape around the scale of reheating. Interestingly, below the threshold w=0 of the equation of state parameter, the broken power-law presents a peak for a dirac delta peak in the scalar spectrum. For a finite width peak, the threshold changes to w=−1/15 depending on the value of the width. In some cases, such a broken power-law gravitational wave spectrum may degenerate to the spectrum from other sources like phase transitions or global cosmic strings.
G. Domènech, S. Pi, and M. Sasaki, “Induced gravitational waves as a probe of thermal history of the universe”, JCAP 08 (2020) 017.
https://iopscience.iop.org/article/10.1088/1475-7516/2020/08/017
Related to Project: B01
Wien (APA) – Er ist eines der Aushängeschilder der österreichischen Quantenphysik: Der Experimentalphysiker Jörg Schmiedmayer, Professor für Quantenphysik an der Technischen Universität (TU) Wien. Sein Forschungsschwerpunkt liegt auf der Entwicklung von Atom-Chips zur kontrollierten Manipulation ultrakalter Atome, was ihm den Beinamen “Mr. Atomchip” eingetragen hat. Am Donnerstag (13.8.) wird er 60 Jahre alt.
Schmiedmayer, geboren am 13. August 1960 in Wien, studierte Physik an der TU Wien und Astronomie an der Uni Wien. Nach einem Zwischenstopp bei der Europäischen Organisation für Kernforschung CERN wurde er 1987 an der TU als Schüler von Helmut Rauch in Physik promoviert. Seine Post-Doc-Jahre absolvierte er, unterstützt von einem Schrödinger-Stipendium, an der Harvard University und am Massachusetts Institute for Technology (MIT), ehe er 1993 zu Anton Zeilinger ans Institut für Experimentalphysik der Uni Innsbruck wechselte. Dort habilitierte er sich 1997 für Experimentalphysik. 2000 nahm er einen Ruf an die Universität Heidelberg an, wo er eine Atomchip-Arbeitsgruppe aufbaute.
Rückholaktion von Siemens, Stadt Wien und TU Wien
Es bedurfte einiger Anstrengungen, Schmiedmayer 2005 wieder nach Österreich zurückzuholen. Für die “Rückholaktion” stellte Siemens anlässlich seines 125-jährigen Bestehens eine Mio. Euro bereit, die Stadt Wien zog mit der gleichen Summe mit. Die TU Wien, wo er eine seit Jahren vakante Professorenstelle für Experimentalphysik erhielt, stellte 1,6 Mio. Euro für den Umbau der Laboratorien und den notwendige Renovierungsarbeiten am Gebäude des Atominstituts zur Verfügung, wo der Physiker 2006 mit seiner Gruppe einzog.
Fix nach Wien übersiedelte Schmiedmayer erst, als sein neues Labor fix und fertig war und er seine Arbeit nahtlos fortsetzen konnte. “Wissenschaft ist wie ein Termingeschäft am Ölmarkt. Wenn man nicht der erste ist, kann man es gleich bleiben lassen”, zeigte er damals seinen Zugang zu wissenschaftlicher Arbeit. Wichtig ist ihm bei dieser vor allem die Unabhängigkeit, erfolgreich werde man darin, “indem man Sachen macht, die einem Spaß machen und die noch niemand gemacht hat”.
“Das Beste aus zwei Welten”
Das gelingt dem Physiker in vielen Bereichen bei Entwicklung und Anwendung sogenannter Atomchips, die für ihn “das Beste aus zwei Welten verbinden”: Die Quantenmanipulation der Atomphysik und die Quantenoptik mit den fantastischen Möglichkeiten der Nanotechnologie. Das Ziel dabei ist die detaillierte Quantenkontrolle über einzelne Atome, ohne dass die Quanteneffekte durch die Umgebung zerstört werden.
2003 gelang ihm erstmals die Herstellung eines sogenannten Bose-Einstein-Kondensats (BEC) auf einem Atom-Chip. Bei BEC handelt es sich um Atomwolken in einem exotischen Materiezustand nahe dem absoluten Nullpunkt (minus 273 Grad Celsius), die sich wie ein einzelnes Quantenobjekt verhalten. Die Physiker um Schmiedmayer können solche BEC in magnetischen und elektrischen Feldern eines Mikrochips beliebig manipulieren.
Damit lassen sich nicht nur mögliche Anwendungen in der Messtechnik demonstrieren, sondern auch fundamentale Fragen von Vielteilchen-Systemen untersuchen. Wie sich große, sehr komplexe Quantensysteme verhalten, ist eine zentrale Frage, spielen sie doch von der Entwicklung des frühen Universums bis zu den Funktionen von Biomolekülen eine wichtige Rolle.
Für die Weiterentwicklung dieser Arbeiten erhielt Schmiedmayer 2013 einen hochdotierten “Advanced Grant” des Europäischen Forschungsrats ERC. Im selben Jahr wurde er wirkliches Mitglied der Österreichischen Akademie der Wissenschaften (ÖAW).
Dieser Artikel wurde am 07.08.2020 veröffentlicht unter:
https://science.apa.at/rubrik/natur_und_technik/Mr_Atomchip_Joerg_Schmiedmayer_wird_60/SCI_20200807_SCI39391351455848914
Wien (APA) – Er ist eines der Aushängeschilder der österreichischen Quantenphysik: Der Experimentalphysiker Jörg Schmiedmayer, Professor für Quantenphysik an der Technischen Universität (TU) Wien. Sein Forschungsschwerpunkt liegt auf der Entwicklung von Atom-Chips zur kontrollierten Manipulation ultrakalter Atome, was ihm den Beinamen “Mr. Atomchip” eingetragen hat. Am Donnerstag (13.8.) wird er 60 Jahre alt.
Schmiedmayer, geboren am 13. August 1960 in Wien, studierte Physik an der TU Wien und Astronomie an der Uni Wien. Nach einem Zwischenstopp bei der Europäischen Organisation für Kernforschung CERN wurde er 1987 an der TU als Schüler von Helmut Rauch in Physik promoviert. Seine Post-Doc-Jahre absolvierte er, unterstützt von einem Schrödinger-Stipendium, an der Harvard University und am Massachusetts Institute for Technology (MIT), ehe er 1993 zu Anton Zeilinger ans Institut für Experimentalphysik der Uni Innsbruck wechselte. Dort habilitierte er sich 1997 für Experimentalphysik. 2000 nahm er einen Ruf an die Universität Heidelberg an, wo er eine Atomchip-Arbeitsgruppe aufbaute.
Rückholaktion von Siemens, Stadt Wien und TU Wien
Es bedurfte einiger Anstrengungen, Schmiedmayer 2005 wieder nach Österreich zurückzuholen. Für die “Rückholaktion” stellte Siemens anlässlich seines 125-jährigen Bestehens eine Mio. Euro bereit, die Stadt Wien zog mit der gleichen Summe mit. Die TU Wien, wo er eine seit Jahren vakante Professorenstelle für Experimentalphysik erhielt, stellte 1,6 Mio. Euro für den Umbau der Laboratorien und den notwendige Renovierungsarbeiten am Gebäude des Atominstituts zur Verfügung, wo der Physiker 2006 mit seiner Gruppe einzog.
Fix nach Wien übersiedelte Schmiedmayer erst, als sein neues Labor fix und fertig war und er seine Arbeit nahtlos fortsetzen konnte. “Wissenschaft ist wie ein Termingeschäft am Ölmarkt. Wenn man nicht der erste ist, kann man es gleich bleiben lassen”, zeigte er damals seinen Zugang zu wissenschaftlicher Arbeit. Wichtig ist ihm bei dieser vor allem die Unabhängigkeit, erfolgreich werde man darin, “indem man Sachen macht, die einem Spaß machen und die noch niemand gemacht hat”.
“Das Beste aus zwei Welten”
Das gelingt dem Physiker in vielen Bereichen bei Entwicklung und Anwendung sogenannter Atomchips, die für ihn “das Beste aus zwei Welten verbinden”: Die Quantenmanipulation der Atomphysik und die Quantenoptik mit den fantastischen Möglichkeiten der Nanotechnologie. Das Ziel dabei ist die detaillierte Quantenkontrolle über einzelne Atome, ohne dass die Quanteneffekte durch die Umgebung zerstört werden.
2003 gelang ihm erstmals die Herstellung eines sogenannten Bose-Einstein-Kondensats (BEC) auf einem Atom-Chip. Bei BEC handelt es sich um Atomwolken in einem exotischen Materiezustand nahe dem absoluten Nullpunkt (minus 273 Grad Celsius), die sich wie ein einzelnes Quantenobjekt verhalten. Die Physiker um Schmiedmayer können solche BEC in magnetischen und elektrischen Feldern eines Mikrochips beliebig manipulieren.
Damit lassen sich nicht nur mögliche Anwendungen in der Messtechnik demonstrieren, sondern auch fundamentale Fragen von Vielteilchen-Systemen untersuchen. Wie sich große, sehr komplexe Quantensysteme verhalten, ist eine zentrale Frage, spielen sie doch von der Entwicklung des frühen Universums bis zu den Funktionen von Biomolekülen eine wichtige Rolle.
Für die Weiterentwicklung dieser Arbeiten erhielt Schmiedmayer 2013 einen hochdotierten “Advanced Grant” des Europäischen Forschungsrats ERC. Im selben Jahr wurde er wirkliches Mitglied der Österreichischen Akademie der Wissenschaften (ÖAW).
Dieser Artikel wurde am 07.08.2020 veröffentlicht unter:
https://science.apa.at/rubrik/natur_und_technik/Mr_Atomchip_Joerg_Schmiedmayer_wird_60/SCI_20200807_SCI39391351455848914
Abstract:
Asymptotic safety is a theoretical proposal for the ultraviolet completion of quantum field theories, in particular for quantum gravity. Significant progress on this program has led to a first characterization of the Reuter fixed point. Further advancement in our understanding of the nature of quantum spacetime requires addressing a number of open questions and challenges. Here, we aim at providing a critical reflection on the state of the art in the asymptotic safety program, specifying and elaborating on open questions of both technical and conceptual nature. We also point out systematic pathways, in various stages of practical implementation, toward answering them. Finally, we also take the opportunity to clarify some common misunderstandings regarding the program.
A. Bonanno, A. Eichhorn, H. Gies, J. M. Pawlowski, R. Percacci, M. Reuter, F. Saueressig, G. P. Vacca, “Critical reflections on asymptotically safe gravity”, Front. in Phys. 8, 269 (2020).
https://www.frontiersin.org/articles/10.3389/fphy.2020.00269/full
Related to Project A02
Abstract:
We investigate the nonequilibrium evolution of the quark-meson model using 2PI effective action techniques. Our numerical simulations, which include the full dynamics of the order parameter of chiral symmetry, show how the model thermalizes into different regions of its phase diagram. In particular, by studying quark and meson spectral functions, we shed light on the real-time dynamics approaching the crossover transition, revealing e.g. the emergence of light effective fermionic degrees of freedom in the infrared. At late times in the evolution, the fluctuation-dissipation relation emerges naturally among both meson and quark degrees of freedom, confirming that the simulation successfully reaches the universal thermal fixed point.
L. Shen, J. Berges, J. Pawlowski, and A. Rothkopf, “Thermalization and dynamical spectral
properties in the quark-meson model”, Phys. Rev. D 102, 16012 (2020).
https://journals.aps.org/prd/abstract/10.1103/PhysRevD.102.016012
Related to Project B03, A01, A02
Abstract:
Currently, there are intense experimental efforts to realize lattice gauge theories in quantum simulators. Except for specific models, however, practical quantum simulators can never be fine-tuned to perfect local gauge invariance. There is thus a strong need for a rigorous understanding of gauge-invariance violation and how to reliably protect against it. As we show through analytic and numerical evidence, in the presence of a gauge invariance-breaking term the gauge violation accumulates only perturbatively at short times before proliferating only at very long times. This proliferation can be suppressed up to infinite times by energetically penalizing processes that drive the dynamics away from the initial gauge-invariant sector. Our results provide a theoretical basis that highlights a surprising robustness of gauge-theory quantum simulators.
J. C. Halimeh and P. Hauke, “Reliability of Lattice Gauge Theories”, Phys. Rev. Lett. 125, 030503
(2020).
https://journals.aps.org/prl/abstract/10.1103/PhysRevLett.125.030503
Related to Project: B04
Abstract:
We investigate a Bose-Einstein condensate in strong interaction with a single impurity particle. While this situation has received considerable interest in recent years, the regime of strong coupling remained inaccessible to most approaches due to an instability in the Bogoliubov theory arising near the resonance. We present a nonlocal extension of the Gross-Pitaevskii theory that is free of such divergences and does not require the use of the Born approximation in any of the interaction potentials. We find a new dynamical transition regime between attractive and repulsive polarons, where an interaction quench results in a finite number of coherent oscillations in the density profiles of the medium and in the contact parameter before equilibrium is reached.
M. Drescher, M. Salmhofer, and T. Enss, “Theory of a resonantly interacting impurity in a Bose-Einstein condensate”, Phys. Rev. Research 2, 032011(R) 2, 032011 (2020).
https://journals.aps.org/prresearch/abstract/10.1103/PhysRevResearch.2.032011
Related to Project C02, C03
Press Release Max-Planck-Gesellschaft
From 1 July 2020 the Max Planck Society has three new Vice Presidents: Asifa Akhtar, Ulman Lindenberger and Klaus Blaum are now part of the Executive Board, which advises President Martin Stratmann and prepares important decisions for the Society. They succeed Bill S. Hansson, Angela D. Friederici and Ferdi Schüth, who had performed outstanding services at Martin Stratmann’s side for six years.
My heart beats for the young scientists,” says Asifa Akhtar, Director and Scientific Member at the MPI for Immunobiology and Epigenetics in Freiburg since 2013. Born in 1971 in Pakistan, the biologist is the first female Vice President in the Biology and Medicine Section. During her term of office, she will be in charge of the Institutes of this Section and will also be the contact person for the Max Planck Schools. She takes over from Bill S. Hansson, Director at the MPI for Chemical Ecology in Jena. The Swedish-born neuroethologist was the first international Vice President at the top of the Max Planck Society, responsible for the area of internationalisation. The integration of new scientific members from abroad was also a matter close to his heart. With an onboarding brochure for new appointees, the “Travel Guide to the Max Planck Society”, he created a new and unconventional format providing new hires with a quick orientation to the Max Planck culture and an easy transition to life in Germany.
Asifa Akhtar now wants to continue this tradition. “Academic science is a beautiful example of integration because you have people from all over the world exchanging knowledge beyond boundaries, cultures or prejudice,” says the Asifa Akhtar. She also wants to advance the issue of equality. “Gender equality needs to be worked on continuously. There are outstanding women in science and we should make all the efforts and use our resources to win them for the Max Planck Society.” In addition, the new Vice President promotes transparent communication both internally and externally, calls for more diversity in the Max Planck Society, and wants to advocate cooperation with local educational institutions.
Ulman Lindenberger was appointed Vice President of the Human Sciences Section of the Max Planck Society. Since 2003, he has been Director of the Center for Lifespan Psychology at the Max Planck Institute for Human Development in Berlin. Ulman Lindenberger succeeds Angela D. Friederici, Director at the MPI for Human Cognitive and Brain Sciences in Leipzig. Over the last six years, Friederici has, among other things, supported the “Chefsache” initiative, a network of renowned leaders from industry and science to promote equal opportunities in Germany. The Lise Meitner Excellence Program was also based on her initiative – a programme that offers young women scientists a transparent career path within the Max Planck Society. Lindenberger will take over the scientific management of the Minerva Stiftung for the Promotion of Scientific Cooperation with Israel from his predecessor. “The Stiftung offers a wide range of instruments for promoting scientific collaboration between Germany and Israel,” says Lindenberger, who has been to Israel several times. In addition, he has made it his mission to strengthen the brand “Max Planck“ and provide new impulses for the appointment process in the Max Planck Society.
The third Vice President is Klaus Blaum. Having been the Director of the “Stored and cooled ions” Department at the Max Planck Institute for Nuclear Physics in Heidelberg since 2007, Blaum is the Vice President responsible for the Institutes of the Chemistry, Physics, and Technology Section. He takes over the office from Ferdi Schüth, who has rendered outstanding services to the Cyber Valley at the Stuttgart and Tübingen sites, the newly founded Max Planck Institute for Cyber Security and Privacy in Bochum, and the Max Planck Schools. Blaum also wants to get involved in technology transfer and Cyber Valley. One topic that particularly connects the physicist with his new colleague Lindenberger is that of sustainability: “I believe that in this area you can make a difference everywhere, and I am firmly convinced that once people realise this, they will want to become involved in sustainability efforts immediately”. In addition, Blaum, who has been maintaining close scientific cooperation in the Asian region for many years, wants to contribute to the exchange between the Max Planck Society and the Chinese Academy of Sciences. Within the Max Planck Society, Blaum sees himself as a bridge builder: “For me, it’s important to emphasize that I see myself as the link between the Section, the Directors, and the President, and the Administrative Headquarters.”
Further Links
https://www.mpg.de/15140971/klaus-blaum-vice-president-chemical-physical-technical-section
Contact
Dr. Christina Beck
Head of the Communication Department Administrative Headquarters of the Max Planck Society, München
Phone: +49 89 2108-1275
beck@gv.mpg.de
Petra Maaß
Editor, national and international research policy Administrative Headquarters of the Max Planck Society, München
Phone: +49 89 2108-1488
petra.maass@gv.mpg.de
Press Release Max-Planck-Gesellschaft
From 1 July 2020 the Max Planck Society has three new Vice Presidents: Asifa Akhtar, Ulman Lindenberger and Klaus Blaum are now part of the Executive Board, which advises President Martin Stratmann and prepares important decisions for the Society. They succeed Bill S. Hansson, Angela D. Friederici and Ferdi Schüth, who had performed outstanding services at Martin Stratmann’s side for six years.
My heart beats for the young scientists,” says Asifa Akhtar, Director and Scientific Member at the MPI for Immunobiology and Epigenetics in Freiburg since 2013. Born in 1971 in Pakistan, the biologist is the first female Vice President in the Biology and Medicine Section. During her term of office, she will be in charge of the Institutes of this Section and will also be the contact person for the Max Planck Schools. She takes over from Bill S. Hansson, Director at the MPI for Chemical Ecology in Jena. The Swedish-born neuroethologist was the first international Vice President at the top of the Max Planck Society, responsible for the area of internationalisation. The integration of new scientific members from abroad was also a matter close to his heart. With an onboarding brochure for new appointees, the “Travel Guide to the Max Planck Society”, he created a new and unconventional format providing new hires with a quick orientation to the Max Planck culture and an easy transition to life in Germany.
Asifa Akhtar now wants to continue this tradition. “Academic science is a beautiful example of integration because you have people from all over the world exchanging knowledge beyond boundaries, cultures or prejudice,” says the Asifa Akhtar. She also wants to advance the issue of equality. “Gender equality needs to be worked on continuously. There are outstanding women in science and we should make all the efforts and use our resources to win them for the Max Planck Society.” In addition, the new Vice President promotes transparent communication both internally and externally, calls for more diversity in the Max Planck Society, and wants to advocate cooperation with local educational institutions.
Ulman Lindenberger was appointed Vice President of the Human Sciences Section of the Max Planck Society. Since 2003, he has been Director of the Center for Lifespan Psychology at the Max Planck Institute for Human Development in Berlin. Ulman Lindenberger succeeds Angela D. Friederici, Director at the MPI for Human Cognitive and Brain Sciences in Leipzig. Over the last six years, Friederici has, among other things, supported the “Chefsache” initiative, a network of renowned leaders from industry and science to promote equal opportunities in Germany. The Lise Meitner Excellence Program was also based on her initiative – a programme that offers young women scientists a transparent career path within the Max Planck Society. Lindenberger will take over the scientific management of the Minerva Stiftung for the Promotion of Scientific Cooperation with Israel from his predecessor. “The Stiftung offers a wide range of instruments for promoting scientific collaboration between Germany and Israel,” says Lindenberger, who has been to Israel several times. In addition, he has made it his mission to strengthen the brand “Max Planck“ and provide new impulses for the appointment process in the Max Planck Society.
The third Vice President is Klaus Blaum. Having been the Director of the “Stored and cooled ions” Department at the Max Planck Institute for Nuclear Physics in Heidelberg since 2007, Blaum is the Vice President responsible for the Institutes of the Chemistry, Physics, and Technology Section. He takes over the office from Ferdi Schüth, who has rendered outstanding services to the Cyber Valley at the Stuttgart and Tübingen sites, the newly founded Max Planck Institute for Cyber Security and Privacy in Bochum, and the Max Planck Schools. Blaum also wants to get involved in technology transfer and Cyber Valley. One topic that particularly connects the physicist with his new colleague Lindenberger is that of sustainability: “I believe that in this area you can make a difference everywhere, and I am firmly convinced that once people realise this, they will want to become involved in sustainability efforts immediately”. In addition, Blaum, who has been maintaining close scientific cooperation in the Asian region for many years, wants to contribute to the exchange between the Max Planck Society and the Chinese Academy of Sciences. Within the Max Planck Society, Blaum sees himself as a bridge builder: “For me, it’s important to emphasize that I see myself as the link between the Section, the Directors, and the President, and the Administrative Headquarters.”
Contact
Dr. Christina Beck
Head of the Communication Department Administrative Headquarters of the Max Planck Society, München
Phone: +49 89 2108-1275
beck@gv.mpg.de
Petra Maaß
Editor, national and international research policy Administrative Headquarters of the Max Planck Society, München Phone: +49 89 2108-1488
petra.maass@gv.mpg.de
Abstract:
Time-domain interferometry (TDI) is a method to probe space-time correlations among particles in condensed matter systems. Applying TDI to quantum systems raises the general question of whether two-time correlations can be reliably measured without an adverse impact of the measurement backaction on the dynamics of the system. Here, we show that a recently developed quantum version of TDI (QTDI) indeed can access the full quantum-mechanical two-time correlations without backaction. We further generalize QTDI to weak classical continuous-mode coherent input states, alleviating the need for single-photon input fields. Finally, we interpret our results by splitting the space-time correlations into two parts. While the first one is associated with projective measurements and thus insensitive to backaction, we identify the second contribution as arising from the coherence properties of the state of the probed target system, such that it is perturbed or even destroyed by measurements on the system.
S. Castrignano and J. Evers, “Backaction-free measurement of quantum correlations via quantum
time-domain interferometry”, Phys. Rev. A 101, 063842 (2020).
https://journals.aps.org/pra/abstract/10.1103/PhysRevA.101.063842
Related to Project B02
Abstract:
A means to extract the fine-structure constant α from precision spectroscopic data on one-electron ions is presented. We show that in an appropriately weighted difference of the bound-electron g factor and the ground state energy, nuclear structural effects can be effectively suppressed. This method is anticipated to deliver an independent value of α via existing or near-future combined Penning trap and x-ray spectroscopic technology, and enables decreasing the uncertainty of α by orders of magnitude.
H. Cakir, N. S. Oreshkina, I. A. Valuev, V. A. Y. V. Debierre, C. H. Keitel, and Z. Harman,
“Improved access to the fine-structure constant with the simplest atomic systems”, arXiv:2006.14261 (2020).
https://arxiv.org/abs/2006.14261
Related to Project B02
Abstract:
We develop two ab initio quantum approaches to thin-film x-ray cavity quantum electrodynamics with spectrally narrow x-ray resonances, such as those provided by Mössbauer nuclei. The first method is based on a few-mode description of the cavity, and promotes and extends existing phenomenological few-mode models to an ab initio theory. The second approach uses analytically known Green’s functions to model the system. The two approaches not only enable one to ab initio derive the effective few-level scheme representing the cavity and the nuclei in the low-excitation regime, but also provide a direct avenue for studies at higher excitation, involving nonlinear or quantum phenomena. The ab initio character of our approaches further enables direct optimizations of the cavity structure and thus of the photonic environment of the nuclei, to tailor the effective quantum optical level scheme towards particular applications. To illustrate the power of the ab initio approaches, we extend the established quantum optical modeling to resonant cavity layers of arbitrary thickness, which is essential to achieve quantitative agreement for cavities used in recent experiments. Further, we consider multilayer cavities featuring electromagnetically induced transparency, derive their quantum optical few-level systems ab initio, and identify the origin of discrepancies in the modeling found previously using phenomenological approaches as arising from cavity field gradients across the resonant layers.
D. Lentrodt, K. P. Heeg, C. H. Keitel, and J. Evers, “Ab initio quantum models for thin-film
x-ray cavity qed”, Phys. Rev. Research 2, 023396 (2020).
https://journals.aps.org/prresearch/abstract/10.1103/PhysRevResearch.2.023396
Related to Project B02
Press Release No. 47/2020, 22 June 2020
One goal of science is to find physical descriptions of nature by studying how basic system components interact with one another. For complex many-body systems, effective theories are frequently used to this end. They allow describing the interactions without having to observe a system on the smallest of scales. Physicists at Heidelberg University have now developed a new method that makes it possible to identify such theories experimentally with the aid of so-called quantum simulators. The results of the research effort, led by Prof. Dr Markus Oberthaler (experimental physics) and Prof. Dr Jürgen Berges (theoretical physics), were published in the journal “Nature Physics”.
Deriving predictions about physical phenomena at the level of individual particles from a microscopic description is practically impossible for large systems. This applies not only to quantum mechanical many-body systems, but also to classical physics, such as when heated water in a cooking pot needs to be described at the level of the individual water molecules. But if a system is observed on large scales, like water waves in a pot, new properties can become relevant under certain preconditions. To describe such physics efficiently, effective theories are used. “Our research aimed to identify these theories in experiments with the help of quantum simulators,” explains Torsten Zache, the primary author of the theoretical portion of the study. Quantum simulators are used to modify many-body systems more simply and to calculate their properties.
The Heidelberg physicists recently demonstrated their newly developed method in an experiment on ultracold rubidium atoms, which are captured in an optical trap and brought out of equilibrium. “In the scenario we prepared, the atoms behave like tiny magnets whose orientation we are able to precisely read out using new processes,” according to Maximilian Prüfer, the primary author on the experimental side of the study. To determine the effective interactions of these “magnets”, the experiment has to be repeated several thousand times, which requires extreme stability.
“The underlying theoretical concepts allow us to interpret the experimental results in a completely new way and thereby gain insights through experiments into areas that have thus far been inaccessible through theory,” points out Prof. Oberthaler. “In turn, this can tell us about new types of theoretical approaches to successfully describe the relevant physical laws in complex many-body systems,” states Prof. Berges. The approach used by the Heidelberg physicists is transferrable to a number of other systems, thus opening groundbreaking territory for quantum simulations. Jürgen Berges and Markus Oberthaler are confident that this new way of identifying effective theories will make it possible to answer fundamental questions in physics.
The research work was conducted under the auspices of the “Isolated Quantum Systems and Universality In Extreme Conditions” Collaborative Research Centre (ISOQUANT) of Heidelberg University. It is also part of the “Entanglement Generation in Universal Time Dynamics” project, for which Prof. Oberthaler received an ERC Advanced Grant from the European Research Council (ERC).
Original Publication
M. Prüfer, T.V. Zache, P. Kunkel, S. Lannig, A. Bonnin, H. Strobel, J. Berges, and M. K. Oberthaler: Experimental extraction of the quantum effective action for a non-equilibrium many-body system. Nature physics (published online 15 June 2020).
https://doi.org/10.1038/s41567-020-0933-6
Contact
Prof. Dr. Markus Oberthaler
Kirchhoff Institute for Physics
INF 227
69120 Heidelberg
Phone: +49 6221 54 5170
markus.oberthaler@kip.uni-heidelberg.de
Prof. Dr. Jürgen Berges
Institute for Theoretical Physics
Philosophenweg 16
69120 Heidelberg
Phone: +49 6221 54 9422
j.berges@thphys.uni-heidelberg.de
Press Release No. 47/2020, 22 June 2020
One goal of science is to find physical descriptions of nature by studying how basic system components interact with one another. For complex many-body systems, effective theories are frequently used to this end. They allow describing the interactions without having to observe a system on the smallest of scales. Physicists at Heidelberg University have now developed a new method that makes it possible to identify such theories experimentally with the aid of so-called quantum simulators. The results of the research effort, led by Prof. Dr Markus Oberthaler (experimental physics) and Prof. Dr Jürgen Berges (theoretical physics), were published in the journal “Nature Physics”.
Deriving predictions about physical phenomena at the level of individual particles from a microscopic description is practically impossible for large systems. This applies not only to quantum mechanical many-body systems, but also to classical physics, such as when heated water in a cooking pot needs to be described at the level of the individual water molecules. But if a system is observed on large scales, like water waves in a pot, new properties can become relevant under certain preconditions. To describe such physics efficiently, effective theories are used. “Our research aimed to identify these theories in experiments with the help of quantum simulators,” explains Torsten Zache, the primary author of the theoretical portion of the study. Quantum simulators are used to modify many-body systems more simply and to calculate their properties.
The Heidelberg physicists recently demonstrated their newly developed method in an experiment on ultracold rubidium atoms, which are captured in an optical trap and brought out of equilibrium. “In the scenario we prepared, the atoms behave like tiny magnets whose orientation we are able to precisely read out using new processes,” according to Maximilian Prüfer, the primary author on the experimental side of the study. To determine the effective interactions of these “magnets”, the experiment has to be repeated several thousand times, which requires extreme stability.
“The underlying theoretical concepts allow us to interpret the experimental results in a completely new way and thereby gain insights through experiments into areas that have thus far been inaccessible through theory,” points out Prof. Oberthaler. “In turn, this can tell us about new types of theoretical approaches to successfully describe the relevant physical laws in complex many-body systems,” states Prof. Berges. The approach used by the Heidelberg physicists is transferrable to a number of other systems, thus opening groundbreaking territory for quantum simulations. Jürgen Berges and Markus Oberthaler are confident that this new way of identifying effective theories will make it possible to answer fundamental questions in physics.
The research work was conducted under the auspices of the “Isolated Quantum Systems and Universality In Extreme Conditions” Collaborative Research Centre (ISOQUANT) of Heidelberg University. It is also part of the “Entanglement Generation in Universal Time Dynamics” project, for which Prof. Oberthaler received an ERC Advanced Grant from the European Research Council (ERC).
Original Publication
M. Prüfer, T.V. Zache, P. Kunkel, S. Lannig, A. Bonnin, H. Strobel, J. Berges, and M. K. Oberthaler: Experimental extraction of the quantum effective action for a non-equilibrium many-body system. Nature physics (published online 15 June 2020).
https://doi.org/10.1038/s41567-020-0933-6
Contact
Prof. Dr. Markus Oberthaler
Kirchhoff Institute for Physics
INF 227
69120 Heidelberg
Phone: +49 6221 54 5170
markus.oberthaler@kip.uni-heidelberg.de
Prof. Dr. Jürgen Berges
Institute for Theoretical Physics
Philosophenweg 16
69120 Heidelberg
Phone: +49 6221 54 9422
j.berges@thphys.uni-heidelberg.de
Pressemitteilung Nr. 47/2020, 22. Juni 2020
Ein Ziel der Wissenschaft ist es, physikalische Beschreibungen der Natur zu finden, indem sie untersucht, wie grundlegende Bestandteile eines Systems miteinander wechselwirken. Für komplexe Vielteilchensysteme werden dazu häufig effektive Theorien verwendet. Damit können Wechselwirkungen beschrieben werden, ohne ein System auf kleinsten Skalen beobachten zu müssen. Physiker der Universität Heidelberg haben jetzt eine neue Methode entwickelt, die es möglich macht, derartige Theorien mithilfe sogenannter Quantensimulatoren experimentell zu bestimmen. Die Forschungsergebnisse, die unter der Leitung von Prof. Dr. Markus Oberthaler (Experimentalphysik) und Prof. Dr. Jürgen Berges (Theoretische Physik) entstanden, wurden in der Fachzeitschrift „Nature Physics“ veröffentlicht.
Vorhersagen über physikalische Phänomene auf der Ebene einzelner Teilchen aus einer mikroskopischen Beschreibung herzuleiten, ist für große Systeme praktisch unmöglich. Das gilt jenseits von quantenmechanischen Vielteilchensystemen bereits in der klassischen Physik, wenn zum Beispiel erhitztes Wasser in einem Kochtopf auf dem Level der einzelnen Wassermoleküle beschrieben werden soll. Betrachtet man allerdings ein System auf großen Skalen, etwa die Wasserwellen im Topf, so können unter bestimmten Voraussetzungen neue Eigenschaften relevant werden. Um deren Physik effizient zu beschreiben, verwendet die Physik effektive Theorien. „Diese Theorien mithilfe von Quantensimulatoren in Experimenten bestimmen zu können, war Ziel unserer Forschung“, sagt Torsten Zache, der für den theoretischen Teil Erstautor der Studie ist. Quantensimulatoren werden dabei eingesetzt, um Vielteilchensysteme einfacher zu modellieren und deren Eigenschaften zu berechnen.
Ihre neuentwickelte Methode haben die Heidelberger Physiker jetzt in einem Experiment mit ultrakalten Rubidiumatomen demonstriert. Diese werden in einer optischen Falle gefangen und aus dem Gleichgewicht gebracht. „In dem von uns so präparierten Szenario verhalten sich die Atome wie kleine Magnete, deren Ausrichtung wir mithilfe neuer Verfahren präzise auslesen können“, so Maximilian Prüfer, der Erstautor auf experimenteller Seite ist. Für die Bestimmung der effektiven Wechselwirkungen dieser „Magnete“ musste das Experiment viele tausend Male wiederholt werden, was eine hohe Stabilität erfordert.
„Die zugrundeliegenden theoretischen Konzepte erlauben es uns, die experimentellen Ergebnisse in einer völlig neuen Art zu interpretieren und damit in Experimenten Erkenntnisse in Bereichen zu erlangen, die bislang auf dem Weg der Theorie noch nicht zugänglich sind“, betont Prof. Oberthaler. „Dies wiederum kann uns Hinweise geben, in welche Richtung neuartige Theorieansätze erfolgreich sein können, um damit die relevanten Physikgesetze in komplexen Vielteilchensystemen zu beschreiben“, sagt Prof. Berges. Der Ansatz der Heidelberger Physiker ist auf eine Vielzahl anderer Systeme übertragbar und eröffnet daher wegweisende Perspektiven für Quantensimulationen. Diese neue Art der Bestimmung effektiver Theorien wird es ermöglichen, fundamentale Fragen in der Physik zu beantworten, sind sich Jürgen Berges und Markus Oberthaler sicher.
Die Forschungsarbeiten wurden im Rahmen des Sonderforschungsbereichs „Isolierte Quantensysteme und Universalität unter extremen Bedingungen“ (ISOQUANT) der Universität Heidelberg durchgeführt. Sie sind zugleich Teil des Projekts „Entanglement Generation in Universal Time Dynamics“, für das Prof. Oberthaler einen ERC Advanced Grant des Europäischen Forschungsrates (ERC) erhalten hat.
Originalpublikation
M. Prüfer, T.V. Zache, P. Kunkel, S. Lannig, A. Bonnin, H. Strobel, J. Berges, and M. K. Oberthaler: Experimental extraction of the quantum effective action for a non-equilibrium many-body system. Nature physics (published online 15 June 2020).
https://doi.org/10.1038/s41567-020-0933-6
Contact
Prof. Dr. Markus Oberthaler
Kirchhoff-Institut für Physik
INF 227
69120 Heidelberg
Telefon: +49 6221 54 5170
markus.oberthaler@kip.uni-heidelberg.de
Prof. Dr. Jürgen Berges
Institut für Theoretische Physik
Philosophenweg 16
69120 Heidelberg
Telefon: +49 6221 54 9422
j.berges@thphys.uni-heidelberg.de
Abstract:
We study an array of ultracold atoms in an optical lattice (Mott insulator) excited with a coherent ultrashort laser pulse to a state where single-electron wave functions spatially overlap. Beyond a threshold principal quantum number where Rydberg orbitals of neighboring lattice sites overlap with each other, the atoms efficiently undergo spontaneous Penning ionization resulting in a drastic change of ion-counting statistics, sharp increase of avalanche ionization, and the formation of an ultracold plasma. These observations signal the actual creation of electronic states with overlapping wave functions, which is further confirmed by a significant difference in ionization dynamics between a Bose-Einstein condensate and a Mott insulator. This system is a promising platform for simulating electronic many-body phenomena dominated by Coulomb interactions in the condensed phase.
M. Mizoguchi, Y. Zhang, M. Kunimi, A. Tanaka, S. Takeda, N. Takei, V. Bharti, K. Koyasu, T. Kishimoto, D. Jaksch, A. Glaetzle, M. Kiffner, G. Masella, G. Pupillo, M. Weidemüller, and K. Ohmori, “Ultrafast Creation of Overlapping Rydberg Electrons in an Atomic BEC and Mott-Insulator Lattice”, Phys. Rev. Lett. 124, 253201 (2020).
https://journals.aps.org/prl/abstract/10.1103/PhysRevLett.124.253201
Related to Project: A05
Abstract:
Within the ultimate goal of classifying universality in quantum many-body dynamics, understanding the relation between out-of-equilibrium and equilibrium criticality is a crucial objective. Models with power-law interactions exhibit rich well-understood critical behavior in equilibrium, but the out-of-equilibrium picture has remained incomplete, despite recent experimental progress. We construct the rich dynamical phase diagram of free-fermionic chains with power-law hopping and pairing and provide analytic and numerical evidence showing a direct connection between nonanalyticities of the return rate and zero crossings of the string order parameter. Our results may explain the experimental observation of so-called accidental dynamical vortices, which appear for quenches within the same topological phase of the Haldane model, as reported by Fläschner et al. [Nat. Phys. 14, 265 (2018)]. Our work is readily applicable to modern ultracold-atom experiments, not least because state-of-the-art quantum gas microscopes can now reliably measure the string order parameter, which, as we show, can serve as an indicator of dynamical criticality.
P. Uhrich, N. Defenu, R. Jafari, and J. C. Halimeh, “Out-of-equilibrium phase diagram of long-range superconductors”, Phys. Rev. B 101, 245148 (2020).
https://journals.aps.org/prb/abstract/10.1103/PhysRevB.101.245148
Related to Project C02
Abstract:
A simultaneous blast-wave fit to particle yields and elliptic flow (v2) measured as a function of transverse momentum in Pb-Pb collisions at energies available at the Large Hadron Collider (LHC) is presented. A compact formula for the calculation of v2(pT) for an elliptic freeze-out surface is used which follows from the Cooper-Frye ansatz without further assumptions. Over the full available pT range, the Υ elliptic flow data is described by the prediction based on the fit to lighter particles. This prediction shows that, due to the large Υ mass, a sizable elliptic flow is only expected at transverse momenta above 10GeV/c.
K. Reygers, A. Schmah, A. Berdnikova, and X. Sun, “Blast-wave description of elliptic flow
at energies available at the CERN Large Hadron Collider”, Phys. Rev. C 101, 064905 (2020).
https://journals.aps.org/prc/abstract/10.1103/PhysRevC.101.064905
Related to Project A01
Abstract:
QED corrections to the g factor of Li-like and B-like ions in a wide range of nuclear charges are presented. Many-electron contributions as well as radiative effects on the one-loop level are calculated. Contributions resulting from the interelectronic interaction, the self-energy effect, and most of the terms of the vacuum-polarization effect are evaluated to all orders in the nuclear coupling strength Zα. Uncertainties resulting from nuclear size effects, numerical computations, and uncalculated effects are discussed.
H. Cakir, V. A. Yerokhin, N. S. Oreshkina, B. Sikora, I. I. Tupitsyn, C. H. Keitel, Z. Harman: QED corrections to the g factor of Li- and B-like ions, Phys. Rev. A 101, 062513 (2020).
https://journals.aps.org/pra/abstract/10.1103/PhysRevA.101.062513
Related to Project B02
Abstract:
A simultaneous blast-wave fit to particle yields and elliptic flow (v2) measured as a function of transverse momentum in Pb-Pb collisions at energies available at the Large Hadron Collider (LHC) is presented. A compact formula for the calculation of v2(pT) for an elliptic freeze-out surface is used which follows from the Cooper-Frye ansatz without further assumptions. Over the full available pT range, the Υ elliptic flow data is described by the prediction based on the fit to lighter particles. This prediction shows that, due to the large Υ mass, a sizable elliptic flow is only expected at transverse momenta above 10GeV/c.
K. Reygers, A. Schmah, A. Berdnikova, and X. Sun, “Blast-wave description of elliptic flow
at energies available at the CERN Large Hadron Collider”, Phys. Rev. C 101, 064905 (2020).
https://journals.aps.org/prc/abstract/10.1103/PhysRevC.101.064905
Related to Project A01
Abstract:
Strongly interacting two-dimensional Fermi systems are one of the great remaining challenges in many-body physics due to the interplay of strong local correlations and enhanced long-range fluctuations. Here, we probe the thermodynamic and transport properties of a 2D Fermi gas across the BEC-BCS crossover by studying the propagation and damping of sound modes. We excite particle currents by imprinting a phase step onto homogeneous Fermi gases trapped in a box potential and extract the speed of sound from the frequency of the resulting density oscillations. We measure the speed of sound across the BEC-BCS crossover and compare the resulting dynamic measurement of the equation of state both to a static measurement based on recording density profiles and to quantum Monte Carlo calculations and find reasonable agreement between all three. We also measure the damping of the sound mode, which is determined by the shear and bulk viscosities as well as the thermal conductivity of the gas. We find that the damping is minimal in the strongly interacting regime and the diffusivity approaches the universal quantum bound ℏ/m of a perfect fluid.
M. Bohlen, L. Sobirey, N. Luick, H. Biss, T. Enss, T. Lompe, H. Moritz: Sound propagation and quantum limited damping in a two-dimensional Fermi gas, Phys. Rev. Lett. 124, 240403 (2020).
https://journals.aps.org/prl/abstract/10.1103/PhysRevLett.124.240403
Related to Project C02, C03
Abstract:
As a contribution to a viable candidate for a standard model of cosmology, we here show that pre-inflationary quantum fluctuations can provide a scenario for the long-sought initial conditions for the inflaton field. Our proposal is based on the assumption that at very high energies (higher than the energy scale of inflation) the vacuum-expectation value (VeV) of the field is trapped in a false vacuum and then, due to renormalization-group (RG) running, the potential starts to flatten out toward low energy, eventually tending to a convex one which allows the field to roll down to the true vacuum. We argue that the proposed mechanism should apply to large classes of inflationary potentials with multiple concave regions. Our findings favor a particle physics origin of chaotic, large-field inflationary models as we eliminate the need for large field fluctuations at the GUT scale. In our analysis, we provide a specific example of such an inflationary potential, whose parameters can be tuned to reproduce the existing cosmological data with good accuracy.
I. G. Marian, N. Defenu, U. D. Jentschura, A. Trombettoni, I. Nandori: Renormalization-Group Running Induced Cosmic Inflation, JCAP 06, 028 (2020).
https://iopscience.iop.org/article/10.1088/1475-7516/2020/06/028
Related to Project C02
Abstract:
We study string fragmentation in high multiplicity proton-proton collisions in a model where the string tension fluctuates. These fluctuations produce exponential pion spectra which are fitted to the transverse momentum distributions of charged particles for different multiplicities. For each multiplicity the so obtained hadronic slope parameter defines the magnitude of the string fluctuations which in turn determines the produced ratio of strange to light quarks. pythia string decay simulations are used to convert each ratio of strange to light quarks to the appropriate ratio of strange hadrons to pions.
H. J. Pirner, B. Kopeliovich, and K. Reygers, “Strangeness enhancement due to string fluctuations”,
Phys. Rev. D 101, 114010 (2020).
https://journals.aps.org/prd/abstract/10.1103/PhysRevD.101.114010
Related to Project A01
Abstract:
On the fundamental level, quantum fluctuations or entanglement lead to complex dynamical behaviour in many-body systems1 for which a description as emergent phenomena can be found within the framework of quantum field theory. A central quantity in these efforts, containing all information about the measurable physical properties, is the quantum effective action2. Though non-equilibrium quantum dynamics can be exactly formulated in terms of the quantum effective action, finding solutions is in general beyond the capabilities of classical computers3. Here, we present a strategy to determine the non-equilibrium quantum effective action4 using analogue quantum simulators, and demonstrate our method experimentally with a quasi-one-dimensional spinor Bose gas out of equilibrium5,6. Spatially resolved snapshots of the complex-valued transversal spin field7 allow us to infer the quantum effective action up to fourth order in an expansion in one-particle irreducible correlation functions at equal times. We uncover a strong suppression of the irreducible four vertex emerging at low momenta in the highly occupied regime far from equilibrium where perturbative descriptions fail8. Our work constitutes a new realm of large-scale analogue quantum computing9, where highly controlled synthetic quantum systems10 provide the means for solving theoretical problems in high-energy and condensed-matter physics with an experimental approach11,12,13,14.
M. Prüfer, T. V. Zache, P. Kunkel, S. Lannig, A. Bonnin, H. Strobel, J. Berges, and M. K. Oberthaler, “Experimental extraction of the quantum effective action for a non-equilibrium many-body system”, Nature Physics 16, 1012–1016 (2020).
https://www.nature.com/articles/s41567-020-0933-6
Related to Project B04, A04, A03
Abstract:
Quantum fluctuations lead to an anomalous violation of parity symmetry in quantum electrodynamics for an even number of spatial dimensions. While the leading parity-odd electric current vanishes in vacuum, we uncover a non-cancellation of the anomaly for strong electric fields with distinct macroscopic signatures. We perform real-time lattice simulations with fully dynamical gauge fields and Wilson fermions in space-time dimensions. In the static field limit, relevant at early times, we solve the problem analytically. Our results point out the fundamental role of quantum anomalies for strong-field phenomena, relevant for a wide range of condensed matter and high-energy applications, but also for the next generation of gauge theory quantum simulators.
R. Ott, T. V. Zache, N. Müller, and J. Berges, “Non-cancellation of the parity anomaly in the strong-field regime of QED2+1”, Phys. Lett. B 805, 135459 (2020).
https://www.sciencedirect.com/science/article/pii/S037026932030263X?via%3Dihub
Related to Project: A01, B03, B02
Abstract:
Strongly correlated systems are often associated with an underlying quantum critical point which governs their behavior in the finite temperature phase diagram. Their thermodynamical and transport properties arise from critical fluctuations and follow universal scaling laws. Here, we develop a microscopic theory of thermal transport in the quantum critical regime expressed in terms of a thermal sum rule and an effective scattering time. We explicitly compute the characteristic scaling functions in a quantum critical model system, the unitary Fermi gas. Moreover, we derive an exact thermal sum rule for heat and energy currents and evaluate it numerically using the nonperturbative Luttinger-Ward approach. For the thermal scattering times we find a simple quantum critical scaling form. Together, this determines the heat conductivity, thermal diffusivity, Prandtl number and sound diffusivity from high temperatures down into the quantum critical regime. The results provide a quantitative description of recent sound attenuation measurements in ultracold Fermi gases.
B. Frank, W. Zwerger, T. Enss: Quantum critical thermal transport in the unitary Fermi gas, Phys. Rev. Research 2, 023301 (2020).
https://journals.aps.org/prresearch/abstract/10.1103/PhysRevResearch.2.023301
Related to Project C02
Abstract:
Transverse momentum spectra of identified particles produced in heavy-ion collisions at the Large Hadron Collider are described with relativistic fluid dynamics. We perform a systematic comparison of experimental data for pions, kaons and protons up to a transverse momentum of 3 GeV/c with calculations using the FluiduM code package to solve the evolution equations of fluid dynamics, the TrENTo model to describe the initial state and the FastReso code to take resonance decays into account. Using data in five centrality classes at the center-of-mass collision energy per nucleon pair sNN−−−√ = 2.76 TeV, we determine systematically the most likely parameters of our theoretical model including the shear and bulk viscosity to entropy ratios, the initialization time, initial density and freeze-out temperature through a global search and quantify their posterior probability. This is facilitated by the very efficient numerical implementation of FluiduM and FastReso. Based on the most likely model parameters we present predictions for the transverse momentum spectra of multi-strange hadrons as well as identified particle spectra from Pb-Pb collisions at sNN−−−√ = 5.02 TeV.
D. Devetak, A. Dubla, S. Floerchinger, E. Grossi, S. Masciocchi, A. Mazeliauskas, and I. Selyuzhenkov, “Global fluid fits to identified particle transverse momentum spectra from heavy-ion collisions at the Large Hadron Collider”, JHEP 06, 44 (2020).
https://link.springer.com/article/10.1007%2FJHEP06(2020)044
Related to Project: A01, A02, C06, ABC
Abstract:
We study a transition between a homogeneous and an inhomogeneous phase in a system of one-dimensional, Raman tunnel-coupled Bose gases. The homogeneous phase shows a flat density and phase profile, whereas the inhomogeneous ground state is characterized by periodic density ripples, and a soliton staircase in the phase difference. We show that under experimentally viable conditions the transition can be tuned by the wavevector difference Q of the Raman beams and can be described by the Pokrovsky-Talapov model for the relative phase between the two condensates. Local imaging available in atom chip experiments allows to observe the soliton lattice directly, while modulation spectroscopy can be used to explore collective modes, such as the phonon mode arising from breaking of translation symmetry by the soliton lattice. In addition, we investigate regimes where the cold atom experiment deviates from the Pokrovsky-Talapov field theory. We predict unusual mesoscopic effects arising from the finite size of the system, such as quantized injection of solitons upon increasing Q, or the system size. For moderate values of Q above criticality, we find that the density modulations in the two gases interplay with the relative phase profile and introduce novel features in the spatial structure of the mode wave-functions. Using an inhomogeneous Bogoliubov theory, we show that spatial quantum fluctuations are intertwined with the emerging soliton staircase. Finally, we comment on the prospects of the ultra-cold atom setup as a tunable platform studying quantum aspects of the Pokrovsky-Talapov theory in and out-of-equilibrium.
V. Kasper, J. Marino, S. Ji, V. Gritsev, J. Schmiedmayer, and E. Demler, “Simulating a quantum
commensurate-incommensurate phase transition using two Raman coupled one dimensional
condensates”, Phys. Rev. B 101, 224102 (2020).
https://journals.aps.org/prb/abstract/10.1103/PhysRevB.101.224102
Related to Project A03
Press Release Nr. 40/2020, 29 May 2020
Heidelberg University succeeded in gaining funding from the German Research Foundation (DFG) in the latest approval round for three, internationally visible collaborative research centres. In physics, the collaborative research centre (CRC) studying isolated quantum systems in experimental and theoretical investigations will continue its work in the second funding period (CRC 1225). Funding has also been extended for two research consortia which are organised as transregional CRCs, each with several participating universities: Scientists of the Medical Faculty Heidelberg along with partners in Freiburg and Munich are involved in hepatitis research (CRC/TRR 179). In the molecular life sciences, researchers in Heidelberg and Berlin are investigating the coordination of signal transmission in living cells (CRC/TRR 186). DFG funding for the upcoming four-year period for all three consortia totals more than 40 million euros.
CRC 1225, “Isolated Quantum Systems and Universality in Extreme Conditions” (ISOQUANT), is focused on a field of research of major importance for numerous applications in physics. Many of these systems exhibit characteristic common properties despite fundamental differences in key parameters such as temperature, density, or field strength. To better understand them, the CRC has adopted cross-disciplinary research approaches that go beyond traditional specialisations. In the first funding phase, the researchers in CRC 1225 were able to uncover new relationships between very different physical systems. For example, laboratory experiments with ultracold atoms point to conclusions about the dynamic properties of matter in the early universe shortly after the Big Bang. The results open up new possibilities in quantum simulation, whereby the properties of different systems can be mapped onto a “reference system” that can be studied in the laboratory. “Exploring universal aspects of very different physical quantum systems allows for a broad bandwidth of experimental and theoretical methods to solve overarching issues in physics. Using this interdisciplinary approach, we made important progress in answering fundamental questions and opened up new avenues for continued research that promise an exciting second funding period,” states Prof. Dr Jürgen Berges, spokesperson of the ISOQUANT CRC and researcher at the Institute for Theoretical Physics of Heidelberg University. The DFG is supporting CRC 1225 with approximately 14.3 million euros.
[…]
Contact
Prof. Dr. Jürgen Berges
Institute for Theoretical Physics
Philosophenweg 16
69120 Heidelberg
Phone: +49 6221 54 9422
j.berges@thphys.uni-heidelberg.de
Press Release Nr. 40/2020, 29 May 2020
Heidelberg University succeeded in gaining funding from the German Research Foundation (DFG) in the latest approval round for three, internationally visible collaborative research centres. In physics, the collaborative research centre (CRC) studying isolated quantum systems in experimental and theoretical investigations will continue its work in the second funding period (CRC 1225). Funding has also been extended for two research consortia which are organised as transregional CRCs, each with several participating universities: Scientists of the Medical Faculty Heidelberg along with partners in Freiburg and Munich are involved in hepatitis research (CRC/TRR 179). In the molecular life sciences, researchers in Heidelberg and Berlin are investigating the coordination of signal transmission in living cells (CRC/TRR 186). DFG funding for the upcoming four-year period for all three consortia totals more than 40 million euros.
CRC 1225, “Isolated Quantum Systems and Universality in Extreme Conditions” (ISOQUANT), is focused on a field of research of major importance for numerous applications in physics. Many of these systems exhibit characteristic common properties despite fundamental differences in key parameters such as temperature, density, or field strength. To better understand them, the CRC has adopted cross-disciplinary research approaches that go beyond traditional specialisations. In the first funding phase, the researchers in CRC 1225 were able to uncover new relationships between very different physical systems. For example, laboratory experiments with ultracold atoms point to conclusions about the dynamic properties of matter in the early universe shortly after the Big Bang. The results open up new possibilities in quantum simulation, whereby the properties of different systems can be mapped onto a “reference system” that can be studied in the laboratory. “Exploring universal aspects of very different physical quantum systems allows for a broad bandwidth of experimental and theoretical methods to solve overarching issues in physics. Using this interdisciplinary approach, we made important progress in answering fundamental questions and opened up new avenues for continued research that promise an exciting second funding period,” states Prof. Dr Jürgen Berges, spokesperson of the ISOQUANT CRC and researcher at the Institute for Theoretical Physics of Heidelberg University. The DFG is supporting CRC 1225 with approximately 14.3 million euros.
[…]
Contact
Prof. Dr. Jürgen Berges
Institute for Theoretical Physics
Philosophenweg 16
69120 Heidelberg
Phone: +49 6221 54 9422
j.berges@thphys.uni-heidelberg.de
Abstract:
The multi-critical fixed points of O(N) symmetric models cease to exist in the N→∞ limit, but the mechanism regulating their annihilation still presents several enigmatic aspects. Here, we explore the evolution of high-order multi-critical points in the (d,N) plane and uncover a complex mosaics for their asymptotic behaviour at large N. This picture is confirmed by various RG approaches and constitutes a fundamental step towards the full comprehension of critical behaviour in O(N) field theories.
https://arxiv.org/abs/2005.1082
Related to Project: C02, C03
Abstract:
Machine learning has the potential to aid our understanding of phase structures in lattice quantum field theories through the statistical analysis of Monte Carlo samples. Available algorithms, in particular those based on deep learning, often demonstrate remarkable performance in the search for previously unidentified features, but tend to lack transparency if applied naively. To address these shortcomings, we propose representation learning in combination with interpretability methods as a framework for the identification of observables. More specifically, we investigate action parameter regression as a pretext task while using layer-wise relevance propagation (LRP) to identify the most important observables depending on the location in the phase diagram. The approach is put to work in the context of a scalar Yukawa model in (2+1)d. First, we investigate a multilayer perceptron to determine an importance hierarchy of several predefined, standard observables. The method is then applied directly to the raw field configurations using a convolutional network, demonstrating the ability to reconstruct all order parameters from the learned filter weights. Based on our results, we argue that due to its broad applicability, attribution methods such as LRP could prove a useful and versatile tool in our search for new physical insights. In the case of the Yukawa model, it facilitates the construction of an observable that characterises the symmetric phase.
S. Bluecher, L. Kades, J. M. Pawlowski, N. Strodthoff, and J. M. Urban, “Towards Novel
Insights in Lattice Field Theory with Explainable Machine Learning”, Phys. Rev. D 101, 094507
(2020)
https://journals.aps.org/prd/abstract/10.1103/PhysRevD.101.094507
Related to Project A02, B03
Abstract:
The excitation of the 8 eV 229mTh isomer through the electronic bridge mechanism in highly charged ions is investigated theoretically. By exploiting the rich level scheme of open 4f orbitals and the robustness of highly charged ions against photoionization, a pulsed high-intensity optical laser can be used to efficiently drive the nuclear transition by coupling it to the electronic shell. We show how to implement a promising electronic bridge scheme in an electron beam ion trap starting from a metastable electronic state. This setup would avoid the need for a tunable vacuum ultraviolet laser. Based on our theoretical predictions, determining the isomer energy with an uncertainty of 10−5 eV could be achieved in one day of measurement time using realistic laser parameters.
P. V. Bilous, H. Bekker, J. Berengut, B. Seiferle, L. von der Wense, P. G. Thirolf, T. Pfeifer, J. R. Crespo López-Urrutia, A. Pálffy.: Electronic bridge excitation in highly charged Th-229 ions, Phys. Rev. Lett. 124, 192502 (2020).
https://journals.aps.org/prl/abstract/10.1103/PhysRevLett.124.192502
Related to Project B01, B02
Press Release MPIK Heidelberg, 06 May 2020
A new door to the quantum world has been opened: when an atom absorbs or releases energy via the quantum leap of an electron, it becomes heavier or lighter. This can be explained by Einstein’s theory of relativity (E = mc2). However, the effect is minuscule for a single atom. Nevertheless, the team of Klaus Blaum and Sergey Eliseev at the Max Planck Institute for Nuclear Physics has successfully measured this infinitesimal change in the mass of individual atoms for the first time. In order to achieve this, they used the ultra-precise Pentatrap atomic balance at the Institute in Heidelberg. The team discovered a previously unobserved quantum state in rhenium, which could be interesting for future atomic clocks. Above all, this extremely sensitive atomic balance enables a better understanding of the complex quantum world of heavy atoms. [Nature, 6th May 2020]
Astonishing, but true: if you wind a mechanical watch, it becomes heavier. The same thing happens when you charge your smartphone. This can be explained by the equivalence of energy (E) and mass (m), which Einstein expressed in the most famous formula in physics: E = mc2 (c: speed of light in vacuum). However, this effect is so small that it completely eludes our everyday experience. A conventional balance would not be able to detect it.
But at the Max Planck Institute for Nuclear Physics in Heidelberg, there is a balance that can: Pentatrap. It can measure the minuscule change in mass of a single atom when an electron in it absorbs or releases energy via a quantum jump, thus opening a new world for precision physics. Such quantum jumps in the electron shells of atoms shape our world – whether in life-giving photosynthesis and general chemical reactions or in the creation of colour and our vision.
An ant on top of an elephant
Rima Schüssler, now a postdoctoral fellow at the Max Planck Institute for Nuclear Physics, has helped build Pentatrap since completing her Master’s thesis in 2014. She is the lead author of a paper on an unexpected discovery made in a collaboration at the Max Planck PTB Riken Centre: In rhenium, there is a previously undiscovered electronic quantum state with special properties. Schüssler uses the following analogy to describe the degree of sensitivity with which Pentatrap can detect the jump of an electron into this quantum state via the mass change of a rhenium atom: “By weighing a six-tonne elephant, we were able to determine whether a ten-milligram ant was crawling on it”.
Pentatrap consists of five Penning traps. In order for such a trap to be able to weigh an atom, it must be electrically charged (i.e. become an ion). Because rhenium was stripped of 29 of its 75 electrons, it is highly charged. This dramatically increases the accuracy of the measurement. The trap captures this highly charged rhenium ion in a combination of a magnetic field and a specially shaped electric field. Inside, it travels in a circular path, which is intricately twisted into itself. In principle, it can be thought of as a ball on a rope, which is allowed to rotate in the air. If this is done with constant force, a heavier ball rotates slower than a lighter one.
An extremely long-lived quantum state in rhenium
In Pentatrap, two rhenium ions rotated alternately in the stacked traps. One ion was in the energetically lowest quantum state. When the second ion was generated, an electron was randomly excited into a higher state by supplying energy. In a sense, it was the wound watch. Because of the stored energy, it became marginally heavier and thus circulated slower than the first ion. Pentatrap precisely counts the number of revolutions per time unit. The difference in the number of revolutions yielded the increase in weight.
Using this method, the team discovered an extremely long-lived quantum state in rhenium. It is metastable (i.e. it decays after a certain lifetime). According to the calculations of theoreticians from the institute led by Zoltán Harman and Christoph H. Keitel, the University of Heidelberg, and the Kastler Brossel Laboratory in Paris, this is 130 days. The position of the quantum state also agrees quite well with model calculations using state-of-the-art quantum mechanical methods.
Possible application in future atomic clocks
Such excited electronic states in highly charged ions are interesting for basic research as well as for possible application in future atomic clocks as researched by the working group of José Crespo López-Urrutia at the Institute in cooperation with the Physikalisch-Technische Bundesanstalt (PTB). For them, the metastable state in rhenium is attractive for several reasons. First, because of its longevity, it corresponds to a sharp orbital frequency of the electron around the atomic nucleus. Second, the electron can be excited with soft X-ray light to jump into this quantum state. In principle, such a clock could tick faster and therefore even more accurately than the current generation of optical atomic clocks. However, according to Ekkehard Peik, who is in charge of the “Time and Frequency” Department at PTB and who was not involved in the work, it is still too early to speculate whether the discovery could be suitable for a new generation of atomic clocks.
“Nevertheless, this new method for discovering long-lived quantum states is spectacular”, stresses the physicist. He imagines that atomic clocks working with such new quantum states could initially offer a new test field for basic research. Because the rhenium ions lack many mutually shielding electrons, the remaining electrons feel the electric field of the atomic nucleus particularly strongly. The electrons therefore race around the nucleus at such high speeds that their motion must be described using Einstein’s theory of special relativity. With the new atomic balance, it would also be possible to test with high precision whether special relativity and quantum theory interact as described by this theory.
In general, the new atomic balance offers a novel access to the quantum-like inner life of heavier atoms. Because these consist of many particles – electrons, protons, and neutrons – they cannot be calculated exactly. The atomic models for theoretical calculations are therefore based on simplifications, and these can now be checked extremely accurately. It might be possible to use such atoms as probes in the search for unknown particles, which can be detected only by the extremely weak gravitational force. This dark matter is one of the greatest unsolved mysteries of physics.
On the path to new physics
An important step towards the access of new physics with atomic-physical methods was also achieved with Pentatrap [Phys. Rev. Lett. 124, 113001]. The Heidelberg researchers carried out mass measurements on a chain of five pairs of xenon isotopes. Using high-resolution laser spectroscopy on similar chains of other elements such as calcium and ytterbium, a linear relationship can be inferred from the small energy differences (isotope shift). Nonlinear deviations from this can, however, be an indication of new physics (further fundamental interactions, new particles, dark matter), which manifests itself under extremely precise observation – an alternative to high-energy experiments. Here too, close cooperation with theory (group of Zoltan Harman at MPIK) should be emphasized. The direct measurement of the binding energy of an electron in a highly charged ion shows a very good agreement with relativistic atomic structure calculations. This creates the basis e.g. for future high-precision tests of quantum electrodynamics.
(RW/BF)
Note: This research was funded by the European Research Council via the Advanced Grant “FunI”.
Original publication
Schüssler, R. X., H. Bekker, M. Braß, H. Cakir, J. R. Crespo López-Urrutia, M. Door, P. Filianin, Z. Harman, M. W. Haverkort, W. J. Huang, P. Indelicato, C. H. Keitel, C. M. König, K. Kromer, M. Müller, Y. N. Novikov, A. Rischka, C. Schweiger, S. Sturm, S. Ulmer, S. Eliseev, K. Blaum: Detection of metastable electronic states by Penning trap mass spectrometry, Nature 581, 42–46 (2020), (DOI: 10.1038/s41586-020-2221-0).
Contact
Dr. Rima Schüssler
Tel.: +49 6221 516-271
rima.schuessler@mpi-hd.mpg.de
Prof. Dr. Klaus Blaum
Tel.: +49 6221 516-851
klaus.blaum@mpi-hd.mpg.de
Press Release MPIK Heidelberg, 06 May 2020
A new door to the quantum world has been opened: when an atom absorbs or releases energy via the quantum leap of an electron, it becomes heavier or lighter. This can be explained by Einstein’s theory of relativity (E = mc2). However, the effect is minuscule for a single atom. Nevertheless, the team of Klaus Blaum and Sergey Eliseev at the Max Planck Institute for Nuclear Physics has successfully measured this infinitesimal change in the mass of individual atoms for the first time. In order to achieve this, they used the ultra-precise Pentatrap atomic balance at the Institute in Heidelberg. The team discovered a previously unobserved quantum state in rhenium, which could be interesting for future atomic clocks. Above all, this extremely sensitive atomic balance enables a better understanding of the complex quantum world of heavy atoms. [Nature, 6th May 2020]
Astonishing, but true: if you wind a mechanical watch, it becomes heavier. The same thing happens when you charge your smartphone. This can be explained by the equivalence of energy (E) and mass (m), which Einstein expressed in the most famous formula in physics: E = mc2 (c: speed of light in vacuum). However, this effect is so small that it completely eludes our everyday experience. A conventional balance would not be able to detect it.
But at the Max Planck Institute for Nuclear Physics in Heidelberg, there is a balance that can: Pentatrap. It can measure the minuscule change in mass of a single atom when an electron in it absorbs or releases energy via a quantum jump, thus opening a new world for precision physics. Such quantum jumps in the electron shells of atoms shape our world – whether in life-giving photosynthesis and general chemical reactions or in the creation of colour and our vision.
An ant on top of an elephant
Rima Schüssler, now a postdoctoral fellow at the Max Planck Institute for Nuclear Physics, has helped build Pentatrap since completing her Master’s thesis in 2014. She is the lead author of a paper on an unexpected discovery made in a collaboration at the Max Planck PTB Riken Centre: In rhenium, there is a previously undiscovered electronic quantum state with special properties. Schüssler uses the following analogy to describe the degree of sensitivity with which Pentatrap can detect the jump of an electron into this quantum state via the mass change of a rhenium atom: “By weighing a six-tonne elephant, we were able to determine whether a ten-milligram ant was crawling on it”.
Pentatrap consists of five Penning traps. In order for such a trap to be able to weigh an atom, it must be electrically charged (i.e. become an ion). Because rhenium was stripped of 29 of its 75 electrons, it is highly charged. This dramatically increases the accuracy of the measurement. The trap captures this highly charged rhenium ion in a combination of a magnetic field and a specially shaped electric field. Inside, it travels in a circular path, which is intricately twisted into itself. In principle, it can be thought of as a ball on a rope, which is allowed to rotate in the air. If this is done with constant force, a heavier ball rotates slower than a lighter one.
An extremely long-lived quantum state in rhenium
In Pentatrap, two rhenium ions rotated alternately in the stacked traps. One ion was in the energetically lowest quantum state. When the second ion was generated, an electron was randomly excited into a higher state by supplying energy. In a sense, it was the wound watch. Because of the stored energy, it became marginally heavier and thus circulated slower than the first ion. Pentatrap precisely counts the number of revolutions per time unit. The difference in the number of revolutions yielded the increase in weight.
Using this method, the team discovered an extremely long-lived quantum state in rhenium. It is metastable (i.e. it decays after a certain lifetime). According to the calculations of theoreticians from the institute led by Zoltán Harman and Christoph H. Keitel, the University of Heidelberg, and the Kastler Brossel Laboratory in Paris, this is 130 days. The position of the quantum state also agrees quite well with model calculations using state-of-the-art quantum mechanical methods.
Possible application in future atomic clocks
Such excited electronic states in highly charged ions are interesting for basic research as well as for possible application in future atomic clocks as researched by the working group of José Crespo López-Urrutia at the Institute in cooperation with the Physikalisch-Technische Bundesanstalt (PTB). For them, the metastable state in rhenium is attractive for several reasons. First, because of its longevity, it corresponds to a sharp orbital frequency of the electron around the atomic nucleus. Second, the electron can be excited with soft X-ray light to jump into this quantum state. In principle, such a clock could tick faster and therefore even more accurately than the current generation of optical atomic clocks. However, according to Ekkehard Peik, who is in charge of the “Time and Frequency” Department at PTB and who was not involved in the work, it is still too early to speculate whether the discovery could be suitable for a new generation of atomic clocks.
“Nevertheless, this new method for discovering long-lived quantum states is spectacular”, stresses the physicist. He imagines that atomic clocks working with such new quantum states could initially offer a new test field for basic research. Because the rhenium ions lack many mutually shielding electrons, the remaining electrons feel the electric field of the atomic nucleus particularly strongly. The electrons therefore race around the nucleus at such high speeds that their motion must be described using Einstein’s theory of special relativity. With the new atomic balance, it would also be possible to test with high precision whether special relativity and quantum theory interact as described by this theory.
In general, the new atomic balance offers a novel access to the quantum-like inner life of heavier atoms. Because these consist of many particles – electrons, protons, and neutrons – they cannot be calculated exactly. The atomic models for theoretical calculations are therefore based on simplifications, and these can now be checked extremely accurately. It might be possible to use such atoms as probes in the search for unknown particles, which can be detected only by the extremely weak gravitational force. This dark matter is one of the greatest unsolved mysteries of physics.
On the path to new physics
An important step towards the access of new physics with atomic-physical methods was also achieved with Pentatrap [Phys. Rev. Lett. 124, 113001]. The Heidelberg researchers carried out mass measurements on a chain of five pairs of xenon isotopes. Using high-resolution laser spectroscopy on similar chains of other elements such as calcium and ytterbium, a linear relationship can be inferred from the small energy differences (isotope shift). Nonlinear deviations from this can, however, be an indication of new physics (further fundamental interactions, new particles, dark matter), which manifests itself under extremely precise observation – an alternative to high-energy experiments. Here too, close cooperation with theory (group of Zoltan Harman at MPIK) should be emphasized. The direct measurement of the binding energy of an electron in a highly charged ion shows a very good agreement with relativistic atomic structure calculations. This creates the basis e.g. for future high-precision tests of quantum electrodynamics.
(RW/BF)
Note: This research was funded by the European Research Council via the Advanced Grant “FunI”.
_______________________________________________________________________________
Original publication
Schüssler, R. X., H. Bekker, M. Braß, H. Cakir, J. R. Crespo López-Urrutia, M. Door, P. Filianin, Z. Harman, M. W. Haverkort, W. J. Huang, P. Indelicato, C. H. Keitel, C. M. König, K. Kromer, M. Müller, Y. N. Novikov, A. Rischka, C. Schweiger, S. Sturm, S. Ulmer, S. Eliseev, K. Blaum: Detection of metastable electronic states by Penning trap mass spectrometry, Nature 581, 42–46 (2020), (DOI: 10.1038/s41586-020-2221-0).
_______________________________________________________________________________
Contact
Dr. Rima Schüssler
Tel.: +49 6221 516-271
rima.schuessler@mpi-hd.mpg.de
Prof. Dr. Klaus Blaum
Tel.: +49 6221 516-851
klaus.blaum@mpi-hd.mpg.de
Pressemitteilung des Max-Planck-Instituts für Kernphysik Heidelberg, 06. Mai 2020
Ein neuer Zugang zur Quantenwelt tut sich auf: Wenn ein Atom über den Quantensprung eines Elektrons Energie aufnimmt oder abgibt, wird es schwerer oder leichter. Ursache ist Einsteins E = mc2. Allerdings ist dieser Effekt bei einem einzelnen Atom ultraklein. Trotzdem gelang es nun einer internationalen Kooperation unter der Führung eines Teams um Klaus Blaum und Sergey Eliseev am Max-Planck-Institut für Kernphysik, diese winzige Massenveränderung einzelner Atome erstmals zu messen. Sie setzte dafür die ultrapräzise Atom-Waage Pentatrap am Institut in Heidelberg ein. Damit entdeckte die Kooperation in Rhenium einen bislang unbeobachteten Quantenzustand, der für zukünftige Atomuhren interessant sein könnte. Vor allem ermöglicht diese extrem empfindliche Atomwaage jedoch ein besseres Verständnis der komplexen Quantenwelt schwerer Atome. [Nature, 06.05.2020]
Es ist erstaunlich, aber wahr: Zieht man eine mechanische Uhr auf, wird sie schwerer, ebenso ein Smartphone beim Aufladen. Ursache ist die Äquivalenz von Energie (E) und Masse (m), die Einstein in die berühmteste Formel der Physik gefasst hat: E = mc2 (c: Vakuumlichtgeschwindigkeit). Allerdings ist dieser Effekt so klein, dass er sich unserer Alltagserfahrung völlig entzieht. Keine uns zugängliche Waage könnte ihn erfassen.
In Heidelberg gibt es allerdings eine solche Waage, am Max-Planck-Institut für Kernphysik. Pentatrap eröffnet der Präzisionsphysik eine neue Welt. Sie kann die unglaublich winzige Massenänderung eines einzelnen Atoms messen, wenn darin ein Elektron über einen Quantensprung Energie aufnimmt oder abgibt. Solche Quantensprünge in den Elektronenhüllen der Atome gestalten unsere Welt, sei es in der lebenspendenden Fotosynthese und generell chemischen Reaktionen oder sei es in der Entstehung von Farbe und überhaupt in unserem Sehen.
Eine Ameise auf einem Elefanten
Rima Schüssler, heute Postdoktorandin am Max-Planck-Institut für Kernphysik, hat Pentatrap seit ihrer Masterarbeit 2014 mit aufgebaut. Sie ist Erstautorin einer Arbeit über eine unerwartete Entdeckung, die in einer Zusammenarbeit am Max-Planck-PTB-Riken-Centers gemacht wurde: In Rhenium gibt es einen bislang unentdeckten elektronischen Quantenzustand mit besonderen Eigenschaften. Für die unglaubliche Empfindlichkeit, mit der Pentatrap den Sprung eines Elektrons in diesen Quantenzustand über die Massenänderung eines Rheniumatoms entdecken konnte, hat Rima Schüssler einen griffigen Vergleich parat: „Durch Wiegen eines sechs Tonnen schweren Elefanten könnten wir feststellen, ob eine zehn Milligramm leichte Ameise auf ihm herumkrabbelt.“
Pentatrap besteht aus fünf sogenannten Penningfallen. Damit eine solche Falle ein Atom wiegen kann, muss es elektrisch geladen sein, also zu einem Ion werden. Dem Rhenium wurden dazu sogar 29 seiner 75 Elektronen weggenommen, weshalb es sehr stark geladen ist, was die Messgenauigkeit steigert. Die Falle fängt dieses hochgeladene Rheniumion in einer Kombination aus einem Magnetfeld und einem speziell geformten elektrischen Feld ein. Darin läuft es in einer Kreisbahn um, die komplex in sich verschraubt ist. Im Prinzip kann man sich das wie eine Kugel an einem Seil vorstellen, die man in der Luft rotieren lässt. Tut man dies mit immer gleicher Kraft, dann rotiert eine schwerere Kugel langsamer als eine leichtere.
Ein extrem langlebiger Quantenzustand in Rhenium
In Pentatrap liefen zwei Rhenium-Ionen abwechselnd in den übereinanderliegenden Fallen um. Ein Ion befand sich im energetisch niedrigsten Quantenzustand. Im zweiten Ion wurde bei seiner Erzeugung ein Elektron durch Zufuhr von Energie zufällig in einen höheren Zustand angeregt – gewissermaßen war es die aufgezogene Uhr. Durch die gespeicherte Energie wurde es minimal schwerer und lief damit langsamer um als das erste Ion. Pentatrap zählt die Anzahl der Umläufe pro Zeiteinheit präzise mit, und die Differenz der Umlaufzahlen ergab den Gewichtszuwachs.
Mit dieser Methode entdeckte das Team im Rhenium einen extrem langlebigen Quantenzustand. Er ist metastabil, das heißt, er zerfällt nach einer gewissen Lebensdauer. Diese liegt aber bei enormen 130 Tagen, haben Theoretiker des Instituts um Zoltán Harman und Christoph H. Keitel, der Universität Heidelberg und vom Laboratoire Kastler Brossel in Paris errechnet. Es zeigte sich auch, dass die Lage des Quantenzustands sehr gut mit Modellrechnungen mit modernsten quantenmechanischen Methoden übereinstimmt.
Mögliche Anwendung in zukünftigen Atomuhren
Solche angeregten elektronischen Zustände in hochgeladenen Ionen sind für Grundlagenforschung interessant, aber auch für eine mögliche Anwendung in zukünftigen Atomuhren, wie sie die Arbeitsgruppe um José Crespo López-Urrutia am Institut in Kooperation mit der Physikalisch-Technischen Bundesanstalt (PTB) erforscht. Für diese ist der metastabile Zustand in Rhenium aus mehreren Gründen attraktiv. Zum einen entspricht er wegen seiner Langlebigkeit einer scharfen Umlauffrequenz des Elektrons um den Atomkern. Zum andern kann das Elektron mit weichem Röntgenlicht zum Sprung in diesen Quantenzustand angeregt werden. Im Prinzip könnte eine solche Uhr schneller und damit noch genauer ticken als die derzeitige Generation optischer Atomuhren. Allerdings ist es nach Ansicht von Ekkehard Peik, der an der PTB den Bereich „Zeit und Frequenz“ leitet und an der Arbeit nicht beteiligt war, noch zu früh für Spekulationen, ob sich die Entdeckung für eine neue Generation von Atomuhren eignen könnte.
„Diese neue Methode zur Entdeckung langlebiger Quantenzustände ist aber spektakulär“, betont der Physiker. Er könnte sich vorstellen, dass mit solchen neuen Quantenzuständen arbeitende Atomuhren erst einmal ein neues Testfeld für die Grundlagenforschung bieten könnten. Weil den Rheniumionen viele sich gegenseitig abschirmende Elektronen fehlen, spüren die übriggebliebenen Elektronen das elektrische Feld des Atomkerns besonders stark. Folglich rasen sie mit so hohen Geschwindigkeiten um den Kern herum, dass ihre Bewegung mit Einsteins Spezieller Relativitätstheorie beschrieben werden muss. Mit der neuen Atomwaage ließe sich auch hochpräzise testen, ob die Spezielle Relativitätstheorie und die Quantentheorie hier so zusammenspielen, wie dies die Theorie bislang beschreibt.
Ganz allgemein bietet die neue Atomwaage einen neuen Zugang zum quantenhaften Innenleben größerer Atome. Da diese aus vielen Teilchen – Elektronen, Protonen und Neutronen – bestehen, lassen sie sich nicht exakt berechnen. Daher beruhen die Atommodelle der Theorie zwangsweise auf Vereinfachungen, und diese können nun extrem genau überprüft werden. Darüber hinaus gibt es auch schon die Idee, solche Atome als Sonden für die Suche nach unbekannten Teilchen zu benutzen, die sich allein über die extrem schwache Gravitationskraft bemerkbar machen. Diese Dunkle Materie ist eines der größten, ungelösten Rätsel der Physik.
Auf dem Weg zu neuer Physik
Ein wichtiger Schritt in Richtung der Erschließung neuer Physik mit atomphysikalischen Methoden wurde ebenfalls mit PENTATRAP erreicht [Phys. Rev. Lett. 124, 113001]. Hierbei führten die Heidelberger Forscher Massenmessungen an einer Kette von fünf Paaren von Xenon-Isotopen durch. Mittels hochauflösender Laserspektroskopie an ähnlichen Ketten anderer Elemente wie Calcium und Ytterbium kann über die geringfügigen Energiedifferenzen (Isotopieverschiebung), in die die Massenmessungen eingehen, auf einen linearen Zusammenhang geschlossen werden. Nichtlineare Abweichungen davon können jedoch ein Indiz für neue Physik sein (weitere fundamentale Wechselwirkungen, neue Teilchen, Dunkle Materie), die sich bei extrem genauer Beobachtung manifestiert – eine Alternative zu Hochenergieexperimenten. Auch hier ist die enge Kooperation mit der Theorie (Gruppe um Zoltan Harman am MPIK) zu betonen. Die direkte Messung der Bindungsenergie eines Elektrons in einem hochgeladenen Ion zeigte eine sehr gute Übereinstimmung mit relativistischen Atomstrukturrechnungen. Dies schafft die Grundlage u. a. für zukünftige hochpräzise Tests der Quantenelektrodynamik.
(RW/BF)
Anmerkung: Diese Forschung wurde durch den Advanced Grant “FunI” des Europäischen Forschungsrats gefördert.
________________________________________________________________________________
Originalpublikation
Schüssler, R. X., H. Bekker, M. Braß, H. Cakir, J. R. Crespo López-Urrutia, M. Door, P. Filianin, Z. Harman, M. W. Haverkort, W. J. Huang, P. Indelicato, C. H. Keitel, C. M. König, K. Kromer, M. Müller, Y. N. Novikov, A. Rischka, C. Schweiger, S. Sturm, S. Ulmer, S. Eliseev, K. Blaum: Detection of metastable electronic states by Penning trap mass spectrometry, Nature 581, 42–46 (2020), (DOI: 10.1038/s41586-020-2221-0).
________________________________________________________________________________
Weblinks
PENTATRAP-Projekt am MPIK
Ionic Quantum Dynamics and High-Precision Theory
________________________________________________________________________________
Kontakt
Dr. Rima Schüssler
Tel.: +49 6221 516-271
rima.schuessler@mpi-hd.mpg.de
Prof. Dr. Klaus Blaum
Tel.: +49 6221 516-851
klaus.blaum@mpi-hd.mpg.de
Abstract:
State-of-the-art optical clocks1 achieve precisions of 10−18 or better using ensembles of atoms in optical lattices2,3 or individual ions in radio-frequency traps4,5. Promising candidates for use in atomic clocks are highly charged ions6 (HCIs) and nuclear transitions7, which are largely insensitive to external perturbations and reach wavelengths beyond the optical range8 that are accessible to frequency combs9. However, insufficiently accurate atomic structure calculations hinder the identification of suitable transitions in HCIs. Here we report the observation of a long-lived metastable electronic state in an HCI by measuring the mass difference between the ground and excited states in rhenium, providing a non-destructive, direct determination of an electronic excitation energy. The result is in agreement with advanced calculations. We use the high-precision Penning trap mass spectrometer PENTATRAP to measure the cyclotron frequency ratio of the ground state to the metastable state of the ion with a precision of 10−11—an improvement by a factor of ten compared with previous measurements10,11. With a lifetime of about 130 days, the potential soft-X-ray frequency reference at 4.96 × 1016 hertz (corresponding to a transition energy of 202 electronvolts) has a linewidth of only 5 × 10−8 hertz and one of the highest electronic quality factors (1024) measured experimentally so far. The low uncertainty of our method will enable searches for further soft-X-ray clock transitions8,12 in HCIs, which are required for precision studies of fundamental physics6.
Schüssler, R. X., H. Bekker, M. Braß, H. Cakir, J. R. Crespo López-Urrutia, M. Door, P. Filianin, Z. Harman, M. W. Haverkort, W. J. Huang, P. Indelicato, C. H. Keitel, C. M. König, K. Kromer, M. Müller, Y. N. Novikov, A. Rischka, C. Schweiger, S. Sturm, S. Ulmer, S. Eliseev, K. Blaum: Detection of metastable electronic states by Penning trap mass spectrometry, Nature 581, 42–46 (2020), (DOI: 10.1038/s41586-020-2221-0).
https://www.nature.com/articles/s41586-020-2221-0
Related to Project B01, B02
Abstract:
We propose that neuromorphic computing can perform quantum operations. Spiking neurons in the active or silent states are connected to the two states of Ising spins. A quantum density matrix is constructed from the expectation values and correlations of the Ising spins. As a step towards quantum computation we show for a two qubit system that quantum gates can be learned as a change of parameters for neural network dynamics.
https://arxiv.org/abs/2005.01533
Related to Project: B01
Abstract:
We study the dynamics of a three-mode bosonic system with mode-changing interactions. For large mode occupations the short-time dynamics is well described by classical mean-field equations allowing us to study chaotic dynamics in the classical system and its signatures in the corresponding quantum dynamics. By introducing a symmetry-breaking term we tune the classical dynamics from integrable to strongly chaotic, which we demonstrate by calculating Poincaré sections and Lyapunov exponents. The corresponding quantum system features level statistics that change from Poissonian in the integrable to Wigner-Dyson in the chaotic case. We investigate the behavior of out-of-time-ordered correlators (OTOCs), specifically the squared commutator, for initial states located in regular and chaotic regions of the classical mixed phase space and find marked differences between the two cases. The short-time behavior is well captured by semi-classical truncated Wigner simulations directly relating these features to properties of the underlying classical mean-field dynamics. We discuss a possible experimental realization of this model system in a Bose-Einstein condensate of rubidium atoms, which allows reversing the sign of the Hamiltonian required for measuring OTOCs.
M. Rautenberg and M. Gärttner, “Classical and quantum chaos in a three-mode bosonic system”,
Phys. Rev. A 101, 053604 (2020)
https://journals.aps.org/pra/abstract/10.1103/PhysRevA.101.053604
Related to Project A04
Press Release Nr 26/2020, 24 April 2020
The fundamental laws of physics are based on symmetries that, among other things, determine the interactions between charged particles. Using ultracold atoms, researchers at Heidelberg University have experimentally constructed the symmetries of quantum electrodynamics. They hope to gain new insights for implementing future quantum technologies that can simulate complex physical phenomena. The results of the study were published in the journal “Science”.
The theory of quantum electrodynamics deals with the electromagnetic interaction between electrons and light particles. It is based on so-called U(1) symmetry, which for instance specifies the movement of particles. With their experiments, the Heidelberg physicists, under the direction of Junior Professor Dr Fred Jendrzejewski, seek to advance the efficient investigation of this complex physical theory. They recently succeeded in experimentally realising one elementary building block. “We see the results of our research as a major step towards a platform built from a chain of properly connected building blocks for a large-scale implementation of quantum electrodynamics in ultracold atoms,” explains Prof. Jendrzejewski, who directs an Emmy Noether group at Heidelberg University’s Kirchhoff Institute for Physics.
According to the researchers, one possible application would be developing large-scale quantum devices to simulate complex physical phenomena that cannot be studied with particle accelerators. The elementary building block developed for this study could also benefit the investigation of problems in materials research, such as in strongly interacting systems that are difficult to calculate.
The research by the Heidelberg scientists was conducted as part of the “Isolated quantum systems and universality under extreme conditions” (ISOQUANT) Collaborative Research Centre funded by the German Research Foundation.
________________________________________________________________________________
Original publication:
A. Mil, T. V. Zache, A. Hegde, A. Xia, R. P. Bhatt, M. K. Oberthaler, Ph. Hauke, J. Berges, F. Jendrzejewski: A scalable realization of local U(1) gauge invariance in cold atomic mixtures, Science 06 Mar 2020: Vol. 367, Issue 6482, pp. 1128-1130
DOI: 10.1126/science.aaz5312
________________________________________________________________________________
Jun. Prof. Dr. Fred Jendrzejewski
Kirchhoff-Institut für Physik
Telefon (06221) 54-5182
fnj@kip.uni-heidelberg.de
Universität Heidelberg
Kommunikation und Marketing
Pressestelle, Telefon (06221) 54-2311
presse@rektorat.uni-heidelberg.de
Press Release Nr 26/2020, 24 April 2020
The fundamental laws of physics are based on symmetries that, among other things, determine the interactions between charged particles. Using ultracold atoms, researchers at Heidelberg University have experimentally constructed the symmetries of quantum electrodynamics. They hope to gain new insights for implementing future quantum technologies that can simulate complex physical phenomena. The results of the study were published in the journal “Science”.
The theory of quantum electrodynamics deals with the electromagnetic interaction between electrons and light particles. It is based on so-called U(1) symmetry, which for instance specifies the movement of particles. With their experiments, the Heidelberg physicists, under the direction of Junior Professor Dr Fred Jendrzejewski, seek to advance the efficient investigation of this complex physical theory. They recently succeeded in experimentally realising one elementary building block. “We see the results of our research as a major step towards a platform built from a chain of properly connected building blocks for a large-scale implementation of quantum electrodynamics in ultracold atoms,” explains Prof. Jendrzejewski, who directs an Emmy Noether group at Heidelberg University’s Kirchhoff Institute for Physics.
According to the researchers, one possible application would be developing large-scale quantum devices to simulate complex physical phenomena that cannot be studied with particle accelerators. The elementary building block developed for this study could also benefit the investigation of problems in materials research, such as in strongly interacting systems that are difficult to calculate.
The research by the Heidelberg scientists was conducted as part of the “Isolated quantum systems and universality under extreme conditions” (ISOQUANT) Collaborative Research Centre funded by the German Research Foundation.
________________________________________________________________________________
Original publication:
A. Mil, T. V. Zache, A. Hegde, A. Xia, R. P. Bhatt, M. K. Oberthaler, Ph. Hauke, J. Berges, F. Jendrzejewski: A scalable realization of local U(1) gauge invariance in cold atomic mixtures, Science 06 Mar 2020: Vol. 367, Issue 6482, pp. 1128-1130
DOI: 10.1126/science.aaz5312
________________________________________________________________________________
Jun. Prof. Dr. Fred Jendrzejewski
Kirchhoff-Institut für Physik
Telefon (06221) 54-5182
fnj@kip.uni-heidelberg.de
Universität Heidelberg
Kommunikation und Marketing
Pressestelle, Telefon (06221) 54-2311
presse@rektorat.uni-heidelberg.de
Pressemitteilung Nr. 26/2020, 24. April 2020
Die fundamentalen Gesetze der Physik basieren auf Symmetrien, die unter anderem die Wechselwirkungen zwischen geladenen Teilchen bestimmen. Forscher der Universität Heidelberg haben mithilfe ultrakalter Atome die Symmetrien der Quantenelektrodynamik experimentell konstruiert. Sie erhoffen sich neue Erkenntnisse für die Realisierung zukünftiger Quantentechnologien, mit denen komplexe physikalische Phänomene simuliert werden können. Die Ergebnisse der Studie wurden in der Fachzeitschrift „Science“ veröffentlicht.
In der Theorie der Quantenelektrodynamik geht es um die elektromagnetische Wechselwirkung von Elektronen mit Lichtteilchen. Sie basiert auf der sogenannten U(1)-Symmetrie, die etwa die Bewegung der Teilchen vorgibt. Mit ihren Experimenten wollen die Heidelberger Physiker unter der Leitung von Juniorprofessor Dr. Fred Jendrzejewski zu einer effizienten Untersuchung dieser komplexen physikalischen Theorie beitragen. Ihnen ist es jetzt gelungen, dafür experimentell einen elementaren Baustein zu realisieren. „Wir sehen unsere Forschungsergebnisse als zentralen Schritt hin zu einer Plattform bestehend aus einer Kette korrekt zusammengesetzter Bausteine, die einer groß angelegten Implementierung der Quantenelektrodynamik in ultrakalten Atomen dienen soll“, erläutert Prof. Jendrzejewski, der am Kirchhoff-Institut für Physik der Universität Heidelberg eine Emmy Noether-Gruppe leitet.
Eine mögliche Anwendung sehen die Wissenschaftler in der Entwicklung von Quantengroßgeräten zur Simulation komplexer physikalischer Phänomene, die nicht mit Teilchenbeschleunigern beobachtet werden können. Auch für die Untersuchung von Problemen in der Materialforschung, zum Beispiel bei stark wechselwirkenden Systemen, die schwer zu berechnen sind, könnte der von den Heidelberger Wissenschaftlern entwickelte Baustein von Nutzen sein.
Die Arbeiten der Heidelberger Wissenschaftler wurden im Rahmen des Sonderforschungsbereichs „Isolierte Quantensysteme und Universalität unter extremen Bedingungen“ (ISOQUANT) von der Deutschen Forschungsgemeinschaft gefördert.
________________________________________________________________________________
Originalpublikation:
A. Mil, T. V. Zache, A. Hegde, A. Xia, R. P. Bhatt, M. K. Oberthaler, Ph. Hauke, J. Berges, F. Jendrzejewski: A scalable realization of local U(1) gauge invariance in cold atomic mixtures, Science 06 Mar 2020: Vol. 367, Issue 6482, pp. 1128-1130
DOI: 10.1126/science.aaz5312
________________________________________________________________________________
Jun. Prof. Dr. Fred Jendrzejewski
Kirchhoff-Institut für Physik
Telefon (06221) 54-5182
fnj@kip.uni-heidelberg.de
Universität Heidelberg
Kommunikation und Marketing
Pressestelle, Telefon (06221) 54-2311
presse@rektorat.uni-heidelberg.de
Pressemitteilung Nr. 26/2020, 24. April 2020
Die fundamentalen Gesetze der Physik basieren auf Symmetrien, die unter anderem die Wechselwirkungen zwischen geladenen Teilchen bestimmen. Forscher der Universität Heidelberg haben mithilfe ultrakalter Atome die Symmetrien der Quantenelektrodynamik experimentell konstruiert. Sie erhoffen sich neue Erkenntnisse für die Realisierung zukünftiger Quantentechnologien, mit denen komplexe physikalische Phänomene simuliert werden können. Die Ergebnisse der Studie wurden in der Fachzeitschrift „Science“ veröffentlicht.
In der Theorie der Quantenelektrodynamik geht es um die elektromagnetische Wechselwirkung von Elektronen mit Lichtteilchen. Sie basiert auf der sogenannten U(1)-Symmetrie, die etwa die Bewegung der Teilchen vorgibt. Mit ihren Experimenten wollen die Heidelberger Physiker unter der Leitung von Juniorprofessor Dr. Fred Jendrzejewski zu einer effizienten Untersuchung dieser komplexen physikalischen Theorie beitragen. Ihnen ist es jetzt gelungen, dafür experimentell einen elementaren Baustein zu realisieren. „Wir sehen unsere Forschungsergebnisse als zentralen Schritt hin zu einer Plattform bestehend aus einer Kette korrekt zusammengesetzter Bausteine, die einer groß angelegten Implementierung der Quantenelektrodynamik in ultrakalten Atomen dienen soll“, erläutert Prof. Jendrzejewski, der am Kirchhoff-Institut für Physik der Universität Heidelberg eine Emmy Noether-Gruppe leitet.
Eine mögliche Anwendung sehen die Wissenschaftler in der Entwicklung von Quantengroßgeräten zur Simulation komplexer physikalischer Phänomene, die nicht mit Teilchenbeschleunigern beobachtet werden können. Auch für die Untersuchung von Problemen in der Materialforschung, zum Beispiel bei stark wechselwirkenden Systemen, die schwer zu berechnen sind, könnte der von den Heidelberger Wissenschaftlern entwickelte Baustein von Nutzen sein.
Die Arbeiten der Heidelberger Wissenschaftler wurden im Rahmen des Sonderforschungsbereichs „Isolierte Quantensysteme und Universalität unter extremen Bedingungen“ (ISOQUANT) von der Deutschen Forschungsgemeinschaft gefördert.
________________________________________________________________________________
Originalpublikation:
A. Mil, T. V. Zache, A. Hegde, A. Xia, R. P. Bhatt, M. K. Oberthaler, Ph. Hauke, J. Berges, F. Jendrzejewski: A scalable realization of local U(1) gauge invariance in cold atomic mixtures, Science 06 Mar 2020: Vol. 367, Issue 6482, pp. 1128-1130
DOI: 10.1126/science.aaz5312
________________________________________________________________________________
Jun. Prof. Dr. Fred Jendrzejewski
Kirchhoff-Institut für Physik
Telefon (06221) 54-5182
fnj@kip.uni-heidelberg.de
Universität Heidelberg
Kommunikation und Marketing
Pressestelle, Telefon (06221) 54-2311
presse@rektorat.uni-heidelberg.de
Abstract:
Based on transport equations we argue that the chiral dynamics in heavy-ion collisions at high collision energies effectively decouples from the thermal physics of the fireball. With full decoupling at LHC energies the chiral condensate relaxes to its vacuum expectation value on a much shorter time scale than the typical evolution time of the fluid dynamical fields and their fluctuations. In particular, the net-baryon density remains coupled to the bulk evolution at all collision energies. As the mass scales of the hadrons are controlled by the chiral condensate, it is reasonable to employ vacuum masses in the statistical description of the hadron production at the chemical freeze-out for high collision energies. We predict that at lower collision energies the coupling of the chiral condensate to the thermal medium gradually increases with consequences for the related hadronic masses. A new estimate for the location of the freeze-out curve takes these effects into account.
M. Bluhm, M. Nahrgang, J. M. Pawlowski: Locating the freeze-out curve in heavy-ion collisions, arXiv:2004.08608, 2020.
https://arxiv.org/abs/2004.08608
Related to Project A02, A01, C05, C06
Abstract:
Quantum many-body systems with exact local gauge symmetries exhibit rich out-of-equilibrium physics such as constrained dynamics and disorder-free localization. In a joint submission, we present evidence of \textit{staircase prethermalization} in a Z2 lattice gauge theory subjected to a small breaking of gauge invariance. Here, we consolidate this finding and the associated emergent nonperturbative timescales analytically and numerically. By means of a Magnus expansion, we demonstrate how exact resonances between different gauge-invariant supersectors are the main reason behind the emergence of staircase prethermalization. Furthermore, we showcase the robustness of our conclusions against various initial conditions including different system sizes, matter fillings, and gauge-invariance sectors, in addition to various boundary conditions, such as different maximal on-site matter occupations. We also elaborate on how our conclusions are unique to local-symmetry models and why they break down in the case of global-symmetry breaking. We moreover extend our results to U(1) lattice gauge theories, illustrating the generality of our findings. Our work offers an analytic footing into the constrained dynamics of lattice gauge theories and provides proof of a certain intrinsic robustness of gauge-theory dynamics to errors in experimental settings.
C. Halimeh, P. Hauke: Origin of staircase prethermalization in lattice gauge theories, arXiv:2004.07254, 2020.
https://arxiv.org/abs/2004.07254
Related to Project B04
Abstract:
The dynamics of lattice gauge theories is characterized by an abundance of local symmetry constraints. Although errors that break gauge symmetry appear naturally in NISQ-era quantum simulators, their influence on the gauge-theory dynamics is insufficiently investigated. As we show, a small gauge breaking of strength λ induces a staircase of long-lived prethermal plateaus. The number of prethermal plateaus increases with the number of matter fields L, with the last plateau being reached at a timescale λ−L/2, showing an intimate relation of the concomitant slowing down of dynamics with the number of local gauge constraints. Our results bode well for NISQ quantum devices, as they indicate that the proliferation timescale of gauge-invariance violation is counterintuitively delayed exponentially in system size.
C. Halimeh, P. Hauke: Staircase prethermalization and constrained dynamics in lattice gauge theories, arXiv:2004.07248, 2020.
https://arxiv.org/abs/2004.07248
Related to Project B04
Abstract:
Partial bosonization of the two-dimensional Hubbard model focuses the functional renormalization flow on channels in which interactions become strong and local order sets in. We compare the momentum structure of the four-fermion vertex, obtained on the basis of a patching approximation, to an effective bosonic description. For parameters in the antiferromagnetic phase near the onset of local antiferromagnetic order, the interaction of the electrons is indeed well described by the exchange of collective bosonic degrees of freedom. The residual four-fermion vertex after the subtraction of the bosonic-exchange contribution is small. We propose that similar partial bosonization techniques can improve the accuracy of renormalization flow studies also for the case of competing order.
JT. Denz, M. Mitter, J. M. Pawlowski, C. Wetterich, and M. Yamada, “Partial bosonization for the two-dimensional Hubbard model”, Phys. Rev. B 101, 155115 (2020).
https://journals.aps.org/prb/abstract/10.1103/PhysRevB.101.155115
Related to Project: C01
Abstract:
Two-particle azimuthal correlations have been measured in neutral current deep inelastic ep scattering with virtuality Q2> 5 GeV2 at a centre-of-mass energy s√ = 318 GeV recorded with the ZEUS detector at HERA. The correlations of charged particles have been measured in the range of laboratory pseudorapidity −1.5 < η < 2.0 and transverse momentum 0.1 < pT< 5.0 GeV and event multiplicities Nch up to six times larger than the average 〈Nch〉 ≈ 5. The two-particle correlations have been measured in terms of the angular observables cn{2} = 〈〈cosnΔφ〉〉, where n is between 1 and 4 and ∆φ is the relative azimuthal angle between the two particles. Comparisons with available models of deep inelastic scattering, which are tuned to reproduce inclusive particle production, suggest that the measured two-particle correlations are dominated by contributions from multijet production. The correlations observed here do not indicate the kind of collective behaviour recently observed at the highest RHIC and LHC energies in high-multiplicity hadronic collisions.
[ZEUS collaboration] S. Floerchinger, S. Masciocchi, I. Selyuzhenkov et al., „Two-particle azimuthal
correlations as a probe of collective behaviour in deep inelastic ep scattering at HERA“,
JHEP 2020, 070 (2020).
https://link.springer.com/article/10.1007%2FJHEP04%282020%29070
Related to Project: C06, ABC
Abstract:
We raise the power from a commercial 10 W frequency comb inside an enhancement cavity and perform multi-photon ionization of gas-phase atoms at 100 MHz for the first time, to the best of our knowledge. An intra-cavity velocity-map-imaging setup collects electron-energy spectra of xenon at rates several orders of magnitude higher than those of conventional laser systems. Consequently, we can use much lower intensities ∼1012W/cm2 without increasing acquisition times above just a few seconds. The high rate and coherence of the stabilized femtosecond pulses are known to be transferred to the actively stabilized cavity and will allow studying purely perturbative multi-photon effects, paving the road towards a new field of precision tests in nonlinear physics.
J. Nauta, J.-H. Oelmann, A. Ackermann, P. Knauer, R. Pappenberger, A. Borodin, I. S. Muhammad, H. Ledwa, T. Pfeifer, and J. R. C. López-Urrutia, “100MHz frequency comb for lowintensity multi-photon studies: intra-cavity velocity-map imaging of xenon”, Opt. Lett. 45, 2156 (2020)
https://www.osapublishing.org/ol/abstract.cfm?uri=ol-45-8-2156
Related to Project: B01
Abstract:
Using the Brink-Axel hypothesis we derive the rate R for nuclear dipole excitation by a laser pulse carrying N≫1 photons with average energy ℏω0≈5 MeV. As expected R∝(ℏω0)3. The rate is also proportional to the aperture α of the laser pulse. Perhaps less expected is the fact that R∝N, irrespective of the degree of coherence of the laser pulse. The expression for R, derived for a nearly stationary laser pulse, is valid also for short times and can, thus, be used in simulations via rate equations of multiple nuclear dipole excitations by a single pulse. The explicit dependence of R on the parameters of the laser pulse and on nuclear parameters given in the paper should help to optimize experiments on laser-nucleus reactions.
A. Pálffy, P.-G. Reinhard, H. A. Weidenmüller: Rate for Laser-Induced Nuclear Dipole Absorption, Phys. Rev. C 101, 034619 (2020), (DOI: 10.1103/PhysRevC.101.034619)
https://journals.aps.org/prc/abstract/10.1103/PhysRevC.101.034619
Related to Project B02
Abstract:
Time-domain interferometry (TDI) is a method to probe space-time correlations among particles in condensed matter systems. Applying TDI to quantum systems raises the general question, whether two-time correlations can be reliably measured without adverse impact of the measurement backaction onto the dynamics of the system. Here, we show that a recently developed quantum version of TDI (QTDI) indeed can access the full quantum-mechanical two-time correlations without backaction. We further generalize QTDI to weak classical continuous-mode coherent input states, alleviating the need for single-photon input fields. Finally, we interpret our results by splitting the space-time correlations into two parts. While the first one is associated to projective measurements and thus insensitive to backaction, we identify the second contribution as arising from the coherence properties of the state of the probed target system, such that it is perturbed or even destroyed by measurements on the system.
S. Castrignano, J. Evers: Backaction-free measurement of quantum correlations via quantum time-domain interferometry, arXiv:2003.11900, 2020.
https://arxiv.org/abs/2003.10338
Related to Project B02
Abstract:
The procedure for the calculation of the self-energy-corrected wave function of the bound electron in the field of the nucleus is discussed. We present the related formulas and discuss the numerical difficulties and the methods used to overcome them. The results of the calculation are presented for a wide range of ions. Possible applications of the numerically obtained wave functions are discussed.
N. S. Oreshkina, H. Cakir, B. Sikora, V. A. Yerokhin, V. Debierre, Z. Harman, and C. H. Keitel,
“Self-energy-corrected dirac wave functions for advanced qed calculations in highly charged
ions”, Phys. Rev. A 101, 032511 (2020).
https://journals.aps.org/pra/abstract/10.1103/PhysRevA.101.032511
Related to Project B02
Abstract:
We discuss the phase structure of QCD for Nf=2 and Nf=2+1 dynamical quark flavors at finite temperature and baryon chemical potential. It emerges dynamically from the underlying fundamental interactions between quarks and gluons in our work. To this end, starting from the perturbative high-energy regime, we systematically integrate out quantum fluctuations toward low energies by using the functional renormalization group. By dynamically hadronizing the dominant interaction channels responsible for the formation of light mesons and quark condensates, we are able to extract the phase diagram for μB/T≲6. We find a critical endpoint at (TCEP,μBCEP)=(107,635) MeV. The curvature of the phase boundary at small chemical potential is κ=0.0142(2), computed from the renormalized light chiral condensate Δl,R. Furthermore, we find indications for an inhomogeneous regime in the vicinity and above the chiral transition for μB≳417 MeV. Where applicable, our results are in very good agreement with the most recent lattice results. We also compare to results from other functional methods and phenomenological freeze-out data. This indicates that a consistent picture of the phase structure at finite baryon chemical potential is beginning to emerge. The systematic uncertainty of our results grows large in the density regime around the critical endpoint and we discuss necessary improvements of our current approximation toward a quantitatively precise determination of QCD phase diagram.
W.-j. Fu, J. M. Pawlowski, and F. Rennecke, “QCD phase structure at finite temperature and density”, Phys. Rev. D 101, 054032 (2020).
https://journals.aps.org/prd/abstract/10.1103/PhysRevD.101.054032
Related to Project: A01, A02, B03, C05, C06
Abstract:
The study of critical properties of systems with long-range interactions has attracted, in recent decades, a continuing interest and motivated the development of several analytical and numerical techniques, in particular in connection with spin models. From the point of view of the investigation of their criticality, a special role is played by systems in which the interactions are long-range enough that their universality class is different from the short-range case and, nevertheless, they maintain the extensivity of thermodynamical quantities. Such interactions are often called weak long-range. In this paper we focus on the study of the critical behaviour of spin systems with weak-long range couplings using functional renormalization group, and we review their remarkable properties. For the sake of clarity and self-consistency, we start from classical spin models and we then move to quantum spin systems.
N. Defenu, A. Codello, S. Ruffo, and A. Trombettoni, “Criticality of Spin Systems with Weak Long-Range Interactions”, J. Phys. A: Math. Theor. 53, 143001 (2020).
https://iopscience.iop.org/article/10.1088/1751-8121/ab6a6c
Related to Project C02
Abstract:
We present a depletion imaging technique to map out the spatial and temporal dependency of the density distribution of an ultracold gas of Rydberg atoms. Locally resolved absorption depletion, observed through differential ground state absorption imaging of a 87Rb cloud in presence and absence of pre-excited Rydberg atoms, reveals their projected two-dimensional distribution. By employing a closed two-level optical transition uncoupled from the Rydberg state, the highly excited atoms are preserved during imaging. We measure the excitation dynamics of the |48S⟩ state of 87Rb, observing a saturation of the two-dimensional Rydberg density. Such outcome can be explained by the Rydberg blockade effect which prevents resonant excitation of close-by Rydberg atoms due to strong dipolar interactions. By combining the superatom description, where atoms within a blockade radius are represented as collective excitations, with a Monte Carlo sampling, we can quantitatively model the observed excitation dynamics and infer the full three-dimensional distribution of Rydberg atoms, that can serve as a starting point for quantum simulation of many-body dynamics involving Rydberg spin systems
M. Ferreira-Cao, V. Gavryusev, T. Franz, R. Ferracini Alves, A. Signoles, G. Zürn, M. Weidemüller: Depletion Imaging of Rydberg atoms in cold atomic gases, J. Phys. B At. Mol. Opt. Phys. 53, 84004 (2020)
https://iopscience.iop.org/article/10.1088/1361-6455/ab7427
Related to Project A05
With today`s notice the Girls’Day und Boys’Day 2020 was cancelled due to the current development of COVID19. There will be no alternative date for 2020. We will defintitely offer our Girls`Day program “Physik hautnah!” for next year! Girls´Day will be then be on 22 April 2021.
Die bundesweiten Aktionstage zur klischeefreien Berufsorientierung für Jungen und Mädchen sind aufgrund der aktuellen Corona-Situation für 2020 abgesagt. Einen Ersatztermin wird es nicht geben. In den letzten zwei Wochen sind in der Bundeskoordinierungsstelle bereits zahlreiche Absagen von beteiligten Unternehmen und Institutionen eingegangen.
Die Absage des Aktionstages am 26. März 2020 gilt für ganz Deutschland. Viele hatten bereits ihre Veranstaltung zur Berufserkundung über die Bundeskoordinierungsstelle abgesagt.
“Mit der Entscheidung schließen wir uns den bundesweiten Vorsichtsmaßnahmen an. Wir bedauern dies, vor allem für die bereits angemeldeten Schülerinnen und Schüler. Den Veranstalterinnen und Veranstaltern danken wir für ihr Engagement und Verständnis”, so die beiden Projektleiterinnen Elisabeth Schöppner und Romy Stühmeier.
Alle über das Girls’Day-Radar und Boys’Day-Radar registrierten Schülerinnen und Schüler sowie teilnehmende Unternehmen und Institutionen werden jetzt von der Bundeskoordinierungsstelle über die Absage informiert.
Das Einstellen von Angeboten und die Anmeldung zum Girls’Day und Boys’Day über die Websites girls-day.de und boys-day.de ist ab sofort nicht mehr möglich.
Der nächste Girls’Day und Boys’Day findet am 22. April 2021 statt.
Abstract:
First ever measurements of the ratios of free cyclotron frequencies of heavy, highly charged ions with Z>50 with relative uncertainties close to 10−11 are presented. Such accurate measurements have become realistic due to the construction of the novel cryogenic multi-Penning-trap mass spectrometer PENTATRAP. Based on the measured frequency ratios, the mass differences of five pairs of stable xenon isotopes, ranging from 126Xe to 134Xe, have been determined. Moreover, the first direct measurement of an electron binding energy in a heavy highly charged ion, namely of the 37th atomic electron in xenon, with an uncertainty of a few eV is demonstrated. The obtained value agrees with the calculated one using two independent, different implementations of the multiconfiguration Dirac-Hartree-Fock method. PENTATRAP opens the door to future measurements of electron binding energies in highly charged heavy ions for more stringent tests of bound-state quantum electrodynamics in strong electromagnetic fields and for an investigation of the manifestation of light dark matter in isotopic chains of certain chemical elements.
A. Rischka, H. Cakir, M. Door, P. Filianin, Z. Harman, W. J. Huang, P. Indelicato, C. H. Keitel, C. M. König, K. Kromer, M. Müller, Y. N. Novikov, R. X. Schüssler, Ch. Schweiger, S. Eliseev, and K. Blaum: Mass-Difference Measurements on Heavy Nuclides with an eV/c2 Accuracy in the PENTATRAP Spectrometer, Phys. Rev. Lett. 124, 113001 (2020)
https://journals.aps.org/prl/abstract/10.1103/PhysRevLett.124.113001
Related to Project B01, B02
Abstract:
We present version 3.0 of the Mathematica package DoFun for the derivation of functional equations. In this version, the derivation of equations for correlation functions of composite operators was added. In the update, the general workflow was slightly modified taking into account experience with the previous version. In addition, various tools were included to improve the usage experience and the code was partially restructured for easier maintenance.
M. Q. Huber, A. K. Cyrol, J.M. Pawlowski: DoFun 3.0: Functional equations in mathematica, Computer Physics Communications Vol. 248 (2020), (DOI: 10.1016/j.cpc.2019.107058).
https://www.sciencedirect.com/science/article/pii/S0010465519303844?via%3Dihub
Related to Project A02, B03, C01, C02
Abstract:
We present an open-source Matlab framework, titled iFluid, for simulating the dynamics of integrable models using the theory of generalized hydrodynamics (GHD). The framework provides an intuitive interface, enabling users to define and solve problems in a few lines of code. Moreover, iFluid can be extended to encompass any integrable model, and the algorithms for solving the GHD equations can be fully customized. We demonstrate how to use iFluid by solving the dynamics of three distinct systems: (i) The quantum Newton’s cradle of the Lieb-Liniger model, (ii) a gradual field release in the XXZ-chain, and (iii) a partitioning protocol in the relativistic sinh-Gordon model.
F. S. Møller and J. Schmiedmayer, “Introducing iFluid: a numerical framework for solving
hydrodynamical equations in integrable models”, SciPost Phys. 8, 041 (2020).
https://scipost.org/10.21468/SciPostPhys.8.3.041
Related to Project A03
Abstract:
We explore the in-medium properties of heavy-quarkonium states at finite baryochemical potential and finite transverse momentum based on a modern complex-valued potential model. Our starting point is a novel, rigorous derivation of the generalized Gauss law for in-medium quarkonium, combining the nonperturbative physics of the vacuum bound state with a weak coupling description of the medium degrees of freedom. Its relation to previous models in the literature is discussed. We show that our approach is able to reproduce the complex lattice QCD heavy quark potential even in the nonperturbative regime, using a single temperature dependent parameter, the Debye mass mD. After vetting the Gauss law potential with state-of-the-art lattice QCD data, we extend it to the regime of finite baryon density and finite velocity, currently inaccessible to first principles simulations. In-medium spectral functions computed from the Gauss law potential are subsequently used to estimate the ψ′/J/ψ ratio in heavy-ion collisions at different beam energies and transverse momenta. We find qualitative agreement with the predictions from the statistical model of hadronization for the √sNN dependence and a mild dependence on the transverse momentum.
D. Lafferty, A. Rothkopf: Improved Gauss law model and in-medium heavy quarkonium at finite density and velocity, Phys. Rev. D 101 (2020) 056010, (DOI: 10.1103/PhysRevD.101.056010).
https://journals.aps.org/prd/abstract/10.1103/PhysRevD.101.056010
Related to Project C05
Abstract:
In the fundamental laws of physics, gauge fields mediate the interaction between charged particles. An example is the quantum theory of electrons interacting with the electromagnetic field, based on U(1) gauge symmetry. Solving such gauge theories is in general a hard problem for classical computational techniques. Although quantum computers suggest a way forward, large-scale digital quantum devices for complex simulations are difficult to build. We propose a scalable analog quantum simulator of a U(1) gauge theory in one spatial dimension. Using interspecies spin-changing collisions in an atomic mixture, we achieve gauge-invariant interactions between matter and gauge fields with spin- and species-independent trapping potentials. We experimentally realize the elementary building block as a key step toward a platform for quantum simulations of continuous gauge theories.
A.Mil, T. V. Zache, A. Hegde, A. Xia, R. P. Bhatt, M. K. Oberthaler, P. Hauke, J. Berges, F. Jendrzejewski: A scalable realization of local U(1) gauge invariance in cold atomic mixtures, Science 367 (2020) 6482.
Related to Project B04, A01, A04
Abstract:
We compute the far-from-equilibrium dynamics of relativistic scalar quantum fields in 3+1 space-time dimensions starting from over-occupied initial conditions. We determine universal scaling exponents and functions for two-point correlators and the four-vertex in a self-similar regime in time and space or momenta. The scaling form of the momentum-dependent four-vertex exhibits a dramatic fall-off toward low momenta. Comparing spectral functions (commutators) and statistical correlations (anticommutators) of field operators allows us to detect strong violations of the fluctuation-dissipation relation in this non-perturbative infrared regime. Based on a self-consistent expansion in the number of field components to next-to-leading order, a wide range of interaction strengths is analyzed and compared to weak-coupling estimates in effective kinetic theory and classical-statistical field theory.
L. Shen, J. Berges: Spectral, statistical and vertex functions in scalar quantum field theory far from equilibrium, Phys. Rev. D 101 (2020), (DOI: 10.1103/PhysRevD.101.056009).
https://journals.aps.org/prd/abstract/10.1103/PhysRevD.101.056009
Related to Project B03
Abstract:
We investigate a Bose-Einstein condensate in strong interaction with a single impurity particle. While this situation has received considerable interest in recent years, the regime of strong coupling remained inaccessible to most approaches due to an instability in Bogoliubov theory arising near the resonance. We present a nonlocal extension of Gross-Pitaevskii theory that is free of such divergences and does not require the use of the Born approximation in any of the interaction potentials. We find a new dynamical transition regime between attractive and repulsive polarons, where an interaction quench results in a finite number of coherent oscillations in the density profiles of the medium and in the contact parameter.
M. Drescher, M. Salmhofer: Theory of a resonantly interacting impurity in a Bose-Einstein condensate, arXiv:2003.01982 (2020)
https://arxiv.org/abs/2003.01982
Related to Project C03, C02
Abstract:
This volume is a collection of contributions for the 7-week program “Probing Nucleons and Nuclei in High Energy Collisions” that was held at the Institute for Nuclear Theory in Seattle, WA, USA, from October 1 until November 16, 2018. The program was dedicated to the physics of the Electron Ion Collider (EIC), the world’s first polarized electron-nucleon (ep) and electron-nucleus (eA) collider to be constructed in the USA. These proceedings are organized by chapters, corresponding to the weeks of the program: Week I, Generalized parton distributions; Week II, Transverse spin and TMDs; Week III, Longitudinal spin; Week IV, Symposium week; Weeks V & VI, eA collisions; Week VII, pA and AA collisions. We hope these proceedings will be useful to readers as a compilation of EIC-related science at the end of the second decade of the XXI century
A. Aidala, F. Aslan, A. Bacchetta, Ian Balitsky, Sanjin Benic, S. Bhattacharya, M. Boglione, M. Burkardt, J. Cammarota, G. A. Chirilli, C. Cocuzza, A. Courtoy, D. de Florian, P. Di Nezza, A. Dumitru, S. Fucini, K. Fukushima, Y. Furletova, L. Gamberg, O. Garcia-Montero, F. Gelis, V. Guzey, Y. Hatta, F. Hautmann, T. J. Hobbs, T. Horn, E. Iancu, S. Joosten, Z.-B. Kang, R. Kishore, Y. V. Kovchegov, P. Kroll, K. Kumericki, K. Kutak, T. Lappi, H.-W. Lin, X. Liu, S. Liuti, C. Lorcé, H. Mäntysaari, C. Marquet, Y. Makris, K. A. Mamo, Y. Mehtar-Tani, A. Metz, Z.-E. Meziani, G. A. Miller, A. Mukherjee, P. M. Nadolsky, F. I. Olness, B. Pasquini, B. Pire, C. Pisano, D. Pitonyak, M. V. Polyakov, A. Prokudin, J.-W. Qiu, M. Radici, A. Rajan, S. Rajesh, M. Rinaldi, K. Roy, C. Royon, N. Sato, M. Schlegel, G. Schnell, P. Schweitzer, S. Scopetta, R. Seidl, K. Semenov-Tian-Shansky, D. Sokhan, A. M. Stasto, L. Szymanowski, A. Tarasov, D. Triantafyllopoulos, T. Ullrich, R. Venugopalan, I. Vitev, W. Vogelsang, A. Vossen, B.-T. Wang, S. Wallon, C. Weiss, B.-W. Xiao, H.-U. Yee, Y. Zhao: Probing Nucleons and Nuclei in High Energy Collisions, arXiv:2002.12333, 2020.
https://arxiv.org/abs/2002.12333
Related to Project A01,A02, C05, C06
Abstract:
Since the incident nuclei in heavy-ion collisions do not carry strangeness, the global net strangeness of the detected hadrons has to vanish. We investigate the impact of strangeness neutrality on the phase structure and thermodynamics of QCD at finite baryon and strangeness chemical potential. To this end, we study the low-energy sector of QCD within a Polyakov loop enhanced quark-meson effective theory with 2+1 dynamical quark flavors. Non-perturbative quantum, thermal, and density fluctuations are taken into account with the functional renormalization group. We show that the impact of strangeness neutrality on thermodynamic quantities such as the equation of state is sizable.
W. Fu, J. M. Pawlowski, and F. Rennecke, “Strangeness Neutrality and QCD Thermodynamics”, SciPost Phys. Core 2, 2 (2020).
https://scipost.org/10.21468/SciPostPhysCore.2.1.002
Related to Project: A01, A02, B03, C05, C06
Abstract:
Gravitational waves (GWs) are inevitably produced by second-order terms in cosmological perturbation theory. Most notably, the so-called induced (GWs) are a window to the small scales part of the primordial spectrum of fluctuations and a key counterpart to the primordial black hole (PBH) scenario. However, semi-analytical solutions are only known for matter and radiation domination eras. In this paper, we present new analytic integral formulas for the induced GWs on subhorizon scales in a general cosmological background with a constant equation-of-state. We also discuss applications to a peaked primordial scalar power spectrum and the PBH scenario.
G. Domènech, “Induced gravitational waves in a general cosmological background”, Int. J. Mod.
Phys. D 29, 2050028 (2020).
https://www.worldscientific.com/doi/abs/10.1142/S0218271820500285
Related to Project: B01
Scientists from the Physikalisch-Technische Bundesanstalt (PTB) and the Max Planck Institute for Nuclear Physics (MPIK) have carried out pioneering optical measurements of highly charged ions with unprecedented precision. To do this, they isolated a single Ar¹³⁺ ion from an extremely hot plasma and brought it practically to rest inside an ion trap together with a laser-cooled, singly charged ion. Employing quantum logic spectroscopy on the ion pair, they have increased the relative precision by a factor of a hundred million over previous methods. This opens up the multitude of highly charged ions for novel atomic clocks and further avenues in the search for new physics. [Nature, 29.01.2020]
Highly charged ions are—although seemingly exotic—a very natural form of visible matter. All the matter in our sun and in all other stars is highly ionized, for example. In many ways, however, highly charged ions are more extreme than neutral atoms or singly charged ions. Due to their high positive charge, the outer electrons of the atomic shell are more strongly bound to the atomic nucleus. They are therefore less sensitive to perturbations by external electromagnetic fields. On the other hand, compared to neutral and singly charged atoms, the effects of special relativity and quantum electrodynamics as well as the interaction with the atomic nucleus are considerably enhanced. Highly charged ions are therefore ideal systems for accurate atomic clocks that can be used to test fundamental physics. The outer electrons in these systems serve as sensitive “quantum sensors” for effects such as previously unknown forces and fields. Since every single element of the periodic table provides as many charge states as there are electrons in the atomic shell, there exists a vast variety of atomic systems to choose from.
To date, however, established measurement techniques as used in optical atomic clocks could not be applied to highly charged ions. The main obstacle manifests already in the process of their production: a large amount of energy is required in order to remove a significant number of electrons from the atoms, and the ions then exist in the form of a plasma as hot as the Sun itself. However, the most precise and accurate experiments require the exact opposite: the lowest possible temperatures and well-controlled ambient conditions in order to reduce shifts and broadening of the spectral lines to be measured. This is hindered by the fact that highly charged ions cannot be directly laser-cooled, and conventional detection methods cannot be applied due to their atomic structure.
Physicists from the Physikalisch-Technische Bundesanstalt and the Max Planck Institute for Nuclear Physics in Heidelberg have now combined individual solutions to each of these problems in a worldwide unique experiment at the QUEST Institute for Experimental Quantum Metrology in Braunschweig. They isolated a single highly charged ion (Ar¹³⁺) from a hot plasma ion source and stored it together with a singly charged beryllium ion in an ion trap. The latter can be laser-cooled very efficiently and through the mutual electrical interaction the temperature of the entire ion pair can be reduced. Eventually, this so-called “sympathetic cooling” forms a two-ion crystal that completely “freezes” into the quantum mechanical ground state of motion at an equivalent temperature of only a few millionths of a degree above absolute zero.
Using an ultrastable laser the scientists precisely resolved the spectral structure of the Ar¹³⁺ ion in a measurement procedure similar to that used in state-of-the-art clocks. For this, they applied the concept of quantum logic, in which the spectroscopy signal is coherently transferred from the highly charged ion to the beryllium ion by means of two laser pulses. The quantum state of the beryllium ion is much easier to determine via laser excitation. “Descriptively, the beryllium ion ‘eavesdrops’ on the state of the less communicative highly charged ion and reports to us about its state”, explains Piet Schmidt, head of the collaboration. “Here, we have improved the relative precision for highly charged ions by a factor of one hundred million compared to traditional spectroscopy,” adds Peter Micke, research assistant at the QUEST Institute and first author of the paper.
Combining all these methods establishes a very general concept that can be applied to most highly charged ions. The beryllium ion can always be used as a so-called logic ion and the production process of the highly charged ions in the plasma with subsequent isolation of a single ion is independent of the choice of the atomic type and the charge state.
José Crespo, head of the group at the Max Planck Institute for Nuclear Physics, emphasizes: “This experiment opens up an unprecedented, extremely extensive area of atomic systems to be used in precision spectroscopy as well as for future clocks with special properties.” For basic research, the great variety of these new, tailored “quantum sensors” enables a promising investigation of fundamental questions: Is our standard model of particle physics complete? What is dark matter? Are fundamental constants really constant?
Original publication:
Coherent laser spectroscopy of highly charged ions using quantum logic
P. Micke, T. Leopold, S. A. King, E. Benkler, L. J. Spieß, L. Schmöger, M. Schwarz, J. R. Crespo López-Urrutia and P. O. Schmidt
Nature 578 (2020), DOI: 10.1038/s41586-020-1959-8
Weblinks:
Photon recoil provides new insight into matter
Frozen highly-charged ions for highest precision spectroscopy
Quantum Logic Spectroscopy group at PTB
Group “Highly charged ion dynamics” at MPIK
Abstract:
Quantum field theory is a powerful tool to describe the relevant physics governing complex quantum many-body systems. Here, we develop a general pathway to extract the irreducible building blocks of quantum field theoretical descriptions and its parameters purely from experimental data. This determination is accomplished by extracting the one-particle irreducible (1PI) correlation functions from which one can construct all physical observables. To match the capabilities of experimental techniques, our approach employs a formulation of quantum field theory based on equal-time correlation functions only. We illustrate the theoretical foundations of our procedure by applying it to the sine-Gordon model in thermal equilibrium and then demonstrate explicitly how to extract these quantities from an experiment where we quantum simulate the sine-Gordon model by two tunnel-coupled superfluids. We extract all 1PI correlation functions up to the 1PI four-point function (interaction vertex) and their variation with momentum, encoding the “running” of the couplings. The measured 1PI correlation functions are compared to the theoretical estimates, verifying our procedure. Our work opens new ways of addressing complex many-body questions emerging in a large variety of settings from fundamental science to practical quantum technology.
T.V. Zache, T. Schweigler, S. Erne, J. Schmiedmayer, J. Berges: Extracting the Field Theory Description of a Quantum Many-Body System from Experimental Data, Phys. Rev. X 10 (2020) 011020.
https://journals.aps.org/prx/abstract/10.1103/PhysRevX.10.011020
Related to Project B03, A03, A04, C01
Abstract:
Precision spectroscopy of atomic systems1 is an invaluable tool for the study of fundamental interactions and symmetries2. Recently, highly charged ions have been proposed to enable sensitive tests of physics beyond the standard model2–5 and the realization of high-accuracy atomic clocks3,5, owing to their high sensitivity to fundamental physics and insensitivity to external perturbations, which result from the high binding energies of their outer electrons. However, the implementation of these ideas has been hindered by the low spectroscopic accuracies (of the order of parts per million) achieved so far6–8. Here we cool trapped, highly charged argon ions to the lowest temperature reported so far, and study them using coherent laser spectroscopy, achieving an increase in precision of eight orders of magnitude. We use quantum logic spectroscopy9,10 to probe the forbidden optical transition in 40Ar13+ at a wavelength of 441 nanometres and measure its excited-state lifetime and g-factor. Our work unlocks the potential of highly charged ions as ubiquitous atomic systems for use in quantum information processing, as frequency standards and in highly sensitive tests of fundamental physics, such as searches for dark-matter candidates11 or violations of fundamental symmetries2.
P. Micke, T. Leopold, S.A. King, E. Benkler, L.J. Spiess, L. Schmoger, M. Schwarz, J.R. Crespo Lopez-Urrutia, P.O. Schmidt: Coherent laser spectroscopy of highly charged ions using quantum logic, Nature 578 (2020)
https://doi.org/10.1038/s41586-020-1959-8
Related to Project B01
Scientists from the Physikalisch-Technische Bundesanstalt (PTB) and the Max Planck Institute for Nuclear Physics (MPIK) have carried out pioneering optical measurements of highly charged ions with unprecedented precision. To do this, they isolated a single Ar¹³⁺ ion from an extremely hot plasma and brought it practically to rest inside an ion trap together with a laser-cooled, singly charged ion. Employing quantum logic spectroscopy on the ion pair, they have increased the relative precision by a factor of a hundred million over previous methods. This opens up the multitude of highly charged ions for novel atomic clocks and further avenues in the search for new physics. [Nature, 29.01.2020]
Highly charged ions are—although seemingly exotic—a very natural form of visible matter. All the matter in our sun and in all other stars is highly ionized, for example. In many ways, however, highly charged ions are more extreme than neutral atoms or singly charged ions. Due to their high positive charge, the outer electrons of the atomic shell are more strongly bound to the atomic nucleus. They are therefore less sensitive to perturbations by external electromagnetic fields. On the other hand, compared to neutral and singly charged atoms, the effects of special relativity and quantum electrodynamics as well as the interaction with the atomic nucleus are considerably enhanced. Highly charged ions are therefore ideal systems for accurate atomic clocks that can be used to test fundamental physics. The outer electrons in these systems serve as sensitive “quantum sensors” for effects such as previously unknown forces and fields. Since every single element of the periodic table provides as many charge states as there are electrons in the atomic shell, there exists a vast variety of atomic systems to choose from.
To date, however, established measurement techniques as used in optical atomic clocks could not be applied to highly charged ions. The main obstacle manifests already in the process of their production: a large amount of energy is required in order to remove a significant number of electrons from the atoms, and the ions then exist in the form of a plasma as hot as the Sun itself. However, the most precise and accurate experiments require the exact opposite: the lowest possible temperatures and well-controlled ambient conditions in order to reduce shifts and broadening of the spectral lines to be measured. This is hindered by the fact that highly charged ions cannot be directly laser-cooled, and conventional detection methods cannot be applied due to their atomic structure.
Physicists from the Physikalisch-Technische Bundesanstalt and the Max Planck Institute for Nuclear Physics in Heidelberg have now combined individual solutions to each of these problems in a worldwide unique experiment at the QUEST Institute for Experimental Quantum Metrology in Braunschweig. They isolated a single highly charged ion (Ar¹³⁺) from a hot plasma ion source and stored it together with a singly charged beryllium ion in an ion trap. The latter can be laser-cooled very efficiently and through the mutual electrical interaction the temperature of the entire ion pair can be reduced. Eventually, this so-called “sympathetic cooling” forms a two-ion crystal that completely “freezes” into the quantum mechanical ground state of motion at an equivalent temperature of only a few millionths of a degree above absolute zero.
Using an ultrastable laser the scientists precisely resolved the spectral structure of the Ar¹³⁺ ion in a measurement procedure similar to that used in state-of-the-art clocks. For this, they applied the concept of quantum logic, in which the spectroscopy signal is coherently transferred from the highly charged ion to the beryllium ion by means of two laser pulses. The quantum state of the beryllium ion is much easier to determine via laser excitation. “Descriptively, the beryllium ion ‘eavesdrops’ on the state of the less communicative highly charged ion and reports to us about its state”, explains Piet Schmidt, head of the collaboration. “Here, we have improved the relative precision for highly charged ions by a factor of one hundred million compared to traditional spectroscopy,” adds Peter Micke, research assistant at the QUEST Institute and first author of the paper.
Combining all these methods establishes a very general concept that can be applied to most highly charged ions. The beryllium ion can always be used as a so-called logic ion and the production process of the highly charged ions in the plasma with subsequent isolation of a single ion is independent of the choice of the atomic type and the charge state.
José Crespo, head of the group at the Max Planck Institute for Nuclear Physics, emphasizes: “This experiment opens up an unprecedented, extremely extensive area of atomic systems to be used in precision spectroscopy as well as for future clocks with special properties.” For basic research, the great variety of these new, tailored “quantum sensors” enables a promising investigation of fundamental questions: Is our standard model of particle physics complete? What is dark matter? Are fundamental constants really constant?
Original publication:
Coherent laser spectroscopy of highly charged ions using quantum logic
P. Micke, T. Leopold, S. A. King, E. Benkler, L. J. Spieß, L. Schmöger, M. Schwarz, J. R. Crespo López-Urrutia and P. O. Schmidt
Nature 578 (2020), DOI: 10.1038/s41586-020-1959-8
Weblinks:
Photon recoil provides new insight into matter
Frozen highly-charged ions for highest precision spectroscopy
Quantum Logic Spectroscopy group at PTB
Group “Highly charged ion dynamics” at MPIK
During the YRC-Meeting on Friday, 24 January 2020 new representatives were elected by the present members. The current office-holders Maximilian Prüfer and Torsten Zache were happy to hand over the responsibility of representing the young researchers to Robert Ott (PhD student A01), Eleonora Lippi (PhD student A05) and Bruno Faigle-Cedzich (PhD student C01). They were encouraging the present students and postdocs to support the new representatives and make full use of the unique opportunity to involve themselves in the organizational, managing and decision-making process of the CRC 1225. Spokesperson Prof. Dr. Berges and coordinator Tina Kuka expressed their sincere thanks and appreciation for the work done in the last 2 years. During their time as representatives, Maximilia and Torsten successfully established the Annual YRC-Retreat, were part of the organizing committee of the International Conference QSEC, invited outstanding international scientists for seminar talks and lecture series and reactivated the monthly CRC 1225 Lunch Seminars.
We are looking forward to the ideas and activities planned by the new representatives Robert, Eleonora and Bruno.
Being aware of the gender imbalance in the field of physics, the CRC 1225 wants to attract and support young female students to take up their bachelor, master or PhD thesis within the collaboration. Therefore, we plan to establish monthly lunch meetings open for female students that are interested in the topics of the CRC 1225. In the rather relaxed atmosphere of the meetings, members of the CRC present the current research work done in the different projects and invite for further discussion and exchange on any arising question.
Kick-off meeting will be on Wednesday, January 29 at 13.15hrs at the Kirchhoff-Institute for Physics, INF 227, SR 2.107 (KIP seminar box 2nd floor).
We will provide food and drinks. No prior registration is needed. We look forward to seeing many of you there! If you have any questions, do not hesitate to contact us via sfb1125@thphys.uni-heidelberg.de.
This is to announce the upcoming 2nd Annual YRC-Retreat at Kurhaus Annweiler, Trifels from April 05-08, 2020. Save the dates in your calendars – more information on programme and registration will follow soon.
Abstract:
In this paper, we propose a framework for studying the properties of the Lefschetz thimbles decomposition for lattice fermion models approaching the thermodynamic limit. The proposed set of algorithms includes the Schur complement solver and the exact computation of the derivatives of the fermion determinant. It allows us to solve the gradient flow equations taking into account the fermion determinant exactly, with high performance. Being able to do so, we can find both real and complex saddle points and describe the structure of the Lefschetz thimbles decomposition for large enough lattices which allows us to extrapolate our results to the thermodynamic limit. We describe the algorithms for a general lattice fermion model, with emphasis on two widely used types of lattice discretizations for relativistic fermions (staggered and Wilson fermions), as well as on interacting tight-binding models for condensed matter systems. As an example, we apply these algorithms to the Hubbard model on a hexagonal lattice. Several technical improvements allow us to deal with lattice volumes as large as 12×12 with Nτ=256 steps in Euclidean time, in order to capture the properties of the thimbles decomposition as the thermodynamic, low-temperature, and continuum limits are approached. Different versions of the Hubbard-Stratonovich (HS) transformation were studied, and we show that the complexity of the thimbles decomposition is very dependent on its specific form. In particular, we provide evidence for the existence of an optimal regime for the hexagonal lattice Hubbard model, with a reduced number of thimbles becoming important in the overall sum. In order to check these findings, we have performed quantum Monte Carlo (QMC) simulations using the gradient flow to deform the integration contour into the complex plane. These calculations were made on small volumes (Ns=8 sites in space), albeit still at low temperatures and with the chemical potential tuned to the van Hove singularity, thus entering into a regime where standard QMC techniques exhibit an exponential decay of the average sign. The results are compared versus exact diagonalization, and we demonstrate the importance of choosing an optimal form for the HS transformation for the Hubbard model to avoid issues associated with ergodicity. We compare the residual sign problem with the state-of-the-art BSS (Blankenbecler, Scalapino, and Sugar)-QMC and show that the average sign can be kept substantially higher using the Lefschetz thimbles approach.
M. Ulybyshev, C. Winterowd, S. Zafeiropoulos: Lefschetz thimbles decomposition for the Hubbard model on the hexagonal lattice, Phys. Rev. D 101 (2020) 014508, (DOI: 10.1103/PhysRevD.101.014508).
https://journals.aps.org/prd/abstract/10.1103/PhysRevD.101.014508
Related to Project C05
Abstract:
In this work we study the stability properties of the ground states of a spin-1 Bose gas in presence of a trapping potential in one spatial dimension. To set the stage we first map out the phase diagram for the trapped system by making use of a, so-called, continuous-time Nesterov method. We present an extension of the method, which has been previously applied to one-component systems, to our multi-component system. We show that it is a powerful and robust tool for finding the ground states of a physical system without the need of an accurate initial guess. We subsequently solve numerically the Bogoliubov de-Gennes equations in order to analyze the stability of the ground states of the trapped spin-1 system. We find that the trapping potential retains the overall structure of the stability diagram, while affecting the spectral details of each of the possible ground state waveforms. It is also found that the peak density of the trapped system is the characteristic quantity describing dynamical instabilities in the system. Therefore replacing the homogeneous density with the peak density of the trapped system leads to good agreement of the homogeneous Bogoliubov predictions with the numerically observed maximal growth rates of dynamically unstable modes. The stability conclusions in the one-dimensional trapped system are independent of the spin coupling strength and the normalized trap strength over several orders of magnitude of their variation.
C.-M. Schmied, T. Gasenzer, M. K. Oberthaler, P.G. Kevrekidis: Stability analysis of ground states in a one-dimensional trapped spin-1 Bose gas, to be published in Communications in Nonlinear Science and Numerical Simulation,Volume 83, April 2020, 105050
https://doi.org/10.1016/j.cnsns.2019.105050
Related to project A04
Abstract:
Quantum simulators allow to explore static and dynamical properties of otherwise intractable quantum many-body systems. In many instances, however, the read-out limits such quantum simulations. In this work, we introduce an innovative experimental read-out exploiting coherent non-interacting dynamics. Specifically, we present a tomographic recovery method allowing to indirectly measure the second moments of the relative density fluctuations between two one-dimensional superfluids, which until now eluded direct measurements. Applying methods from signal processing, we show that we can reconstruct the relative density fluctuations from non-equilibrium data of the relative phase fluctuations. We employ the method to investigate equilibrium states, the dynamics of phonon occupation numbers and even to predict recurrences. The method opens a new window for quantum simulations with one-dimensional superfluids, enabling a deeper analysis of their equilibration and thermalization dynamics.
M. Gluza, T. Schweigler, B. Rauer, C. Krumnow, J. Schmiedmayer, J. Eisert: Quantum read-out for cold atomic quantum simulators, Commun. Phys. 3, 12 (2020).
https://www.nature.com/articles/s42005-019-0273-y
Related to Project A03
Abstract:
We present a new approach to take into account resonance decays in the blast-wave model fits of identified hadron spectra. Thanks to precalculated decayed particle spectra, we are able to extract, in a matter of seconds, the multiplicity dependence of the single freeze-out temperature Tfo, average fluid velocity ⟨βT⟩, velocity exponent n, and the volume dV/dy of an expanding fireball. In contrast to blast-wave fits without resonance feed-down, our approach results in a freeze-out temperature of Tfo≈150MeV, which has only weak dependence on multiplicity and collision system. Finally, we discuss separate chemical and kinetic freeze-outs separated by partial chemical equilibrium.
A. Mazeliauskas, V. Vislavicius, „Temperature and fluid velocity on the freeze-out surface
from π, K, p spectra in pp, p–Pb and Pb–Pb collisions“, Phys. Rev. C 101, 014910 (2020).
https://journals.aps.org/prc/abstract/10.1103/PhysRevC.101.014910
Related to Project A01, C06
Writing in Nature, researchers describe the first-time observation of ‘self-organized criticality’ in a controlled laboratory experiment. Complex systems exist in mathematics and physics, but also occur in nature and society. The concept of self-organized criticality claims that without external input, complex systems in non-equilibrium tend to develop into a critical state far away from a stable equilibrium. That way, they reinforce their own non-equilibrium.
Systems that are at first glance quite different, like the dissemination of information in social networks or the spread of fire or disease, may have similar characteristics. One example is an avalanche-like behaviour that reinforces itself instead of coming to a standstill. However, these complex systems are very difficult to study under controlled lab conditions.
For the first time, researchers from the European Centre for Quantum Sciences (CESQ) in Strasbourg, in collaboration with researchers from the universities of Cologne and Heidelberg and the California Institute of Technology, have succeeded in observing the most important features of self-organized criticality in a controlled experiment – including universal avalanche behavior.
The team worked with a gas consisting of potassium atoms, which they prepared at very low temperatures, close to absolute zero. ‘In this state, the gas is easier to control, which makes it more suitable for studying the fundamental quantum properties of atoms,’ said Professor Shannon Whitlock at the Institute of Supramolecular Science and Technology at the University of Strasbourg.
By stimulating gas atoms with lasers, the team was able to influence the interactions between these atoms. ‘When stimulated, the atoms can either generate new secondary stimulations or discharge spontaneously’, explained Tobias Wintermantel, a doctoral researcher in Whitlock’s team.
When the laser was switched on, many atoms initially escaped very quickly. Their remaining number in the gas stabilized at the same value. Also, the number of remaining particles depended on the intensity of the laser. ‘Comparing our lab results with a theoretical model, we saw that these two effects have the same origin,’ said the theoretical physicist Professor Sebastian Diehl from the University of Cologne. This was a first indication of the phenomenon of self-organized criticality.
‘The experiments showed that some systems develop by themselves up to their critical point of phase transition’, Diehl added. This is surprising: in a typical phase transition, like boiling water turing from liquid to gas, there is only one critical point. In boiling water, self-organized criticality would mean that the system would automatically remain in a state of suspension between liquid and gas at the critical transition point – even if the temperature was changed. So far, this concept has never been verified and tested in such a highly controllable physical system.
After the experiment, the team returned to the lab to confirm another striking feature of self-organized criticality: a self-sustaining behavior of atomic decay, similar to that of continuously replenished avalanches. Similar characteristics have already been qualitatively observed in the past in other cases – such as earthquakes or solar eruptions. ‘For the first time, we observed the key elements of self-organized criticality quantitatively in the lab. We were able to establish a highly controllable atomic experimental system’, said Shannon Whitlock.
In further steps, the scientists now want to investigate how the quantum nature of atoms influences the self-organization mechanism. ‘In the long term, this might contribute to creating new quantum technologies or to solving some computation problems that are difficult for normal computers’, Diehl concluded.
The phenomenon of self-organized criticality was first developed for avalanches in 1987 by physicists Per Bak, Chao Tang and Kurt Wiesenfeld. Further models by other researchers for evolution, forest fires and earthquakes followed. So far, no general conditions that trigger self-organized criticality have been identified.
Publication:
https://www.nature.com/articles/s41586-019-1908-6
Official Press Release (French) from 16 January 2020 : http://www.recherche.unistra.fr/index.php?id=31278
English versions were published at the following places:
https://phys.org/news/2020-01-quantum-physics-self-organized-criticality.html
https://www.sciencedaily.com/releases/2020/01/200116121828.htm
https://www.azoquantum.com/News.aspx?newsID=6841
Writing in Nature, researchers describe the first-time observation of ‘self-organized criticality’ in a controlled laboratory experiment. Complex systems exist in mathematics and physics, but also occur in nature and society. The concept of self-organized criticality claims that without external input, complex systems in non-equilibrium tend to develop into a critical state far away from a stable equilibrium. That way, they reinforce their own non-equilibrium.
Systems that are at first glance quite different, like the dissemination of information in social networks or the spread of fire or disease, may have similar characteristics. One example is an avalanche-like behaviour that reinforces itself instead of coming to a standstill. However, these complex systems are very difficult to study under controlled lab conditions.
For the first time, researchers from the European Centre for Quantum Sciences (CESQ) in Strasbourg, in collaboration with researchers from the universities of Cologne and Heidelberg and the California Institute of Technology, have succeeded in observing the most important features of self-organized criticality in a controlled experiment – including universal avalanche behavior.
The team worked with a gas consisting of potassium atoms, which they prepared at very low temperatures, close to absolute zero. ‘In this state, the gas is easier to control, which makes it more suitable for studying the fundamental quantum properties of atoms,’ said Professor Shannon Whitlock at the Institute of Supramolecular Science and Technology at the University of Strasbourg.
By stimulating gas atoms with lasers, the team was able to influence the interactions between these atoms. ‘When stimulated, the atoms can either generate new secondary stimulations or discharge spontaneously’, explained Tobias Wintermantel, a doctoral researcher in Whitlock’s team.
When the laser was switched on, many atoms initially escaped very quickly. Their remaining number in the gas stabilized at the same value. Also, the number of remaining particles depended on the intensity of the laser. ‘Comparing our lab results with a theoretical model, we saw that these two effects have the same origin,’ said the theoretical physicist Professor Sebastian Diehl from the University of Cologne. This was a first indication of the phenomenon of self-organized criticality.
‘The experiments showed that some systems develop by themselves up to their critical point of phase transition’, Diehl added. This is surprising: in a typical phase transition, like boiling water turing from liquid to gas, there is only one critical point. In boiling water, self-organized criticality would mean that the system would automatically remain in a state of suspension between liquid and gas at the critical transition point – even if the temperature was changed. So far, this concept has never been verified and tested in such a highly controllable physical system.
After the experiment, the team returned to the lab to confirm another striking feature of self-organized criticality: a self-sustaining behavior of atomic decay, similar to that of continuously replenished avalanches. Similar characteristics have already been qualitatively observed in the past in other cases – such as earthquakes or solar eruptions. ‘For the first time, we observed the key elements of self-organized criticality quantitatively in the lab. We were able to establish a highly controllable atomic experimental system’, said Shannon Whitlock.
In further steps, the scientists now want to investigate how the quantum nature of atoms influences the self-organization mechanism. ‘In the long term, this might contribute to creating new quantum technologies or to solving some computation problems that are difficult for normal computers’, Diehl concluded.
The phenomenon of self-organized criticality was first developed for avalanches in 1987 by physicists Per Bak, Chao Tang and Kurt Wiesenfeld. Further models by other researchers for evolution, forest fires and earthquakes followed. So far, no general conditions that trigger self-organized criticality have been identified.
Publication:
https://www.nature.com/articles/s41586-019-1908-6
Official Press Release (French) from 16 January 2020 : http://www.recherche.unistra.fr/index.php?id=31278
English versions were published at the following places:
https://phys.org/news/2020-01-quantum-physics-self-organized-criticality.html
https://www.sciencedaily.com/releases/2020/01/200116121828.htm
https://www.azoquantum.com/News.aspx?newsID=6841
Abstract:
Self-organized criticality is an elegant explanation of how complex structures emerge and persist throughout nature, and why such structures often exhibit similar scale-invariant properties. Although self-organized criticality is sometimes captured by simple models that feature a critical point as an attractor for the dynamics, the connection to real-world systems is exceptionally hard to test quantitatively. Here we observe three key signatures of self-organized criticality in the dynamics of a driven–dissipative gas of ultracold potassium atoms: self-organization to a stationary state that is largely independent of the initial conditions; scale-invariance of the final density characterized by a unique scaling function; and large fluctuations of the number of excited atoms (avalanches) obeying a characteristic power-law distribution. This work establishes a well-controlled platform for investigating self-organization phenomena and non-equilibrium criticality, with experimental access to the underlying microscopic details of the system.
S. Helmrich, A. Arias, G. Lochead, M. Buchhold, S. Diehl, S. Whitlock: Signatures of Self-Organised Criticality in an Ultracold Atomic Gas, Nature 577 (2020).
https://www.nature.com/articles/s41586-019-1908-6
Related to Project A05
Abstract:
Few-mode models are a cornerstone of the theoretical work in quantum optics, with the famous single-mode Jaynes-Cummings model being only the most prominent example. In this work, we develop an ab initio few-mode theory, a framework connecting few-mode system-bath models to ab initio methods. We first present a method to derive exact few-mode Hamiltonians for noninteracting quantum potential scattering problems and demonstrate how to rigorously reconstruct the scattering matrix from such few-mode Hamiltonians. We show that, upon inclusion of a background scattering contribution, an ab initio version of the well-known input-output formalism is equivalent to standard scattering theory. On the basis of these exact results for noninteracting systems, we construct an effective few-mode expansion scheme for interacting theories, which allows us to extract the relevant degrees of freedom from a continuum in an open quantum system. As a whole, our results demonstrate that few-mode as well as input-output models can be extended to a general class of problems and open up the associated toolbox to be applied to various platforms and extreme regimes. We outline differences of the ab initio results to standard model assumptions, which may lead to qualitatively different effects in certain regimes. The formalism is exemplified in various simple physical scenarios. In the process, we provide a proof of concept of the method, demonstrate important properties of the expansion scheme, and exemplify new features in extreme regimes.
D. Lentrodt, J. Evers: Ab Initio Few-Mode Theory for Quantum Potential Scattering Problems, Phys. Rev. X 10 (2020) 011008
https://journals.aps.org/prx/abstract/10.1103/PhysRevX.10.011008
Related to Project B02
Abstract:
Persistent homology provides a robust methodology to infer topological structures from point cloud data. Here we explore the persistent homology of point clouds embedded into a probabilistic setting, exploiting the theory of point processes. We provide variants of notions of ergodicity and investigate measures on the space of persistence diagrams. In particular we introduce the notion of self-similar scaling of persistence diagram expectation measures and prove a packing relation for the occurring dynamical scaling exponents. As a byproduct we generalize the strong law of large numbers for persistent Betti numbers proven in [Hiraoka et al., Ann. Appl. Probab. 28(5), 2018] for averages over cubes to arbitrary convex averaging sequences.
D. Spitz, A. Wienhard, “The self-similar evolution of stationary point processes via persistent homology”, arXiv:2012.05751 (2020).
https://arxiv.org/abs/2012.05751
Related to Project B03
Abstract:
Two-loop self-energy corrections to the bound-electron g factor are investigated theoretically to all orders in the nuclear binding strength parameter Zα. The separation of divergences is performed by dimensional regularization, and the contributing diagrams are regrouped into specific categories to yield finite results. We evaluate numerically the loop-after-loop terms, and the remaining diagrams by treating the Coulomb interaction in the electron propagators up to first order. The results show that such two-loop terms are mandatory to take into account for projected near-future stringent tests of quantum electrodynamics and for the determination of fundamental constants through the g factor.
https://journals.aps.org/prresearch/abstract/10.1103/PhysRevResearch.2.012002
Related to Project B02
Abstract:
Recent theoretical works have proposed atomic clocks based on narrow optical transitions in highly charged ions. The most interesting candidates for searches of physics beyond the Standard Model are those which occur at rare orbital crossings where the shell structure of the periodic table is reordered. There are only three such crossings expected to be accessible in highly charged ions, and hitherto none have been observed as both experiment and theory have proven difficult. In this work we observe an orbital crossing in a system chosen to be tractable from both sides: Pr9+. We present electron beam ion trap measurements of its spectra, including the inter-configuration lines that reveal the sought-after crossing. With state-of-the-art calculations we show that the proposed nHz-wide clock line has a very high sensitivity to variation of the fine-structure constant, α, and violation of local Lorentz invariance; and has extremely low sensitivity to external perturbations.
H. Bekker, A. Borschevsky, Z. Harman, C. H. Keitel, T. Pfeifer, P. O. Schmidt, J. R. Crespo López-Urrutia, J. C. Berengut: Detection of the 5p−4f orbital crossing and its optical clock transition in Pr9+
Published 11 December 2019 in Nature Communications volume 10, Article number: 5651 (2019)
https://www.nature.com/articles/s41467-019-13406-9
Related to project B01
Justus Liebig University Giessen (JLU) is awarding the Röntgen Prize this year to Dr Adriana Pálffy-Buß. Since completing her doctorate, the prize winner has been conducting research in the theory division at the Max Planck Institute for Nuclear Physics in Heidelberg. She is receiving the award for her outstanding contributions in the field of theoretical atomic and nuclear physics, in particular, for the development of a new control mechanism for X-ray quanta.
Dr Adriana Pálffy-Buß was one of the first in the relatively new research field of X-ray quantum optics to demonstrate in ground-breaking theoretical work, how to control single X-ray photons by means of suitable nuclear transitions. This innovative technology opens up opportunities for the development of new ultracompact information storage.
The predictions of the physicist were recently confirmed experimentally at DESY in Hamburg. The theoretical and experimental work with the participation of Dr Adriana Pálffy-Buß has been published in numerous prestigious journals. She has also published significant works on the interaction of X-ray lasers with atomic nuclei, which promises an interesting application for storing energy.
Press Release from November 18, 2019
Justus Liebig University Giessen (JLU) is awarding the Röntgen Prize this year to Dr Adriana Pálffy-Buß. Since completing her doctorate, the prize winner has been conducting research in the theory division at the Max Planck Institute for Nuclear Physics in Heidelberg. She is receiving the award for her outstanding contributions in the field of theoretical atomic and nuclear physics, in particular, for the development of a new control mechanism for X-ray quanta.
Dr Adriana Pálffy-Buß was one of the first in the relatively new research field of X-ray quantum optics to demonstrate in ground-breaking theoretical work, how to control single X-ray photons by means of suitable nuclear transitions. This innovative technology opens up opportunities for the development of new ultracompact information storage.
The predictions of the physicist were recently confirmed experimentally at DESY in Hamburg. The theoretical and experimental work with the participation of Dr Adriana Pálffy-Buß has been published in numerous prestigious journals. She has also published significant works on the interaction of X-ray lasers with atomic nuclei, which promises an interesting application for storing energy.
Press Release from November 18, 2019
The CRC 1225 invites all interested woman for a public lecture (language: German) with coach, bestseller author and mentor Sigrid Meuselbach on the topic of “Erfolgsfaktoren und Stolpersteine auf dem Karriereweg von Frauen – Strategien für mehr Selbstbehauptung im Job”.
Wednesday, 27 November 2019
Kirchhoff-Institute for Physics, INF 227, HS 2
18:00-19:00 Public Lecture
19:00 -20:00 Reception with drinks and finger food; time for discussion and exchange with the speaker
Press Release No.90/2019, 09 August 2019
Many phenomena of the natural world evidence symmetries in their dynamic evolution which help researchers to better understand a system’s inner mechanism. In quantum physics, however, these symmetries are not always achieved. In laboratory experiments with ultracold lithium atoms, researchers from the Center for Quantum Dynamics at Heidelberg University have proven for the first time the theoretically predicted deviation from classical symmetry. Their results were published in the journal “Science”.
“In the world of classical physics, the energy of an ideal gas rises proportionally with the pressure applied. This is a direct consequence of scale symmetry, and the same relation is true in every scale invariant system. In the world of quantum mechanics, however, the interactions between the quantum particles can become so strong that this classical scale symmetry no longer applies”, explains Associate Professor Dr Tilman Enss from the Institute for Theoretical Physics. His research group collaborated with Professor Dr Selim Jochim’s group at the Institute for Physics.
In their experiments, the researchers studied the behaviour of an ultracold, superfluid gas of lithium atoms. When the gas is moved out of its equilibrium state, it starts to repeatedly expand and contract in a “breathing” motion. Unlike classical particles, these quantum particles can bind into pairs and, as a result, the superfluid becomes stiffer the more it is compressed. The group headed by primary authors Dr Puneet Murthy and Dr Nicolo Defenu – colleagues of Prof. Jochim and Dr Enss – observed this deviation from classical scale symmetry and thereby directly verified the quantum nature of this system. The researchers report that this effect gives a better insight into the behaviour of systems with similar properties such as graphene or superconductors, which have no electrical resistance when they are cooled below a certain critical temperature.
________________________________________________________________________________
Original Publication:
P. A. Murthy, N. Defenu, L. Bayha, M. Holten, P. M. Preiss, T. Enss, and S. Jochim: Quantum scale anomaly
and spatial coherence in a 2D Fermi superfluid, Science Vol. 365, pp. 268-272 (19 Jul 2019).
DOI: 10.1126/science.aau4402
________________________________________________________________________________
Dr. Nicolo Defenu
Institute for Theoretical Physics
Phone +49 6221 54-9339
defenu@thphys.uni-heidelberg.de
Heidelberg University
Communications and Marketing
Press Office, phone +49 6221 54-2311
presse@rektorat.uni-heidelberg.de
Press Release No.90/2019, 09 August 2019
Many phenomena of the natural world evidence symmetries in their dynamic evolution which help researchers to better understand a system’s inner mechanism. In quantum physics, however, these symmetries are not always achieved. In laboratory experiments with ultracold lithium atoms, researchers from the Center for Quantum Dynamics at Heidelberg University have proven for the first time the theoretically predicted deviation from classical symmetry. Their results were published in the journal “Science”.
“In the world of classical physics, the energy of an ideal gas rises proportionally with the pressure applied. This is a direct consequence of scale symmetry, and the same relation is true in every scale invariant system. In the world of quantum mechanics, however, the interactions between the quantum particles can become so strong that this classical scale symmetry no longer applies”, explains Associate Professor Dr Tilman Enss from the Institute for Theoretical Physics. His research group collaborated with Professor Dr Selim Jochim’s group at the Institute for Physics.
In their experiments, the researchers studied the behaviour of an ultracold, superfluid gas of lithium atoms. When the gas is moved out of its equilibrium state, it starts to repeatedly expand and contract in a “breathing” motion. Unlike classical particles, these quantum particles can bind into pairs and, as a result, the superfluid becomes stiffer the more it is compressed. The group headed by primary authors Dr Puneet Murthy and Dr Nicolo Defenu – colleagues of Prof. Jochim and Dr Enss – observed this deviation from classical scale symmetry and thereby directly verified the quantum nature of this system. The researchers report that this effect gives a better insight into the behaviour of systems with similar properties such as graphene or superconductors, which have no electrical resistance when they are cooled below a certain critical temperature.
________________________________________________________________________________
Original Publication:
P. A. Murthy, N. Defenu, L. Bayha, M. Holten, P. M. Preiss, T. Enss, and S. Jochim: Quantum scale anomaly
and spatial coherence in a 2D Fermi superfluid, Science Vol. 365, pp. 268-272 (19 Jul 2019).
DOI: 10.1126/science.aau4402
________________________________________________________________________________
Dr. Nicolo Defenu
Institute for Theoretical Physics
Phone +49 6221 54-9339
defenu@thphys.uni-heidelberg.de
Heidelberg University
Communications and Marketing
Press Office, phone +49 6221 54-2311
presse@rektorat.uni-heidelberg.de
Pressemitteilung Nr. 90/2019, 9. August 2019
Viele Phänomene der Natur zeigen in ihrer dynamischen Entwicklung Symmetrien, die Aufschluss über den inneren Mechanismus eines Systems geben können. Im Bereich der Quantenphysik sind diese Symmetrien jedoch nicht immer erfüllt. Wissenschaftler vom Zentrum für Quantendynamik der Universität Heidelberg konnten bei Laborexperimenten mit ultrakalten Lithiumatomen nun erstmals eine bereits in der Theorie vorhergesagte Abweichung von der klassischen Symmetrie nachweisen. Veröffentlicht wurden die Forschungsergebnisse in der Fachzeitschrift „Science“.
„In der Welt der klassischen Physik steigt die Energie eines idealen Gases proportional zum Druck, den man darauf ausübt. Das ist eine unmittelbare Folge der Skalensymmetrie, und dieselbe Relation gilt in jedem skaleninvarianten System. In der Welt der Quantenmechanik können jedoch die Wechselwirkungen zwischen Quantenteilchen so stark werden, dass diese klassische Skalensymmetrie nicht mehr gültig ist“, erläutert Privatdozent Dr. Tilman Enss vom Institut für Theoretische Physik, dessen Forschungsgruppe bei diesen Experimenten mit der Gruppe von Prof. Dr. Selim Jochim vom Physikalischen Institut der Ruperto Carola zusammenarbeitet.
Bei ihren Experimenten haben die Forscher das Verhalten eines ultrakalten, supraflüssigen Gases aus Lithiumatomen untersucht. Wenn das Gas aus seinem Gleichgewichtszustand gebracht wird, beginnt es sich in einer „atmenden“ Bewegung immer wieder auszudehnen und zu komprimieren. Anders als klassische Teilchen können sich diese Quantenteilchen zu Paaren verbinden, was dazu führt, dass sich die Supraflüssigkeit umso schwerer komprimieren lässt, je dichter sie ist. Eine solche Abweichung von der klassischen Skalensymmetrie hat nun die Gruppe um die beiden Erstautoren der Studie Dr. Puneet Murthy und Dr. Nicolo Defenu – Mitarbeiter von Prof. Jochim und Dr. Enss – beobachtet und damit die Quantennatur dieses Systems unmittelbar nachgewiesen. Dieser Effekt gibt, so die Wissenschaftler, einen besseren Einblick in das Verhalten von Systemen mit ähnlichen Eigenschaften wie Graphen oder Supraleitern, bei denen Strom ohne jeglichen Widerstand fließen kann, wenn eine sogenannte Sprungtemperatur unterschritten wird.
________________________________________________________________________________
Originalpublikation:
P. A. Murthy, N. Defenu, L. Bayha, M. Holten, P. M. Preiss, T. Enss, and S. Jochim: Quantum scale anomaly
and spatial coherence in a 2D Fermi superfluid, Science Vol. 365, pp. 268-272 (19 Jul 2019).
DOI: 10.1126/science.aau4402
________________________________________________________________________________
Dr. Nicolo Defenu
Institut für Theoretische Physik
Telefon (06221) 54-9339
defenu@thphys.uni-heidelberg.de
Universität Heidelberg
Kommunikation und Marketing
Pressestelle, Telefon (06221) 54-2311
presse@rektorat.uni-heidelberg.de
Pressemitteilung Nr. 90/2019, 9. August 2019
Viele Phänomene der Natur zeigen in ihrer dynamischen Entwicklung Symmetrien, die Aufschluss über den inneren Mechanismus eines Systems geben können. Im Bereich der Quantenphysik sind diese Symmetrien jedoch nicht immer erfüllt. Wissenschaftler vom Zentrum für Quantendynamik der Universität Heidelberg konnten bei Laborexperimenten mit ultrakalten Lithiumatomen nun erstmals eine bereits in der Theorie vorhergesagte Abweichung von der klassischen Symmetrie nachweisen. Veröffentlicht wurden die Forschungsergebnisse in der Fachzeitschrift „Science“.
„In der Welt der klassischen Physik steigt die Energie eines idealen Gases proportional zum Druck, den man darauf ausübt. Das ist eine unmittelbare Folge der Skalensymmetrie, und dieselbe Relation gilt in jedem skaleninvarianten System. In der Welt der Quantenmechanik können jedoch die Wechselwirkungen zwischen Quantenteilchen so stark werden, dass diese klassische Skalensymmetrie nicht mehr gültig ist“, erläutert Privatdozent Dr. Tilman Enss vom Institut für Theoretische Physik, dessen Forschungsgruppe bei diesen Experimenten mit der Gruppe von Prof. Dr. Selim Jochim vom Physikalischen Institut der Ruperto Carola zusammenarbeitet.
Bei ihren Experimenten haben die Forscher das Verhalten eines ultrakalten, supraflüssigen Gases aus Lithiumatomen untersucht. Wenn das Gas aus seinem Gleichgewichtszustand gebracht wird, beginnt es sich in einer „atmenden“ Bewegung immer wieder auszudehnen und zu komprimieren. Anders als klassische Teilchen können sich diese Quantenteilchen zu Paaren verbinden, was dazu führt, dass sich die Supraflüssigkeit umso schwerer komprimieren lässt, je dichter sie ist. Eine solche Abweichung von der klassischen Skalensymmetrie hat nun die Gruppe um die beiden Erstautoren der Studie Dr. Puneet Murthy und Dr. Nicolo Defenu – Mitarbeiter von Prof. Jochim und Dr. Enss – beobachtet und damit die Quantennatur dieses Systems unmittelbar nachgewiesen. Dieser Effekt gibt, so die Wissenschaftler, einen besseren Einblick in das Verhalten von Systemen mit ähnlichen Eigenschaften wie Graphen oder Supraleitern, bei denen Strom ohne jeglichen Widerstand fließen kann, wenn eine sogenannte Sprungtemperatur unterschritten wird.
________________________________________________________________________________
Originalpublikation:
P. A. Murthy, N. Defenu, L. Bayha, M. Holten, P. M. Preiss, T. Enss, and S. Jochim: Quantum scale anomaly
and spatial coherence in a 2D Fermi superfluid, Science Vol. 365, pp. 268-272 (19 Jul 2019).
DOI: 10.1126/science.aau4402
________________________________________________________________________________
Dr. Nicolo Defenu
Institut für Theoretische Physik
Telefon (06221) 54-9339
defenu@thphys.uni-heidelberg.de
Universität Heidelberg
Kommunikation und Marketing
Pressestelle, Telefon (06221) 54-2311
presse@rektorat.uni-heidelberg.de
Pressemitteilung des MPIK Heidelberg, 26. Juni 2019
Physiker des Max-Planck-Instituts für Kernphysik in Heidelberg berichten über das erste Ergebnis des neuen Alphatrap-Experiments. Sie haben den g-Faktor eines gebundenen Elektrons in hochgeladenen (borartigen) Argonionen mit einer unerreichten Genauigkeit von 9 Dezimalstellen gemessen. Im Vergleich zu einer neuen hochpräzisen quantenelektrodynamischen Berechnung fanden sie eine hervorragende Übereinstimmung bis auf 7 Dezimalstellen. Dies ebnet den Weg für empfindliche Tests der QED in starken Feldern, wie die präzise Messung der Feinstrukturkonstanten α sowie den Nachweis möglicher Signaturen neuer Physik.[Physical Review Letters, 27. Juni 2019]
Die Quantenelektrodynamik (QED) beschreibt die Wechselwirkung von geladenen Teilchen mit elektromagnetischen Feldern und ist die am besten getestete physikalische Theorie. Sie liefert äußerst exakte Vorhersagen für physikalische Messgrößen und bisher wurde in keiner Messung eine Abweichung davon gezeigt. Dennoch ist es von fundamentalem Interesse, mögliche Grenzen der Gültigkeit der QED zu finden – dies wäre ein Weg zu neuer Physik. Ein guter Zugang dafür ist ein hochpräziser Test unter extremen Bedingungen, d. h. bei extrem hohen Feldstärken.
Physiker der Abteilung von Klaus Blaum am Heidelberger Max-Planck-Institut für Kernphysik haben hierzu die magnetischen Eigenschaften von hochgeladenen Argon-Ionen in der neuen Ionenfalle ALPHATRAP mit hoher Präzision vermessen. Das untersuchte borartige Argon ist dreizehnfach geladen, und hat – wie das Element Bor – fünf Elektronen, aber eine deutlich höhere Kernladung von 18 Elementarladungen (statt 5 für Bor). Das elektrische Feld des Atomkerns, dem das äußerste Elektron ausgesetzt ist, ist dadurch im Vergleich zu Bor etwa um einen Faktor 900 erhöht.
Messgröße ist die Magnetisierung des Elektrons, die durch den so genannten g-Faktor bestimmt wird. Hierzu tragen im betrachteten Beispiel sowohl der innere Drehimpuls (Spin) als auch die Bahnbewegung des Elektrons um den Atomkern (Bahndrehimpuls) bei. Der g-Faktor als Verhältnis des magnetischen Moments des Elektrons zu seinem Gesamt-Drehimpuls ist ein Maß für die Stärke der magnetischen Wechselwirkung und kann mittels der QED sehr genau berechnet wie auch im Experiment mit vergleichbarer Genauigkeit bestimmt werden. Der Vergleich von Theorie und Experiment stellt daher einen empfindlichen Test der QED für gebundene Elektronen dar.
Für die Messung an einzelnen hochgeladenen Argon-Ionen kam erstmals die doppelte Penning-Ionenfalle Alphatrap zum Einsatz. Die Ionen werden durch ein starkes äußeres Magnetfeld auf eine Kreisbahn (Zyklotronbewegung) um die Fallenachse gezwungen. Die ringförmigen Elektroden können auf verschiedene elektrische Spannungen gelegt werden und so das Ion an einem Entweichen entlang der Fallenachse hindern wie auch entlang dieser in gewünschter Weise transportieren. Im Präzisionsfalle genannten Teil wird die Zyklotronfrequenz zerstörungsfrei mit extrem hoher Genauigkeit gemessen. Gleichzeitig werden Mikrowellen eingestrahlt, die bei geeigneter Frequenz den Spin des Elektrons samt seiner Bewegung umklappen kann – stellt man sich das Elektron als einen winzigen Kreisel vor, so stellt sich dieser dabei auf den Kopf.
Diese so-genanten „Spin-Flips“ treten in Resonanz mit der Mikrowellenfrequenz am häufigsten auf. Um solche Quantensprünge nachzuweisen, wird das Ion in die dafür entwickelte Analysfalle transportiert wo man die Ausrichtung des Spins anhand einer präzisen Frequenzmessung feststellen kann. Mit dieser Methode gelang dank der hohen Präzision und Stabilität des gesamten Aufbaus, den g-Faktor auf 9 Stellen genau zu bestimmen.
Zum Vergleich wurde in der Theorie-Abteilung von Christoph Keitel am MPIK und in einer weiteren Gruppe um Dmitry Glazov von der St. Petersburg Universität der g-Faktor für borartiges Argon neu berechnet, wobei neben QED-Beiträgen auch die Wechselwirkung mit den übrigen vier Elektronen und der Rückstoß des Atomkerns Berücksichtigung fanden. Es wurde eine Genauigkeit von sieben Stellen erreicht und der theoretische Wert stimmt auf diesem Niveau hervorragend mit dem experimentellen Resultat überein. Es handelt sich um einen der präzisesten Tests von QED-Beiträgen von Mehrelektronensystemen in starken Feldern und bereitet den Weg für zukünftige Messungen mit ALPHATRAP. Hierzu zählt auch die hochpräzise Bestimmung der von Arnold Sommerfeld eingeführten Feinstrukturkonstante α, welche als fundamentale Naturkonstante in der QED die Stärke der elektrischen und magnetischen Kräfte bestimmt.
________________________________________________________________________________
Originalpublikation:
I. Arapoglou, A. Egl, M. Höcker, T. Sailer, B. Tu, A. Weigel, R. Wolf, H. Cakir, V. A. Yerokhin, N. S. Oreshkina,
V. A. Agababaev, A. V. Volotka, D. V. Zinenko, D. A. Glazov, Z. Harman, C. H. Keitel, S. Sturm and K. Blaum:
The g-factor of Boronlike Argon 40Ar13+, Phys. Rev. Lett. 122, 253001 (2019)
doi.org/10.1103/PhysRevLett.122.253001
________________________________________________________________________________
Dr. Sven Sturm
MPI für Kernphysik
sven.sturm@mpi-hd.mpg.de
Tel.: +49 6221 516-447
Prof. Dr. Klaus Blaum
MPI für Kernphysik
klaus.blaum@mpi-hd.mpg.de
Tel.: +49 6221 516-160
Dr. Zoltán Harman
MPI für Kernphysik
harman@mpi-hd.mpg.de
Tel.: +49 6221 516-163
Hon.-Prof. Dr. Christoph Keitel
MPI für Kernphysik
keitel@mpi-hd.mpg.de
Tel.: +49 6221 516-150
Pressemitteilung des MPIK Heidelberg, 26. Juni 2019
Physiker des Max-Planck-Instituts für Kernphysik in Heidelberg berichten über das erste Ergebnis des neuen Alphatrap-Experiments. Sie haben den g-Faktor eines gebundenen Elektrons in hochgeladenen (borartigen) Argonionen mit einer unerreichten Genauigkeit von 9 Dezimalstellen gemessen. Im Vergleich zu einer neuen hochpräzisen quantenelektrodynamischen Berechnung fanden sie eine hervorragende Übereinstimmung bis auf 7 Dezimalstellen. Dies ebnet den Weg für empfindliche Tests der QED in starken Feldern, wie die präzise Messung der Feinstrukturkonstanten α sowie den Nachweis möglicher Signaturen neuer Physik.[Physical Review Letters, 27. Juni 2019]
Die Quantenelektrodynamik (QED) beschreibt die Wechselwirkung von geladenen Teilchen mit elektromagnetischen Feldern und ist die am besten getestete physikalische Theorie. Sie liefert äußerst exakte Vorhersagen für physikalische Messgrößen und bisher wurde in keiner Messung eine Abweichung davon gezeigt. Dennoch ist es von fundamentalem Interesse, mögliche Grenzen der Gültigkeit der QED zu finden – dies wäre ein Weg zu neuer Physik. Ein guter Zugang dafür ist ein hochpräziser Test unter extremen Bedingungen, d. h. bei extrem hohen Feldstärken.
Physiker der Abteilung von Klaus Blaum am Heidelberger Max-Planck-Institut für Kernphysik haben hierzu die magnetischen Eigenschaften von hochgeladenen Argon-Ionen in der neuen Ionenfalle ALPHATRAP mit hoher Präzision vermessen. Das untersuchte borartige Argon ist dreizehnfach geladen, und hat – wie das Element Bor – fünf Elektronen, aber eine deutlich höhere Kernladung von 18 Elementarladungen (statt 5 für Bor). Das elektrische Feld des Atomkerns, dem das äußerste Elektron ausgesetzt ist, ist dadurch im Vergleich zu Bor etwa um einen Faktor 900 erhöht.
Messgröße ist die Magnetisierung des Elektrons, die durch den so genannten g-Faktor bestimmt wird. Hierzu tragen im betrachteten Beispiel sowohl der innere Drehimpuls (Spin) als auch die Bahnbewegung des Elektrons um den Atomkern (Bahndrehimpuls) bei. Der g-Faktor als Verhältnis des magnetischen Moments des Elektrons zu seinem Gesamt-Drehimpuls ist ein Maß für die Stärke der magnetischen Wechselwirkung und kann mittels der QED sehr genau berechnet wie auch im Experiment mit vergleichbarer Genauigkeit bestimmt werden. Der Vergleich von Theorie und Experiment stellt daher einen empfindlichen Test der QED für gebundene Elektronen dar.
Für die Messung an einzelnen hochgeladenen Argon-Ionen kam erstmals die doppelte Penning-Ionenfalle Alphatrap zum Einsatz. Die Ionen werden durch ein starkes äußeres Magnetfeld auf eine Kreisbahn (Zyklotronbewegung) um die Fallenachse gezwungen. Die ringförmigen Elektroden können auf verschiedene elektrische Spannungen gelegt werden und so das Ion an einem Entweichen entlang der Fallenachse hindern wie auch entlang dieser in gewünschter Weise transportieren. Im Präzisionsfalle genannten Teil wird die Zyklotronfrequenz zerstörungsfrei mit extrem hoher Genauigkeit gemessen. Gleichzeitig werden Mikrowellen eingestrahlt, die bei geeigneter Frequenz den Spin des Elektrons samt seiner Bewegung umklappen kann – stellt man sich das Elektron als einen winzigen Kreisel vor, so stellt sich dieser dabei auf den Kopf.
Diese so-genanten „Spin-Flips“ treten in Resonanz mit der Mikrowellenfrequenz am häufigsten auf. Um solche Quantensprünge nachzuweisen, wird das Ion in die dafür entwickelte Analysfalle transportiert wo man die Ausrichtung des Spins anhand einer präzisen Frequenzmessung feststellen kann. Mit dieser Methode gelang dank der hohen Präzision und Stabilität des gesamten Aufbaus, den g-Faktor auf 9 Stellen genau zu bestimmen.
Zum Vergleich wurde in der Theorie-Abteilung von Christoph Keitel am MPIK und in einer weiteren Gruppe um Dmitry Glazov von der St. Petersburg Universität der g-Faktor für borartiges Argon neu berechnet, wobei neben QED-Beiträgen auch die Wechselwirkung mit den übrigen vier Elektronen und der Rückstoß des Atomkerns Berücksichtigung fanden. Es wurde eine Genauigkeit von sieben Stellen erreicht und der theoretische Wert stimmt auf diesem Niveau hervorragend mit dem experimentellen Resultat überein. Es handelt sich um einen der präzisesten Tests von QED-Beiträgen von Mehrelektronensystemen in starken Feldern und bereitet den Weg für zukünftige Messungen mit ALPHATRAP. Hierzu zählt auch die hochpräzise Bestimmung der von Arnold Sommerfeld eingeführten Feinstrukturkonstante α, welche als fundamentale Naturkonstante in der QED die Stärke der elektrischen und magnetischen Kräfte bestimmt.
________________________________________________________________________________
Originalpublikation:
I. Arapoglou, A. Egl, M. Höcker, T. Sailer, B. Tu, A. Weigel, R. Wolf, H. Cakir, V. A. Yerokhin, N. S. Oreshkina,
V. A. Agababaev, A. V. Volotka, D. V. Zinenko, D. A. Glazov, Z. Harman, C. H. Keitel, S. Sturm and K. Blaum:
The g-factor of Boronlike Argon 40Ar13+, Phys. Rev. Lett. 122, 253001 (2019)
doi.org/10.1103/PhysRevLett.122.253001
________________________________________________________________________________
Dr. Sven Sturm
MPI für Kernphysik
sven.sturm@mpi-hd.mpg.de
Tel.: +49 6221 516-447
Prof. Dr. Klaus Blaum
MPI für Kernphysik
klaus.blaum@mpi-hd.mpg.de
Tel.: +49 6221 516-160
Dr. Zoltán Harman
MPI für Kernphysik
harman@mpi-hd.mpg.de
Tel.: +49 6221 516-163
Hon.-Prof. Dr. Christoph Keitel
MPI für Kernphysik
keitel@mpi-hd.mpg.de
Tel.: +49 6221 516-150
Pressemitteilung Nr. 48/2019, 14. Mai 2019
Bei der Berechnung quantenphysikalischer Fragen in Vielteilchensystemen, zum Beispiel für die Vorhersage von Materialeigenschaften, stoßen klassische Rechner schnell an ihre Kapazitätsgrenzen. Abhilfe könnten digitale Quantensimulatoren schaffen. Bisher sind diese jedoch drastisch limitiert auf kleine Systeme mit wenigen Teilchen und lediglich kurzen Simulationszeiten. Nun konnte Dr. Philipp Hauke, Physiker an der Universität Heidelberg, gemeinsam mit Kollegen aus Dresden und Innsbruck (Österreich) zeigen, dass derartige Simulationen viel „robuster“ und damit viel stabiler sein können als bislang angenommen. Die Ergebnisse ihrer Forschungen wurden in „Science Advances“ veröffentlicht.
In der Quantenphysik bezeichnet die Vielteilchentheorie die Beschreibung einer großen Zahl von Teilchen, die miteinander in Wechselwirkung stehen. Im Zustand des thermodynamischen Gleichgewichtes lässt sich das Vielteilchensystem durch nur wenige Größen wie Temperatur oder Druck beschreiben; sie sind dabei weitgehend homogen für das gesamte System. Was passiert jedoch in der zeitlichen Folge nach einer starken Störung, etwa bei einer Materialprobe, bei der durch kurze Laserpulse abrupt Energie im Material deponiert wird? Diese sogenannte Nichtgleichgewichtsdynamik von wechselwirkenden Vielteilchensystemen exakt zu berechnen ist ein herausragendes Problem der Quantenphysik.
Berechnungen mit „klassischen“ Computern erfordern Ressourcen, die exponentiell mit der Anzahl der beteiligten Quantenteilchen ansteigen. „Das bedeutet, dass rechnerisch exakte Methoden bereits bei wenigen Dutzend Teilchen versagen. Dies liegt weit unterhalb der Anzahl, die zum Beispiel benötigt wird, um Materialeigenschaften vorherzusagen. In solchen Fällen sind Wissenschaftler auf Näherungsmethoden angewiesen, die jedoch oft unkontrolliert sind, gerade wenn es um dynamische Eigenschaften geht“, erläutert Dr. Hauke, der am Kirchhoff-Institut für Physik und am Institut für Theoretische Physik der Universität Heidelberg forscht. Ein möglicher Ausweg besteht in der digitalen Quantensimulation. Die Nichtgleichgewichtsdynamik wird dabei mit Simulatoren untersucht, die selbst quantenmechanischen Gesetzmäßigkeiten folgen.
Um die zeitliche Entwicklung in einem Quantencomputer darstellen zu können, muss diese in einzelne Operationen zerlegt werden. Dieses Vorgehen – auch Trotterisierung genannt – erzeugt jedoch unvermeidlich einen in der Simulation selbst liegenden Fehler. Dieser Trotter-Fehler kann durch ausreichend feine Zerlegungen abgeschwächt werden. Allerdings müssen extrem kleine Zerlegungsschritte gewählt werden, um eine längere zeitliche Entwicklung zuverlässig abbilden zu können. Nach dem bisherigen Stand der Forschung weitet sich der Fehler bei langen Zeitspannen und einer größeren Teilchenzahl schnell aus, was die digitale Quantensimulation in der Praxis drastisch limitiert auf kleine Systeme und kurze Zeiten.
Wie die Forscher nun mithilfe von numerischen Demonstrationen und analytischen Argumenten zeigen konnten, ist die digitale Quantensimulation jedoch viel „robuster“ und damit stabiler als bislang angenommen, solange nicht der volle Zustand eines jeden einzelnen Teilchens, sondern nur die in der Praxis relevanten Größen – wie Mittelwerte über das Gesamtsystem – in den Blick genommen werden. Für derartige Größen gibt es eine scharfe Grenze zwischen einem Bereich mit kontrollierbaren Fehlern und einer Simulation, die kein verwertbares Resultat mehr liefern kann. Unterhalb dieser Grenze hat der Trotter-Fehler nur begrenzte Auswirkungen – und zwar bei allen Zeitspannen, die bisher in der Praxis simuliert werden können, und weitgehend unabhängig von der beteiligten Teilchenzahl.
Die Forschungen haben zugleich gezeigt, dass die digitale Quantensimulation mit unverhofft großen Schritten der Zerlegung bei der Trotterisierung erstaunlich genaue Ergebnisse liefern kann. „Eine Simulation, die das Verhalten vieler Quantenteilchen über einen langen Zeitraum vorhersagen kann, wird damit immer wahrscheinlicher. Dies öffnet die Tür ein beträchtliches Stück weiter für praktische Anwendungen, die von der Materialwissenschaft über Quantenchemie hin zu Fragestellungen der fundamentalen Physik reichen“, betont Dr. Hauke, der die Forschungsgruppe „Quantenoptik und Quantenvielteilchentheorie“ leitet.
Die Forschungsarbeiten wurden gemeinsam mit Dr. Markus Heyl vom Max-Planck-Institut für Physik komplexer Systeme in Dresden und Prof. Dr. Peter Zoller von der Universität Innsbruck durchgeführt. An der Universität Heidelberg fanden sie im Rahmen des Sonderforschungsbereichs „Isolierte Quantensysteme und Universalität unter extremen Bedingungen“ (SFB 1225) statt.
________________________________________________________________________________
Originalpublikation:
M. Heyl, P. Hauke, P. Zoller: Quantum localization bounds Trotter errors in digital quantum simulation, Science
Advances (2019; 5:eaau834)
DOI: 10.1126/sciadv.aau8342
________________________________________________________________________________
Dr. Philipp Hauke
Kirchhoff-Institut für Physik
Tel. +49 6221 54-5185
philipp.hauke@kip.uni-heidelberg.de
Kommunikation und Marketing
Pressestelle
Tel. +49 6221 54-2311
presse@rektorat.uni-heidelberg.de
Pressemitteilung Nr. 48/2019, 14. Mai 2019
Bei der Berechnung quantenphysikalischer Fragen in Vielteilchensystemen, zum Beispiel für die Vorhersage von Materialeigenschaften, stoßen klassische Rechner schnell an ihre Kapazitätsgrenzen. Abhilfe könnten digitale Quantensimulatoren schaffen. Bisher sind diese jedoch drastisch limitiert auf kleine Systeme mit wenigen Teilchen und lediglich kurzen Simulationszeiten. Nun konnte Dr. Philipp Hauke, Physiker an der Universität Heidelberg, gemeinsam mit Kollegen aus Dresden und Innsbruck (Österreich) zeigen, dass derartige Simulationen viel „robuster“ und damit viel stabiler sein können als bislang angenommen. Die Ergebnisse ihrer Forschungen wurden in „Science Advances“ veröffentlicht.
In der Quantenphysik bezeichnet die Vielteilchentheorie die Beschreibung einer großen Zahl von Teilchen, die miteinander in Wechselwirkung stehen. Im Zustand des thermodynamischen Gleichgewichtes lässt sich das Vielteilchensystem durch nur wenige Größen wie Temperatur oder Druck beschreiben; sie sind dabei weitgehend homogen für das gesamte System. Was passiert jedoch in der zeitlichen Folge nach einer starken Störung, etwa bei einer Materialprobe, bei der durch kurze Laserpulse abrupt Energie im Material deponiert wird? Diese sogenannte Nichtgleichgewichtsdynamik von wechselwirkenden Vielteilchensystemen exakt zu berechnen ist ein herausragendes Problem der Quantenphysik.
Berechnungen mit „klassischen“ Computern erfordern Ressourcen, die exponentiell mit der Anzahl der beteiligten Quantenteilchen ansteigen. „Das bedeutet, dass rechnerisch exakte Methoden bereits bei wenigen Dutzend Teilchen versagen. Dies liegt weit unterhalb der Anzahl, die zum Beispiel benötigt wird, um Materialeigenschaften vorherzusagen. In solchen Fällen sind Wissenschaftler auf Näherungsmethoden angewiesen, die jedoch oft unkontrolliert sind, gerade wenn es um dynamische Eigenschaften geht“, erläutert Dr. Hauke, der am Kirchhoff-Institut für Physik und am Institut für Theoretische Physik der Universität Heidelberg forscht. Ein möglicher Ausweg besteht in der digitalen Quantensimulation. Die Nichtgleichgewichtsdynamik wird dabei mit Simulatoren untersucht, die selbst quantenmechanischen Gesetzmäßigkeiten folgen.
Um die zeitliche Entwicklung in einem Quantencomputer darstellen zu können, muss diese in einzelne Operationen zerlegt werden. Dieses Vorgehen – auch Trotterisierung genannt – erzeugt jedoch unvermeidlich einen in der Simulation selbst liegenden Fehler. Dieser Trotter-Fehler kann durch ausreichend feine Zerlegungen abgeschwächt werden. Allerdings müssen extrem kleine Zerlegungsschritte gewählt werden, um eine längere zeitliche Entwicklung zuverlässig abbilden zu können. Nach dem bisherigen Stand der Forschung weitet sich der Fehler bei langen Zeitspannen und einer größeren Teilchenzahl schnell aus, was die digitale Quantensimulation in der Praxis drastisch limitiert auf kleine Systeme und kurze Zeiten.
Wie die Forscher nun mithilfe von numerischen Demonstrationen und analytischen Argumenten zeigen konnten, ist die digitale Quantensimulation jedoch viel „robuster“ und damit stabiler als bislang angenommen, solange nicht der volle Zustand eines jeden einzelnen Teilchens, sondern nur die in der Praxis relevanten Größen – wie Mittelwerte über das Gesamtsystem – in den Blick genommen werden. Für derartige Größen gibt es eine scharfe Grenze zwischen einem Bereich mit kontrollierbaren Fehlern und einer Simulation, die kein verwertbares Resultat mehr liefern kann. Unterhalb dieser Grenze hat der Trotter-Fehler nur begrenzte Auswirkungen – und zwar bei allen Zeitspannen, die bisher in der Praxis simuliert werden können, und weitgehend unabhängig von der beteiligten Teilchenzahl.
Die Forschungen haben zugleich gezeigt, dass die digitale Quantensimulation mit unverhofft großen Schritten der Zerlegung bei der Trotterisierung erstaunlich genaue Ergebnisse liefern kann. „Eine Simulation, die das Verhalten vieler Quantenteilchen über einen langen Zeitraum vorhersagen kann, wird damit immer wahrscheinlicher. Dies öffnet die Tür ein beträchtliches Stück weiter für praktische Anwendungen, die von der Materialwissenschaft über Quantenchemie hin zu Fragestellungen der fundamentalen Physik reichen“, betont Dr. Hauke, der die Forschungsgruppe „Quantenoptik und Quantenvielteilchentheorie“ leitet.
Die Forschungsarbeiten wurden gemeinsam mit Dr. Markus Heyl vom Max-Planck-Institut für Physik komplexer Systeme in Dresden und Prof. Dr. Peter Zoller von der Universität Innsbruck durchgeführt. An der Universität Heidelberg fanden sie im Rahmen des Sonderforschungsbereichs „Isolierte Quantensysteme und Universalität unter extremen Bedingungen“ (SFB 1225) statt.
________________________________________________________________________________
Originalpublikation:
M. Heyl, P. Hauke, P. Zoller: Quantum localization bounds Trotter errors in digital quantum simulation, Science
Advances (2019; 5:eaau834)
DOI: 10.1126/sciadv.aau8342
________________________________________________________________________________
Dr. Philipp Hauke
Kirchhoff-Institut für Physik
Tel. +49 6221 54-5185
philipp.hauke@kip.uni-heidelberg.de
Kommunikation und Marketing
Pressestelle
Tel. +49 6221 54-2311
presse@rektorat.uni-heidelberg.de
Abstract:
Quantum annealing is a computing paradigm that has the ambitious goal of efficiently solving large-scale combinatorial optimization problems of practical importance. However, many challenges have yet to be overcome before this goal can be reached. This perspectives article first gives a brief introduction to the concept of quantum annealing, and then highlights new pathways that may clear the way towards feasible and large scale quantum annealing. Moreover, since this field of research is to a strong degree driven by a synergy between experiment and theory, we discuss both in this work. An important focus in this article is on future perspectives, which complements other review articles, and which we hope will motivate further research.
P. Hauke, H. G. Katzgraber, W. Lechner, H. Nishimori, and W. D. Oliver, “Perspectives of quantum annealing: Methods and implementations”, Rep. Prog. Phys. 83, 054401 (2020).
https://iopscience.iop.org/article/10.1088/1361-6633/ab85b8
Related to Project: B04
Pressemitteilung GSI vom 15.04.2019
Professor Peter Braun-Munzinger, wissenschaftlicher Direktor des ExtreMe Matter Instituts EMMI am GSI Helmholtzzentrum für Schwerionenforschung, hat die renommierte Stern-Gerlach-Medaille der Deutschen Physikalischen Gesellschaft (DPG) erhalten. Gemeinsam mit Peter Braun-Munzinger ist auch Professorin Johanna Stachel von der Universität Heidelberg ausgezeichnet worden. Die beiden Forscher erhalten den Preis für ihre herausragenden Beiträge zum Bau und Betrieb zentraler Detektoren des ALICE Experiments am CERN Large Hadron Collider sowie zur Interpretation von Schwerionenkollisionen und zum Verständnis der Phasenstruktur von Materie unter extremen Bedingungen. Verliehen wurde die Auszeichnung bei der DPG-Jahrestagung in Rostock.
Der 72 Jahre alte Kernphysiker Peter Braun-Munzinger, der sich vor allem mit ultrarelativistischen Schwerionenstößen und dem dabei erzeugten Quark-Gluon-Plasma befasst, leitete von 1996 bis 2011 die ALICE-Abteilung bei GSI und war in dieser Zeit auch als Professor an der TU Darmstadt tätig. GSI hat von Beginn an eine führende Rolle bei Bau und wissenschaftlichem Programm von ALICE gespielt, einem der größten Experimente am europäischen Kernforschungszentrum CERN. Hauptziel von ALICE ist es, einen Materiezustand, der bis Sekundenbruchteile nach dem Urknall existierte, das „Quark-Gluon Plasma“, zu erforschen.
Professor Peter Braun-Munzinger studierte Physik an der Universität Heidelberg, wo er mit summa cum laude promovierte. Als Doktorand war er Stipendiat der Studienstiftung des Deutschen Volkes, danach folgte die Zeit als Post-Doktorand am Max-Planck-Institut für Kernphysik in Heidelberg. Ab 1976 war Braun-Munzinger an der State University of New York at Stony Brook, 1982 wurde er dort Full Professor. Nach seiner Rückkehr nach Deutschland war er 1998 bis 2010 Projektleiter der „Time Projection Chamber“ von ALICE am CERN, von 2011 bis 2016 Vorsitzender des Collaboration Boards von ALICE und von 2011 bis 2014 Helmholtz-Professor bei GSI. Seit Oktober 2014 ist er Honorarprofessor an der Universität Heidelberg.
Von 1984 bis 1987 und erneut von 2000 bis 2002 war Braun-Munzinger außerdem Mitherausgeber von Physical Review Letters, einer der ältesten und angesehensten Fachzeitschriften in der Physik, die von der American Physical Society herausgegeben wird. Das wissenschaftliche Werk von Peter Braun-Munzinger wurde mit zahlreichen Auszeichnungen gewürdigt: Unter anderem wurde er 1994 Fellow der American Physical Society, 2011 Mitglied der Academia Europaea. Im Jahr 2014 erhielt er den Lise-Meitner-Preis. Nun kam die Verleihung der Stern-Gerlach-Medaille hinzu.
Auch die 64 Jahre alte Professorin Johanna Stachel, die jetzt als erste Frau die Stern-Gerlach-Medaille erhielt, ist über ALICE mit GSI verbunden. Die Kern- und Teilchenphysikerin, die an der Johannes Gutenberg-Universität Mainz Chemie und Physik studiert und mit summa cum laude promoviert hat, konzentriert sich bei ihrer Forschung auf das Verständnis der Kollisionen von Atomkernen mit ultra-relativistischen Energien. Johanna Stachel lehrt an der Ruprecht-Karls-Universität Heidelberg. Im Forschungszentrum CERN in Genf erforscht sie in Experimenten mit dem Teilchenbeschleuniger Large Hadron Collider das „Quark-Gluon-Plasma“und leitet das „Transition Radiation Detector“-Projekt bei ALICE; zudem ist sie Sprecherin des BMBF-Forschungsschwerpunkts ALICE. Von 2012 bis 2014 war sie Präsidentin der Deutschen Physikalischen Gesellschaft. Auch Johanna Stachels Wirken wurde bereits mit zahlreichen Auszeichnungen gewürdigt, unter anderem ist sie Mitglied der Nationalen Akademie der Wissenschaften Leopoldina und hat das Bundesverdienstkreuz am Bande sowie den Lise-Meitner-Preis erhalten.
Professor Paolo Giubellino, Wissenschaftlicher Geschäftsführer von GSI und FAIR und bis 2016 Sprecher des ALICE-Experiments, zeigte sich sehr erfreut über die aktuelle Auszeichnung für Braun-Munzinger und Stachel: „Sie haben beide außerordentliche Beiträge zur Physik der Schwerionen-Kollisionen geleistet. Ich bin sehr froh, dass Professor Peter Braun-Munzinger als wissenschaftlicher Direktor des ExtreMe Matter Instituts EMMI seine große Kompetenz bei GSI einbringt und Grundlegendes dazu beiträgt, neue Aspekte extremer Materie zu entdecken und zu verstehen. Auch für das wissenschaftliche Programm des künftigen Beschleunigerzentrums FAIR haben die wissenschaftlichen Arbeiten herausragende Bedeutung.“
Die Stern-Gerlach-Medaille ist die höchste Auszeichnung der DPG für herausragende Leistungen auf dem Gebiet der experimentellen Physik. Sie wird jährlich verliehen. Der Preis besteht aus einer handgeschriebenen Urkunde aus Pergament und einer goldenen Medaille mit den Porträts der beiden Physiker Otto Stern und Walther Gerlach, nach denen auch der Stern-Gerlach-Versuch benannt ist, ein grundlegendes Experiment in der Physik. (BP)
________________________________________________________________________________
Videos:
Stern-Gerlach-Medaille 2019 – Interview: Prof. Dr. Johanna Stachel & Prof. Dr. Peter Braun-Munzinger
Stern-Gerlach-Medaille 2019 – Preisträgervortrag von Prof. Dr. Johanna Stachel
Stern-Gerlach-Medaille 2019 – Preisträgervortrag von Prof. Dr. Peter Braun-Munzinger
________________________________________________________________________________
Kontakt zur Presse- und Öffentlichkeitsarbeit
Dr. Ingo Peter, Pressesprecher
Fon: +49-6159-71-2598
Katharina Becker, Teamassistenz
Fon: +49-6159-71-1397
Pressemitteilung GSI vom 15.04.2019
Professor Peter Braun-Munzinger, wissenschaftlicher Direktor des ExtreMe Matter Instituts EMMI am GSI Helmholtzzentrum für Schwerionenforschung, hat die renommierte Stern-Gerlach-Medaille der Deutschen Physikalischen Gesellschaft (DPG) erhalten. Gemeinsam mit Peter Braun-Munzinger ist auch Professorin Johanna Stachel von der Universität Heidelberg ausgezeichnet worden. Die beiden Forscher erhalten den Preis für ihre herausragenden Beiträge zum Bau und Betrieb zentraler Detektoren des ALICE Experiments am CERN Large Hadron Collider sowie zur Interpretation von Schwerionenkollisionen und zum Verständnis der Phasenstruktur von Materie unter extremen Bedingungen. Verliehen wurde die Auszeichnung bei der DPG-Jahrestagung in Rostock.
Der 72 Jahre alte Kernphysiker Peter Braun-Munzinger, der sich vor allem mit ultrarelativistischen Schwerionenstößen und dem dabei erzeugten Quark-Gluon-Plasma befasst, leitete von 1996 bis 2011 die ALICE-Abteilung bei GSI und war in dieser Zeit auch als Professor an der TU Darmstadt tätig. GSI hat von Beginn an eine führende Rolle bei Bau und wissenschaftlichem Programm von ALICE gespielt, einem der größten Experimente am europäischen Kernforschungszentrum CERN. Hauptziel von ALICE ist es, einen Materiezustand, der bis Sekundenbruchteile nach dem Urknall existierte, das „Quark-Gluon Plasma“, zu erforschen.
Professor Peter Braun-Munzinger studierte Physik an der Universität Heidelberg, wo er mit summa cum laude promovierte. Als Doktorand war er Stipendiat der Studienstiftung des Deutschen Volkes, danach folgte die Zeit als Post-Doktorand am Max-Planck-Institut für Kernphysik in Heidelberg. Ab 1976 war Braun-Munzinger an der State University of New York at Stony Brook, 1982 wurde er dort Full Professor. Nach seiner Rückkehr nach Deutschland war er 1998 bis 2010 Projektleiter der „Time Projection Chamber“ von ALICE am CERN, von 2011 bis 2016 Vorsitzender des Collaboration Boards von ALICE und von 2011 bis 2014 Helmholtz-Professor bei GSI. Seit Oktober 2014 ist er Honorarprofessor an der Universität Heidelberg.
Von 1984 bis 1987 und erneut von 2000 bis 2002 war Braun-Munzinger außerdem Mitherausgeber von Physical Review Letters, einer der ältesten und angesehensten Fachzeitschriften in der Physik, die von der American Physical Society herausgegeben wird. Das wissenschaftliche Werk von Peter Braun-Munzinger wurde mit zahlreichen Auszeichnungen gewürdigt: Unter anderem wurde er 1994 Fellow der American Physical Society, 2011 Mitglied der Academia Europaea. Im Jahr 2014 erhielt er den Lise-Meitner-Preis. Nun kam die Verleihung der Stern-Gerlach-Medaille hinzu.
Auch die 64 Jahre alte Professorin Johanna Stachel, die jetzt als erste Frau die Stern-Gerlach-Medaille erhielt, ist über ALICE mit GSI verbunden. Die Kern- und Teilchenphysikerin, die an der Johannes Gutenberg-Universität Mainz Chemie und Physik studiert und mit summa cum laude promoviert hat, konzentriert sich bei ihrer Forschung auf das Verständnis der Kollisionen von Atomkernen mit ultra-relativistischen Energien. Johanna Stachel lehrt an der Ruprecht-Karls-Universität Heidelberg. Im Forschungszentrum CERN in Genf erforscht sie in Experimenten mit dem Teilchenbeschleuniger Large Hadron Collider das „Quark-Gluon-Plasma“und leitet das „Transition Radiation Detector“-Projekt bei ALICE; zudem ist sie Sprecherin des BMBF-Forschungsschwerpunkts ALICE. Von 2012 bis 2014 war sie Präsidentin der Deutschen Physikalischen Gesellschaft. Auch Johanna Stachels Wirken wurde bereits mit zahlreichen Auszeichnungen gewürdigt, unter anderem ist sie Mitglied der Nationalen Akademie der Wissenschaften Leopoldina und hat das Bundesverdienstkreuz am Bande sowie den Lise-Meitner-Preis erhalten.
Professor Paolo Giubellino, Wissenschaftlicher Geschäftsführer von GSI und FAIR und bis 2016 Sprecher des ALICE-Experiments, zeigte sich sehr erfreut über die aktuelle Auszeichnung für Braun-Munzinger und Stachel: „Sie haben beide außerordentliche Beiträge zur Physik der Schwerionen-Kollisionen geleistet. Ich bin sehr froh, dass Professor Peter Braun-Munzinger als wissenschaftlicher Direktor des ExtreMe Matter Instituts EMMI seine große Kompetenz bei GSI einbringt und Grundlegendes dazu beiträgt, neue Aspekte extremer Materie zu entdecken und zu verstehen. Auch für das wissenschaftliche Programm des künftigen Beschleunigerzentrums FAIR haben die wissenschaftlichen Arbeiten herausragende Bedeutung.“
Die Stern-Gerlach-Medaille ist die höchste Auszeichnung der DPG für herausragende Leistungen auf dem Gebiet der experimentellen Physik. Sie wird jährlich verliehen. Der Preis besteht aus einer handgeschriebenen Urkunde aus Pergament und einer goldenen Medaille mit den Porträts der beiden Physiker Otto Stern und Walther Gerlach, nach denen auch der Stern-Gerlach-Versuch benannt ist, ein grundlegendes Experiment in der Physik. (BP)
________________________________________________________________________________
Videos:
Stern-Gerlach-Medaille 2019 – Interview: Prof. Dr. Johanna Stachel & Prof. Dr. Peter Braun-Munzinger
Stern-Gerlach-Medaille 2019 – Preisträgervortrag von Prof. Dr. Johanna Stachel
Stern-Gerlach-Medaille 2019 – Preisträgervortrag von Prof. Dr. Peter Braun-Munzinger
________________________________________________________________________________
Kontakt zur Presse- und Öffentlichkeitsarbeit
Dr. Ingo Peter, Pressesprecher
Fon: +49-6159-71-2598
Katharina Becker, Teamassistenz
Fon: +49-6159-71-1397
Pressemitteilung des MPIK Heidelberg, 01. April 2019
In der jüngsten Ausschreibungsrunde für Advanced Grants des Europäischen Forschungsrats (ERC) war Prof. Dr. Klaus Blaum vom MPI für Kernphysik mit seinem Projekt „FunI“ bereits zum zweiten Mal erfolgreich. Das Projekt wird über 5 Jahre mit insgesamt 2,5 Millionen Euro gefördert.
Die vier fundamentalen Wechselwirkungen und ihre Symmetrien, die Fundamentalkonstanten sowie die Eigenschaften von Elementarteilchen wie ihre Massen bestimmen die grundlegende Struktur des Universums und bilden die Grundlage für das so exzellent bewährte Standardmodell der Physik. Nachdem Klaus Blaum bereits 2011 einen ERC Advanced Grant für die Bestimmung von Fundamentkonstanten wie die Masse des Elektrons und des Protons erhielt, widmet er sich nun in seinem neuen ERC Advanced Grant mit dem Namen „FunI“ den fundamentalen Wechselwirkungen. Dabei sind u.a. Experimente mit gespeicherten hochgeladenen Ionen für zwingende Tests der Quantenelektrodynamik und der speziellen Relativitätstheorie geplant, letztere über eine verbesserte Überprüfung der Äquivalenz von Masse und Energie, E=mc2. Um die aktuellen Grenzen deutlich zu verbessern, sind im Rahmen von FunI auch technische Innovationen angestrebt, wie das sympathetische Laserkühlen einzelner Ionen.
Klaus Blaum studierte Physik an der Johannes Gutenberg-Universität Mainz. Nach der Promotion in Mainz wechselte er als Postdoktorand der GSI Helmholtzzentrum für Schwerionenforschung Darmstadt an das Europäische Kernforschungszentrum CERN in Genf. 2004 bis 2007 leitete er eine Helmholtz-Forschungsgruppe in Mainz (Habilitation 2006), von wo ihn seine Karriere nach Heidelberg an das MPI für Kernphysik führte. Dorthin wurde er 2007 mit nur 35 Jahren als Direktor der Abteilung „Gespeicherte und gekühlte Ionen“ berufen. Zugleich ist er Professor und Mitglied der Fakultät für Physik und Astronomie der Ruprecht-Karls-Universität Heidelberg. Für seine bahnbrechenden Arbeiten erhielt er eine Reihe von Preisen.
Der Europäische Forschungsrat vergibt Advanced Grants an etablierte Wissenschaftler*innen, die bereits bedeutende Beiträge zu ihrem Fachgebiet geleistet haben, für bahnbrechend neue und riskante Projekte. Die Forschung muss in einer Forschungsinstitution stattfinden, die in einem Mitgliedsstaat der Europäischen Union oder einem assoziierten Staat angesiedelt ist. Aus der jüngsten Ausschreibungsrunde werden – bei einer Erfolgsquote von 10,8% – 222 Antragsteller*innen gefördert.
________________________________________________________________________________
EU invests €540 million to boost cutting-edge research – Pressemeldung des ERC
Acht Max-Planck-Forscher werben hohe EU-Förderung ein – Pressemeldung der MPG
________________________________________________________________________________
Kontakt
Prof. Dr. Klaus Blaum
MPIK Heidelberg
Tel.: 06221 516850
Pressemitteilung des MPIK Heidelberg, 01. April 2019
In der jüngsten Ausschreibungsrunde für Advanced Grants des Europäischen Forschungsrats (ERC) war Prof. Dr. Klaus Blaum vom MPI für Kernphysik mit seinem Projekt „FunI“ bereits zum zweiten Mal erfolgreich. Das Projekt wird über 5 Jahre mit insgesamt 2,5 Millionen Euro gefördert.
Die vier fundamentalen Wechselwirkungen und ihre Symmetrien, die Fundamentalkonstanten sowie die Eigenschaften von Elementarteilchen wie ihre Massen bestimmen die grundlegende Struktur des Universums und bilden die Grundlage für das so exzellent bewährte Standardmodell der Physik. Nachdem Klaus Blaum bereits 2011 einen ERC Advanced Grant für die Bestimmung von Fundamentkonstanten wie die Masse des Elektrons und des Protons erhielt, widmet er sich nun in seinem neuen ERC Advanced Grant mit dem Namen „FunI“ den fundamentalen Wechselwirkungen. Dabei sind u.a. Experimente mit gespeicherten hochgeladenen Ionen für zwingende Tests der Quantenelektrodynamik und der speziellen Relativitätstheorie geplant, letztere über eine verbesserte Überprüfung der Äquivalenz von Masse und Energie, E=mc2. Um die aktuellen Grenzen deutlich zu verbessern, sind im Rahmen von FunI auch technische Innovationen angestrebt, wie das sympathetische Laserkühlen einzelner Ionen.
Klaus Blaum studierte Physik an der Johannes Gutenberg-Universität Mainz. Nach der Promotion in Mainz wechselte er als Postdoktorand der GSI Helmholtzzentrum für Schwerionenforschung Darmstadt an das Europäische Kernforschungszentrum CERN in Genf. 2004 bis 2007 leitete er eine Helmholtz-Forschungsgruppe in Mainz (Habilitation 2006), von wo ihn seine Karriere nach Heidelberg an das MPI für Kernphysik führte. Dorthin wurde er 2007 mit nur 35 Jahren als Direktor der Abteilung „Gespeicherte und gekühlte Ionen“ berufen. Zugleich ist er Professor und Mitglied der Fakultät für Physik und Astronomie der Ruprecht-Karls-Universität Heidelberg. Für seine bahnbrechenden Arbeiten erhielt er eine Reihe von Preisen.
Der Europäische Forschungsrat vergibt Advanced Grants an etablierte Wissenschaftler*innen, die bereits bedeutende Beiträge zu ihrem Fachgebiet geleistet haben, für bahnbrechend neue und riskante Projekte. Die Forschung muss in einer Forschungsinstitution stattfinden, die in einem Mitgliedsstaat der Europäischen Union oder einem assoziierten Staat angesiedelt ist. Aus der jüngsten Ausschreibungsrunde werden – bei einer Erfolgsquote von 10,8% – 222 Antragsteller*innen gefördert.
________________________________________________________________________________
EU invests €540 million to boost cutting-edge research – Pressemeldung des ERC
Acht Max-Planck-Forscher werben hohe EU-Förderung ein – Pressemeldung der MPG
________________________________________________________________________________
Kontakt
Prof. Dr. Klaus Blaum
MPIK Heidelberg
Tel.: 06221 516850
Pressemitteilung des MPIK Heidelberg, 24. Januar 2019
Die Königlich Schwedische Akademie der Wissenschaften hat Prof. Dr. Klaus Blaum zum Auswärtigen Mitglied ihrer Physikklasse gewählt. Die Entscheidung fiel in der Generalversammlung am 16.01.2019. Bei einer der kommenden Sitzungen soll Klaus Blaum im Rahmen einer kleinen Zeremonie in der Akademie begrüßt werden und eine Urkunde erhalten.
Die 1739 gegründete Königlich Schwedische Akademie der Wissenschaften ist eine unabhängige Nichtregierungs-Organisation, deren Aufgabe es ist, die Wissenschaften, insbesondere Naturwissenschaften und Mathematik, zu fördern und ihren Einfluss in der Gesellschaft zu stärken. Sie gliedert sich in 10 Klassen (Fachgruppen), gibt mehrere Zeitungen und Zeitschriften heraus und betreibt auch eigene Forschungsinstitute. Die Akademie hat aktuell ca. 460 schwedische und 175 auswärtige Mitglieder.
Es ist eine herausragende Anerkennung erfolgreicher Forschung, als Mitglied der Königlich Schwedischen Akademie gewählt zu werden. Außerdem ist die Akademie mit ihren Nobel-Komitees für die Vergabe der Nobelpreise in Physik und Chemie sowie den Alfred-Nobel-Gedächtnispreis der Schwedischen Reichsbank für Wirtschaftswissenschaften verantwortlich und vergibt eine Reihe von weiteren Preisen.
Klaus Blaum studierte Physik an der Johannes Gutenberg-Universität Mainz. Nach der Promotion in Mainz wechselte er als Postdoktorand der GSI Helmholtzzentrum für Schwerionenforschung Darmstadt an das Europäische Kernforschungszentrum CERN in Genf. 2004 bis 2007 leitete er eine Helmholtz-Forschungsgruppe in Mainz (Habilitation 2006), von wo ihn seine Karriere nach Heidelberg an das MPI für Kernphysik führte. Dorthin wurde er 2007 mit nur 35 Jahren als Direktor der Abteilung „Gespeicherte und gekühlte Ionen“ berufen. Zugleich ist er Professor und Mitglied der Fakultät für Physik und Astronomie der Ruprecht-Karls-Universität Heidelberg. Für seine bahnbrechenden Arbeiten erhielt er eine Reihe von Preisen, darunter den Gustav-Hertz-Preis 2004 der Deutschen Physikalischen Gesellschaft, den Mattauch-Herzog-Preis 2005 der Deutschen Gesellschaft für Massenspektrometrie, 2012 (zusammen mit seinen Doktoranden Anke Wagner und Sven Sturm) den Helmholtzpreis für Präzisionsmessung, 2013 den G.N. Flerov-Preis und 2016 den Lise Meitner Award des Physik-Zentrums Göteborg. Außerdem wurde er 2008 zum Fellow der American Physical Society ernannt und gewann 2011 einen Advanced Grant des Europäischen Forschungsrats.
________________________________________________________________________________
Mitteilung der Königlich Schwedischen Akademie der Wissenschaften
________________________________________________________________________________
Kontakt
Prof. Dr. Klaus Blaum
MPIK Heidelberg
Tel.: 06221 516850
Pressemitteilung des MPIK Heidelberg, 24. Januar 2019
Die Königlich Schwedische Akademie der Wissenschaften hat Prof. Dr. Klaus Blaum zum Auswärtigen Mitglied ihrer Physikklasse gewählt. Die Entscheidung fiel in der Generalversammlung am 16.01.2019. Bei einer der kommenden Sitzungen soll Klaus Blaum im Rahmen einer kleinen Zeremonie in der Akademie begrüßt werden und eine Urkunde erhalten.
Die 1739 gegründete Königlich Schwedische Akademie der Wissenschaften ist eine unabhängige Nichtregierungs-Organisation, deren Aufgabe es ist, die Wissenschaften, insbesondere Naturwissenschaften und Mathematik, zu fördern und ihren Einfluss in der Gesellschaft zu stärken. Sie gliedert sich in 10 Klassen (Fachgruppen), gibt mehrere Zeitungen und Zeitschriften heraus und betreibt auch eigene Forschungsinstitute. Die Akademie hat aktuell ca. 460 schwedische und 175 auswärtige Mitglieder.
Es ist eine herausragende Anerkennung erfolgreicher Forschung, als Mitglied der Königlich Schwedischen Akademie gewählt zu werden. Außerdem ist die Akademie mit ihren Nobel-Komitees für die Vergabe der Nobelpreise in Physik und Chemie sowie den Alfred-Nobel-Gedächtnispreis der Schwedischen Reichsbank für Wirtschaftswissenschaften verantwortlich und vergibt eine Reihe von weiteren Preisen.
Klaus Blaum studierte Physik an der Johannes Gutenberg-Universität Mainz. Nach der Promotion in Mainz wechselte er als Postdoktorand der GSI Helmholtzzentrum für Schwerionenforschung Darmstadt an das Europäische Kernforschungszentrum CERN in Genf. 2004 bis 2007 leitete er eine Helmholtz-Forschungsgruppe in Mainz (Habilitation 2006), von wo ihn seine Karriere nach Heidelberg an das MPI für Kernphysik führte. Dorthin wurde er 2007 mit nur 35 Jahren als Direktor der Abteilung „Gespeicherte und gekühlte Ionen“ berufen. Zugleich ist er Professor und Mitglied der Fakultät für Physik und Astronomie der Ruprecht-Karls-Universität Heidelberg. Für seine bahnbrechenden Arbeiten erhielt er eine Reihe von Preisen, darunter den Gustav-Hertz-Preis 2004 der Deutschen Physikalischen Gesellschaft, den Mattauch-Herzog-Preis 2005 der Deutschen Gesellschaft für Massenspektrometrie, 2012 (zusammen mit seinen Doktoranden Anke Wagner und Sven Sturm) den Helmholtzpreis für Präzisionsmessung, 2013 den G.N. Flerov-Preis und 2016 den Lise Meitner Award des Physik-Zentrums Göteborg. Außerdem wurde er 2008 zum Fellow der American Physical Society ernannt und gewann 2011 einen Advanced Grant des Europäischen Forschungsrats.
________________________________________________________________________________
Mitteilung der Königlich Schwedischen Akademie der Wissenschaften
________________________________________________________________________________
Kontakt
Prof. Dr. Klaus Blaum
MPIK Heidelberg
Tel.: 06221 516850
Pressemitteilung Universität Heidelberg vom 29. November 2018
Prof. Dr. Christof Wetterich, Wissenschaftler am Institut für Theoretische Physik der Universität Heidelberg, wird von der Deutschen Physikalischen Gesellschaft (DPG) ausgezeichnet. Für seine bahnbrechenden Arbeiten auf dem Gebiet der Quantenfeldtheorie erhält er den Gentner-Kastler-Preis. Wie die DPG betont, gilt die Auszeichnung insbesondere der von ihm entwickelten Methode der sogenannten funktionalen Renormierung, die Anwendungen in vielen verschiedenen Gebieten der modernen Physik findet. Der Preis wird gemeinsam mit der Société Francáise de Physique in Erinnerung an zwei herausragende deutsche und französische Physiker, Wolfgang Gentner und Alfred Kastler, für besonders wertvolle wissenschaftliche Beiträge vergeben. Im jährlichen Wechsel geht die Auszeichnung, die mit einem Preisgeld von 3.000 Euro dotiert ist, an einen Physiker aus Deutschland und Frankreich.
Die von Prof. Wetterich formulierte funktionale Renormierung erlaubt es, die Komplexität der makroskopischen Welt auf die „einfachen“ physikalischen Gesetze des Mikrokosmos zurückzuführen. Dadurch wird es möglich, physikalische Fragestellungen in ganz unterschiedlichen Bereichen der Physik zu bearbeiten und zu lösen. So wird diese Methode nicht nur in der Festkörperphysik angewendet, sondern zum Beispiel auch im Bereich der ultrakalten Quantengase oder in der Forschung zur Entwicklung von Strukturen im Universum. Aber auch bei der Suche nach Antworten auf fundamentale Fragestellungen der Theoretischen Physik, wie der Frage nach dem Ursprung und den Eigenschaften der Dunklen Energie, spielt die von Christof Wetterich entwickelte Methode eine wichtige Rolle.
Christof Wetterich studierte Physik in Paris und Köln sowie in Freiburg, wo er auch promoviert wurde und sich später habilitierte. Der Wissenschaftler wirkte am europäischen Forschungszentrum CERN in Genf, an der Universität Bern (Schweiz) sowie am Deutschen Elektronen-Synchrotron DESY in Hamburg. Seit 1992 hat er eine Professur am Institut für Theoretische Physik der Universität Heidelberg inne. Die Verleihung des Gentner-Kastler-Preises findet im kommenden Jahr in Frankreich statt.
________________________________________________________________________________
Prof. Dr. Christof Wetterich
Institut für Theoretische Physik
Tel. +49 6221 54-9340
c.wetterich@thphys.uni-heidelberg.de
Kommunikation und Marketing
Pressestelle
Tel. +49 6221 54-2311
presse@rektorat.uni-heidelberg.de
Pressemitteilung Universität Heidelberg vom 29. November 2018
Prof. Dr. Christof Wetterich, Wissenschaftler am Institut für Theoretische Physik der Universität Heidelberg, wird von der Deutschen Physikalischen Gesellschaft (DPG) ausgezeichnet. Für seine bahnbrechenden Arbeiten auf dem Gebiet der Quantenfeldtheorie erhält er den Gentner-Kastler-Preis. Wie die DPG betont, gilt die Auszeichnung insbesondere der von ihm entwickelten Methode der sogenannten funktionalen Renormierung, die Anwendungen in vielen verschiedenen Gebieten der modernen Physik findet. Der Preis wird gemeinsam mit der Société Francáise de Physique in Erinnerung an zwei herausragende deutsche und französische Physiker, Wolfgang Gentner und Alfred Kastler, für besonders wertvolle wissenschaftliche Beiträge vergeben. Im jährlichen Wechsel geht die Auszeichnung, die mit einem Preisgeld von 3.000 Euro dotiert ist, an einen Physiker aus Deutschland und Frankreich.
Die von Prof. Wetterich formulierte funktionale Renormierung erlaubt es, die Komplexität der makroskopischen Welt auf die „einfachen“ physikalischen Gesetze des Mikrokosmos zurückzuführen. Dadurch wird es möglich, physikalische Fragestellungen in ganz unterschiedlichen Bereichen der Physik zu bearbeiten und zu lösen. So wird diese Methode nicht nur in der Festkörperphysik angewendet, sondern zum Beispiel auch im Bereich der ultrakalten Quantengase oder in der Forschung zur Entwicklung von Strukturen im Universum. Aber auch bei der Suche nach Antworten auf fundamentale Fragestellungen der Theoretischen Physik, wie der Frage nach dem Ursprung und den Eigenschaften der Dunklen Energie, spielt die von Christof Wetterich entwickelte Methode eine wichtige Rolle.
Christof Wetterich studierte Physik in Paris und Köln sowie in Freiburg, wo er auch promoviert wurde und sich später habilitierte. Der Wissenschaftler wirkte am europäischen Forschungszentrum CERN in Genf, an der Universität Bern (Schweiz) sowie am Deutschen Elektronen-Synchrotron DESY in Hamburg. Seit 1992 hat er eine Professur am Institut für Theoretische Physik der Universität Heidelberg inne. Die Verleihung des Gentner-Kastler-Preises findet im kommenden Jahr in Frankreich statt.
________________________________________________________________________________
Prof. Dr. Christof Wetterich
Institut für Theoretische Physik
Tel. +49 6221 54-9340
c.wetterich@thphys.uni-heidelberg.de
Kommunikation und Marketing
Pressestelle
Tel. +49 6221 54-2311
presse@rektorat.uni-heidelberg.de
Press Release No. 143/2018, 26 November 2018
Heidelberg physicist Dr Philipp Hauke has been awarded a highly endowed ERC Starting Grant for excellent young researchers from the European Research Council (ERC). The approx. 1.5 million euros in funding will support his research in the field of quantum mechanics over a period of five years. The physicist is investigating how the entanglement of particles at the microscopic level influences the macroscopic properties of matter. The findings could be useful for new technological applications. His project launches in November 2018. Philipp Hauke is a research group leader at the Kirchhoff Institute for Physics at Heidelberg University.
According to Dr Hauke, groups of particles – the quantum components – in the microscopic world can be brought into an “entangled” state. The individual components relinquish their identity in the process, so every component always “knows” the behaviour of the others. “Major experimental strides in the past several years have made it possible to manipulate matter at the single-component level and recreate complex materials from scratch in order to examine their properties”, states the Heidelberg physicist. “We now know that entanglement at this fundamental level has to play a major role. Yet until now, this entanglement has only been fully understood between very few particles. Furthermore, it is very difficult to detect experimentally in many-body systems.” The “Strong Entanglement in Quantum Many-body Theory” (StrEnQTh) project aims to overcome these hurdles.
Philipp Hauke (b. 1983) studied in Munich and Lausanne (Switzerland), completing his doctorate at the Institute of Photonic Sciences in Barcelona (Spain). He pursued postdoctoral research at the University of Innsbruck (Austria). Dr Hauke has led the “Quantum optics and quantum many-body theory” research group at the Kirchhoff Institute for Physics at Heidelberg University since 2017.
The European Research Council awards the Starting Grant to excellent young researchers. Funding is awarded based on the scientific excellence of the young researchers and the innovative potential of their research ideas.
________________________________________________________________________________
Dr Philipp Hauke
Kirchhoff Institute for Physics
Phone +49 6221 54-5185
philipp.hauke@kip.uni-heidelberg.de
Communications and Marketing
Press Office
Phone +49 6221 54-2311
presse@rektorat.uni-heidelberg.de
Press Release No. 143/2018, 26 November 2018
Heidelberg physicist Dr Philipp Hauke has been awarded a highly endowed ERC Starting Grant for excellent young researchers from the European Research Council (ERC). The approx. 1.5 million euros in funding will support his research in the field of quantum mechanics over a period of five years. The physicist is investigating how the entanglement of particles at the microscopic level influences the macroscopic properties of matter. The findings could be useful for new technological applications. His project launches in November 2018. Philipp Hauke is a research group leader at the Kirchhoff Institute for Physics at Heidelberg University.
According to Dr Hauke, groups of particles – the quantum components – in the microscopic world can be brought into an “entangled” state. The individual components relinquish their identity in the process, so every component always “knows” the behaviour of the others. “Major experimental strides in the past several years have made it possible to manipulate matter at the single-component level and recreate complex materials from scratch in order to examine their properties”, states the Heidelberg physicist. “We now know that entanglement at this fundamental level has to play a major role. Yet until now, this entanglement has only been fully understood between very few particles. Furthermore, it is very difficult to detect experimentally in many-body systems.” The “Strong Entanglement in Quantum Many-body Theory” (StrEnQTh) project aims to overcome these hurdles.
Philipp Hauke (b. 1983) studied in Munich and Lausanne (Switzerland), completing his doctorate at the Institute of Photonic Sciences in Barcelona (Spain). He pursued postdoctoral research at the University of Innsbruck (Austria). Dr Hauke has led the “Quantum optics and quantum many-body theory” research group at the Kirchhoff Institute for Physics at Heidelberg University since 2017.
The European Research Council awards the Starting Grant to excellent young researchers. Funding is awarded based on the scientific excellence of the young researchers and the innovative potential of their research ideas.
________________________________________________________________________________
Dr Philipp Hauke
Kirchhoff Institute for Physics
Phone +49 6221 54-5185
philipp.hauke@kip.uni-heidelberg.de
Communications and Marketing
Press Office
Phone +49 6221 54-2311
presse@rektorat.uni-heidelberg.de
Pressemitteilung Nr. 143/2018, 26. November 2018
Der Heidelberger Physiker Dr. Philipp Hauke erhält eine hochdotierte Förderung des Europäischen Forschungsrates (ERC), einen ERC Starting Grant für herausragende junge Wissenschaftler. Mit den Mitteln in Höhe von rund 1,5 Millionen Euro für einen Zeitraum von fünf Jahren werden seine Forschungen auf dem Gebiet der Quantenmechanik gefördert. Im Zentrum steht dabei die Frage, wie die Verschränkung von Teilchen auf mikroskopischer Ebene die makroskopischen Eigenschaften der Materie beeinflusst. Diese Erkenntnisse sollen für neuartige technologische Anwendungen nutzbar gemacht werden. Sein Projekt startet noch im November dieses Jahres. Philipp Hauke ist Forschungsgruppenleiter am Kirchhoff-Institut für Physik der Universität Heidelberg.
Wie Dr. Hauke erläutert, können in der mikroskopischen Welt Gruppen von Teilchen – die Quantenbausteine – in einen „verschränkten“ Zustand gebracht werden. Dabei geben die einzelnen Bausteine ihre Identität auf, so dass jeder Baustein stets um das Verhalten der anderen „weiß“. „Große experimentelle Fortschritte in den vergangenen Jahren haben es möglich gemacht, Materie auf der Ebene einzelner Bausteine zu manipulieren und komplexe Materialien von Grund auf nachzubauen, um ihre Eigenschaften zu überprüfen“, so der Heidelberger Physiker. „Wir wissen heutzutage, dass Verschränkung auf dieser fundamentalen Ebene eine zentrale Rolle spielen muss. Allerdings ist diese Verschränkung bislang nur zwischen sehr wenigen Teilchen vollkommen verstanden. Zudem ist sie in Vielteilchensystemen experimentell nur sehr schwer nachweisbar.“ Ziel seines ERC Projekts „Strong Entanglement in Quantum Many-body Theory“ (StrEnQTh) ist es, diese Hürden zu überwinden.
Philipp Hauke (Jahrgang 1983) hat in München sowie in Lausanne (Schweiz) studiert. Die Doktorarbeit entstand am Institute of Photonic Sciences in Barcelona (Spanien). Als Postdoktorand wirkte er an der Universität Innsbruck (Österreich). Seit 2017 ist Dr. Hauke Leiter der Forschung „Quantenoptik und Quantenvielteilchentheorie“ am Kirchhoff-Institut für Physik der Universität Heidelberg.
Der Europäische Forschungsrat vergibt den Starting Grant an herausragende junge Wissenschaftlerinnen und Wissenschaftler. Kriterien für die Förderung sind die wissenschaftliche Exzellenz der Nachwuchsforscher und das innovative Potential ihrer Forschungsideen.
________________________________________________________________________________
Dr. Philipp Hauke
Kirchhoff-Institut für Physik
Tel. +49 6221 54-5185
philipp.hauke@kip.uni-heidelberg.de
Kommunikation und Marketing
Pressestelle
Tel. +49 6221 54-2311
presse@rektorat.uni-heidelberg.de
Pressemitteilung Nr. 143/2018, 26. November 2018
Der Heidelberger Physiker Dr. Philipp Hauke erhält eine hochdotierte Förderung des Europäischen Forschungsrates (ERC), einen ERC Starting Grant für herausragende junge Wissenschaftler. Mit den Mitteln in Höhe von rund 1,5 Millionen Euro für einen Zeitraum von fünf Jahren werden seine Forschungen auf dem Gebiet der Quantenmechanik gefördert. Im Zentrum steht dabei die Frage, wie die Verschränkung von Teilchen auf mikroskopischer Ebene die makroskopischen Eigenschaften der Materie beeinflusst. Diese Erkenntnisse sollen für neuartige technologische Anwendungen nutzbar gemacht werden. Sein Projekt startet noch im November dieses Jahres. Philipp Hauke ist Forschungsgruppenleiter am Kirchhoff-Institut für Physik der Universität Heidelberg.
Wie Dr. Hauke erläutert, können in der mikroskopischen Welt Gruppen von Teilchen – die Quantenbausteine – in einen „verschränkten“ Zustand gebracht werden. Dabei geben die einzelnen Bausteine ihre Identität auf, so dass jeder Baustein stets um das Verhalten der anderen „weiß“. „Große experimentelle Fortschritte in den vergangenen Jahren haben es möglich gemacht, Materie auf der Ebene einzelner Bausteine zu manipulieren und komplexe Materialien von Grund auf nachzubauen, um ihre Eigenschaften zu überprüfen“, so der Heidelberger Physiker. „Wir wissen heutzutage, dass Verschränkung auf dieser fundamentalen Ebene eine zentrale Rolle spielen muss. Allerdings ist diese Verschränkung bislang nur zwischen sehr wenigen Teilchen vollkommen verstanden. Zudem ist sie in Vielteilchensystemen experimentell nur sehr schwer nachweisbar.“ Ziel seines ERC Projekts „Strong Entanglement in Quantum Many-body Theory“ (StrEnQTh) ist es, diese Hürden zu überwinden.
Philipp Hauke (Jahrgang 1983) hat in München sowie in Lausanne (Schweiz) studiert. Die Doktorarbeit entstand am Institute of Photonic Sciences in Barcelona (Spanien). Als Postdoktorand wirkte er an der Universität Innsbruck (Österreich). Seit 2017 ist Dr. Hauke Leiter der Forschung „Quantenoptik und Quantenvielteilchentheorie“ am Kirchhoff-Institut für Physik der Universität Heidelberg.
Der Europäische Forschungsrat vergibt den Starting Grant an herausragende junge Wissenschaftlerinnen und Wissenschaftler. Kriterien für die Förderung sind die wissenschaftliche Exzellenz der Nachwuchsforscher und das innovative Potential ihrer Forschungsideen.
________________________________________________________________________________
Dr. Philipp Hauke
Kirchhoff-Institut für Physik
Tel. +49 6221 54-5185
philipp.hauke@kip.uni-heidelberg.de
Kommunikation und Marketing
Pressestelle
Tel. +49 6221 54-2311
presse@rektorat.uni-heidelberg.de
Pressemitteilung des MPIK Heidelberg, 15. November 2018
Für ihre wegweisenden theoretischen Untersuchungen von Quanteneffekten in der Wechselwirkung von Röntgenstrahlung mit Atomkernen erhält PD Dr. Adriana Pálffy-Buß den Hertha-Sponer-Preis der Deutschen Physikalischen Gesellschaft für das Jahr 2019.
Der Hertha-Sponer-Preis wird für hervorragende wissenschaftliche Arbeiten auf dem Gebiet der Physik an eine Wissenschaftlerin vergeben und soll vor allem jüngere Wissenschaftlerinnen durch öffentliche Auszeichnung ermutigen und so mehr Frauen für die Physik gewinnen. Er ist mit einem Preisgeld von 3000 € und einer Urkunde dotiert. Gleichzeitig ist die Preisträgerin eingeladen, während der Jahrestagung einen Vortrag über Ihr Arbeitsgebiet zu halten. Die Preisverleihung findet im Rahmen eines feierlichen Abendessens am 12. März 2019 in Rostock statt.
Nach dem Physikstudium an der Universität Bukarest promovierte Adriana Pálffy-Buß 2006 an der Justus-Liebig-Universität Gießen. Anschließend wechselte sie an das MPI für Kernphysik in die Abteilung von Christoph Keitel, wo sie – unterbrochen von Kurzaufenthalten an der University of Strathclyde in Glasgow – seit 2011 die Gruppe „Nukleare und atomare Quantendynamik“ leitet. 2014 habilitierte sie an der Universität Heidelberg. Adriana Pálffy-Buß hat in einer Reihe von theoretischen Pionierarbeiten untersucht, wie man Atomkerne für neuartige Anwendungen in der Röntgenquantenoptik verwenden kann. Ihre Vorhersagen über die Erzielung einer verstärkten Wechselwirkung zwischen Strahlung und Atomkernen, der sogenannten “starken Kopplung”, welche in der Quantenoptik als Grundstein vieler Quanteneffekte bekannt ist, wurde vor kurzem experimentell in nanoskopischen Systemen bestätigt.
_____________________________________________
Neue Kontrollmöglichkeiten mit und für Röntgenlicht, Max-Planck-Jahrbuch 2017
Elektronen wirksamer als Röntgenlaser (Meldung vom 24.02.2014)
Pressemitteilung Nr. 280, Justus-Liebig-Universität Gießen, 18. November 2019
________________________________________________________________________________
Kontakt:
PD Dr. Adriana Pálffy-Buß
E-Mail: palffy@mpi-hd.mpg.de
Tel.: 06221 516171
Pressemitteilung des MPIK Heidelberg, 15. November 2018
Für ihre wegweisenden theoretischen Untersuchungen von Quanteneffekten in der Wechselwirkung von Röntgenstrahlung mit Atomkernen erhält PD Dr. Adriana Pálffy-Buß den Hertha-Sponer-Preis der Deutschen Physikalischen Gesellschaft für das Jahr 2019.
Der Hertha-Sponer-Preis wird für hervorragende wissenschaftliche Arbeiten auf dem Gebiet der Physik an eine Wissenschaftlerin vergeben und soll vor allem jüngere Wissenschaftlerinnen durch öffentliche Auszeichnung ermutigen und so mehr Frauen für die Physik gewinnen. Er ist mit einem Preisgeld von 3000 € und einer Urkunde dotiert. Gleichzeitig ist die Preisträgerin eingeladen, während der Jahrestagung einen Vortrag über Ihr Arbeitsgebiet zu halten. Die Preisverleihung findet im Rahmen eines feierlichen Abendessens am 12. März 2019 in Rostock statt.
Nach dem Physikstudium an der Universität Bukarest promovierte Adriana Pálffy-Buß 2006 an der Justus-Liebig-Universität Gießen. Anschließend wechselte sie an das MPI für Kernphysik in die Abteilung von Christoph Keitel, wo sie – unterbrochen von Kurzaufenthalten an der University of Strathclyde in Glasgow – seit 2011 die Gruppe „Nukleare und atomare Quantendynamik“ leitet. 2014 habilitierte sie an der Universität Heidelberg. Adriana Pálffy-Buß hat in einer Reihe von theoretischen Pionierarbeiten untersucht, wie man Atomkerne für neuartige Anwendungen in der Röntgenquantenoptik verwenden kann. Ihre Vorhersagen über die Erzielung einer verstärkten Wechselwirkung zwischen Strahlung und Atomkernen, der sogenannten “starken Kopplung”, welche in der Quantenoptik als Grundstein vieler Quanteneffekte bekannt ist, wurde vor kurzem experimentell in nanoskopischen Systemen bestätigt.
________________________________________________________________________________
Neue Kontrollmöglichkeiten mit und für Röntgenlicht, Max-Planck-Jahrbuch 2017
Elektronen wirksamer als Röntgenlaser (Meldung vom 24.02.2014)
Pressemitteilung Nr. 280, Justus-Liebig-Universität Gießen, 18. November 2019
________________________________________________________________________________
Kontakt:
PD Dr. Adriana Pálffy-Buß
E-Mail: palffy@mpi-hd.mpg.de
Tel.: 06221 516171
Pressemitteilung Nr. 136/2018, 15. November 2018
Mit der wichtigsten Auszeichnung, die die Deutsche Physikalische Gesellschaft (DPG) auf dem Gebiet der experimentellen Physik vergibt, wird die Heidelberger Wissenschaftlerin Prof. Dr. Johanna Stachel ausgezeichnet. Sie erhält die Stern-Gerlach-Medaille gemeinsam mit Prof. Dr. Peter Braun-Munzinger vom GSI Helmholtzzentrum für Schwerionenforschung in Darmstadt. Die DPG würdigt damit die herausragenden Beiträge des Forscherehepaares zur Interpretation von Schwerionenkollisionen und zum Verständnis der Phasenstruktur von Materie unter extremen Bedingungen ebenso wie die federführenden Arbeiten in der Entwicklung und Etablierung experimenteller Techniken. Johanna Stachel lehrt und forscht als Professorin für Experimentalphysik am Physikalischen Institut der Universität Heidelberg; Peter Braun-Munzinger ist Honorarprofessor an der Ruperto Carola.
Am europäischen Forschungszentrum CERN in Genf ist Prof. Stachel maßgeblich an den Experimenten mit dem Teilchenbeschleuniger LHC und der ALICE-Kollaboration beteiligt. Im Mittelpunkt der Forschung steht das Quark-Gluon-Plasma. Aus diesem Materiezustand, der unmittelbar nach dem Urknall den Kosmos erfüllte, entwickelten sich einst die Grundbausteine der Materie und schließlich alles, was wir heute im Universum vorfinden. Der Large Hadron Collider (LHC) macht es möglich, diese kosmische „Ursuppe“ nachzubilden und zu untersuchen. Nach der Mitwirkung an Bau und Betrieb zentraler Detektoren für das Experiment ALICE haben Prof. Stachel und Prof. Braun-Munzinger in einer Serie von Veröffentlichungen zentrale Erkenntnisse aus ihren langjährigen Arbeiten zur Natur des Phasenübergangs vorgestellt. Gemeinsam mit Kollegen konnten sie mit Präzision die Übergangstemperatur bestimmen. Zudem gewannen sie neue Einblicke in den Mechanismus, wie ein Quark-Gluon-Plasma beim Abkühlen in die normalen Materiebausteine wie Protonen, Neutronen oder Atomkerne ausfriert. Dabei gelang auch der Nachweis eines von Johanna Stachel und Peter Braun-Munzinger vorhergesagten Phänomens. Für ihre gemeinsam erbrachten, herausragenden Forschungsleistungen in der Hochenergie-Schwerionenforschung werden die beiden Wissenschaftler nun geehrt.
Johanna Stachel studierte Chemie und Physik und wurde an der Universität Mainz promoviert. Im Anschluss forschte sie dreizehn Jahre in den USA an der Stony Brook University und dem Brookhaven National Laboratory. 1996 wurde sie an die Universität Heidelberg berufen. Für ihre Arbeiten erhielt sie mehrere Forschungspreise, im Jahr 1999 wurde sie mit dem Bundesverdienstkreuz ausgezeichnet. Prof. Stachel hat in zahlreichen wissenschaftlichen Gremien wie dem Scientific Policy Committee des CERN und dem US-amerikanischen Nuclear Science Advisory Committee mitgewirkt und engagiert sich auch aktuell in vielfältiger Weise in Einrichtungen der Wissenschaftsförderung. Von 2012 bis 2014 war sie Präsidentin der Deutschen Physikalischen Gesellschaft – als erste Frau seit Gründung der DPG im Jahr 1845. Diese ist die älteste nationale und mit mehr als 60.000 Mitgliedern auch größte physikalische Fachgesellschaft der Welt.
________________________________________________________________________________
Videos:
Stern-Gerlach-Medaille 2019 – Interview: Prof. Dr. Johanna Stachel & Prof. Dr. Peter Braun-Munzinger
Stern-Gerlach-Medaille 2019 – Preisträgervortrag von Prof. Dr. Johanna Stachel
Stern-Gerlach-Medaille 2019 – Preisträgervortrag von Prof. Dr. Peter Braun-Munzinger
________________________________________________________________________________
Prof. Dr. Johanna Stachel
Physikalisches Institut
INF 226, 69120 Heidelberg
T: +49 6221 54 19501
stachel@physi.uni-heidelberg.de
Kommunikation und Marketing
Pressestelle
Tel. +49 6221 54-2311
presse@rektorat.uni-heidelberg.de
Pressemitteilung Nr. 136/2018, 15. November 2018
Mit der wichtigsten Auszeichnung, die die Deutsche Physikalische Gesellschaft (DPG) auf dem Gebiet der experimentellen Physik vergibt, wird die Heidelberger Wissenschaftlerin Prof. Dr. Johanna Stachel ausgezeichnet. Sie erhält die Stern-Gerlach-Medaille gemeinsam mit Prof. Dr. Peter Braun-Munzinger vom GSI Helmholtzzentrum für Schwerionenforschung in Darmstadt. Die DPG würdigt damit die herausragenden Beiträge des Forscherehepaares zur Interpretation von Schwerionenkollisionen und zum Verständnis der Phasenstruktur von Materie unter extremen Bedingungen ebenso wie die federführenden Arbeiten in der Entwicklung und Etablierung experimenteller Techniken. Johanna Stachel lehrt und forscht als Professorin für Experimentalphysik am Physikalischen Institut der Universität Heidelberg; Peter Braun-Munzinger ist Honorarprofessor an der Ruperto Carola.
Am europäischen Forschungszentrum CERN in Genf ist Prof. Stachel maßgeblich an den Experimenten mit dem Teilchenbeschleuniger LHC und der ALICE-Kollaboration beteiligt. Im Mittelpunkt der Forschung steht das Quark-Gluon-Plasma. Aus diesem Materiezustand, der unmittelbar nach dem Urknall den Kosmos erfüllte, entwickelten sich einst die Grundbausteine der Materie und schließlich alles, was wir heute im Universum vorfinden. Der Large Hadron Collider (LHC) macht es möglich, diese kosmische „Ursuppe“ nachzubilden und zu untersuchen. Nach der Mitwirkung an Bau und Betrieb zentraler Detektoren für das Experiment ALICE haben Prof. Stachel und Prof. Braun-Munzinger in einer Serie von Veröffentlichungen zentrale Erkenntnisse aus ihren langjährigen Arbeiten zur Natur des Phasenübergangs vorgestellt. Gemeinsam mit Kollegen konnten sie mit Präzision die Übergangstemperatur bestimmen. Zudem gewannen sie neue Einblicke in den Mechanismus, wie ein Quark-Gluon-Plasma beim Abkühlen in die normalen Materiebausteine wie Protonen, Neutronen oder Atomkerne ausfriert. Dabei gelang auch der Nachweis eines von Johanna Stachel und Peter Braun-Munzinger vorhergesagten Phänomens. Für ihre gemeinsam erbrachten, herausragenden Forschungsleistungen in der Hochenergie-Schwerionenforschung werden die beiden Wissenschaftler nun geehrt.
Johanna Stachel studierte Chemie und Physik und wurde an der Universität Mainz promoviert. Im Anschluss forschte sie dreizehn Jahre in den USA an der Stony Brook University und dem Brookhaven National Laboratory. 1996 wurde sie an die Universität Heidelberg berufen. Für ihre Arbeiten erhielt sie mehrere Forschungspreise, im Jahr 1999 wurde sie mit dem Bundesverdienstkreuz ausgezeichnet. Prof. Stachel hat in zahlreichen wissenschaftlichen Gremien wie dem Scientific Policy Committee des CERN und dem US-amerikanischen Nuclear Science Advisory Committee mitgewirkt und engagiert sich auch aktuell in vielfältiger Weise in Einrichtungen der Wissenschaftsförderung. Von 2012 bis 2014 war sie Präsidentin der Deutschen Physikalischen Gesellschaft – als erste Frau seit Gründung der DPG im Jahr 1845. Diese ist die älteste nationale und mit mehr als 60.000 Mitgliedern auch größte physikalische Fachgesellschaft der Welt.
________________________________________________________________________________
Videos:
Stern-Gerlach-Medaille 2019 – Interview: Prof. Dr. Johanna Stachel & Prof. Dr. Peter Braun-Munzinger
Stern-Gerlach-Medaille 2019 – Preisträgervortrag von Prof. Dr. Johanna Stachel
Stern-Gerlach-Medaille 2019 – Preisträgervortrag von Prof. Dr. Peter Braun-Munzinger
________________________________________________________________________________
Prof. Dr. Johanna Stachel
Physikalisches Institut
INF 226, 69120 Heidelberg
T: +49 6221 54 19501
stachel@physi.uni-heidelberg.de
Kommunikation und Marketing
Pressestelle
Tel. +49 6221 54-2311
presse@rektorat.uni-heidelberg.de
Press Release No. 132/2018, 9 November 2018
Different physical systems – isolated from the environment and far from equilibrium – can behave the same. Quantum mechanically, this is the case if the dynamics of a many-particle system become universal. In two fundamentally different experiments, physicists from Heidelberg University and the TU Vienna (Austria) have now succeeded in verifying this universality in ultracold clouds of Rubidium atoms. The improved understanding of the systems studied permit making predictions for totally different systems. The research results were published in “Nature”.
The expansion of the Universe directly after the Big Bang, the ramifications of a heavy-ion collision in the particle accelerator at CERN, or clouds of atoms at ultracold temperatures – many isolated physical systems are naturally out of equilibrium, yet the relevant quantities are often hard to measure. This is different for ultracold atomic gases. “The high degree of control over our system allows us to set up interesting initial conditions. In the course of the dynamics we are able to measure precisely the universal observables, for example the state of the atoms, spatially resolved“, explains Prof. Dr Markus Oberthaler, founder of the Synthetic Quantum Systems research group at Heidelberg University’s Kirchhoff Institute for Physics.
In their experiment, the Heidelberg researchers prepared about 70,000 ultracold Rubidium atoms. They were thrown out of equilibrium by a rapid change in an external magnetic field. At ultracold temperatures around 10 nanokelvins and in perfect isolation from the environment, the atoms behave like magnets that interact with each other. The characteristics of the universal dynamics become observable only after – for experiments with ultracold atoms – long evolution times. “This requires an extremely stable experimental setup, but it does allow us to investigate the dynamics very precisely”, says Maximilian Prüfer, the primary author of the study.
The research group of Prof. Dr Jörg Schmiedmayer at the TU Vienna observed another type of universal dynamics. “Universality provides a new method to gain essential information about quantum systems usually not accessible in the laboratory“, says Jörg Schmiedmayer. Prof. Dr Thomas Gasenzer of the Kirchhoff Institute for Physics and Prof. Dr Jürgen Berges of the Institute for Theoretical Physics at Ruperto Carola were involved in these investigations. They are also co-authors of the Heidelberg study under the direction of Prof. Oberthaler.
The characterisation of quantum mechanical many-particle systems far from equilibrium is very important, for example, for understanding structure formation in nature. Markus Oberthaler emphasises: “The impressive result of both experiments is that they found two different universality classes. This suggests that we are on the track of a fundamental structure.” The work in Heidelberg and Vienna was conducted under the auspices of the “Isolated quantum systems and universality in extreme conditions” Collaborative Research Centre at Heidelberg University.
________________________________________________________________________________
Original publications:
M. Prüfer, P. Kunkel, H. Strobel, S. Lannig, D. Linnemann, C.M. Schmied, J. Berges, T. Gasenzer, and M.K.
Oberthaler: Observation of universal dynamics in a spinor Bose gas far from equilibrium, Nature 563, 217-220
(2018)
DOI:10.1038/s41586-018-0659-0
S. Erne, R. Bücker, T. Gasenzer, J. Berges, and J. Schmiedmayer: Universal dynamics in an isolated onedimensional
Bose gas far from equilibrium, Nature 563, 225-229 (2018) DOI: 10.1038/s41586-018-0667-0
________________________________________________________________________________
Prof. Dr. Markus Oberthaler
Kirchhoff Institute for Physics
Phone +49 6221 54-5170
markus.oberthaler@kip.uni-heidelberg.de
Communications and Marketing
Press Office
Phone +49 6221 54-2311
presse@rektorat.uni-heidelberg.de
Press Release No. 132/2018, 9 November 2018
Different physical systems – isolated from the environment and far from equilibrium – can behave the same. Quantum mechanically, this is the case if the dynamics of a many-particle system become universal. In two fundamentally different experiments, physicists from Heidelberg University and the TU Vienna (Austria) have now succeeded in verifying this universality in ultracold clouds of Rubidium atoms. The improved understanding of the systems studied permit making predictions for totally different systems. The research results were published in “Nature”.
The expansion of the Universe directly after the Big Bang, the ramifications of a heavy-ion collision in the particle accelerator at CERN, or clouds of atoms at ultracold temperatures – many isolated physical systems are naturally out of equilibrium, yet the relevant quantities are often hard to measure. This is different for ultracold atomic gases. “The high degree of control over our system allows us to set up interesting initial conditions. In the course of the dynamics we are able to measure precisely the universal observables, for example the state of the atoms, spatially resolved“, explains Prof. Dr Markus Oberthaler, founder of the Synthetic Quantum Systems research group at Heidelberg University’s Kirchhoff Institute for Physics.
In their experiment, the Heidelberg researchers prepared about 70,000 ultracold Rubidium atoms. They were thrown out of equilibrium by a rapid change in an external magnetic field. At ultracold temperatures around 10 nanokelvins and in perfect isolation from the environment, the atoms behave like magnets that interact with each other. The characteristics of the universal dynamics become observable only after – for experiments with ultracold atoms – long evolution times. “This requires an extremely stable experimental setup, but it does allow us to investigate the dynamics very precisely”, says Maximilian Prüfer, the primary author of the study.
The research group of Prof. Dr Jörg Schmiedmayer at the TU Vienna observed another type of universal dynamics. “Universality provides a new method to gain essential information about quantum systems usually not accessible in the laboratory“, says Jörg Schmiedmayer. Prof. Dr Thomas Gasenzer of the Kirchhoff Institute for Physics and Prof. Dr Jürgen Berges of the Institute for Theoretical Physics at Ruperto Carola were involved in these investigations. They are also co-authors of the Heidelberg study under the direction of Prof. Oberthaler.
The characterisation of quantum mechanical many-particle systems far from equilibrium is very important, for example, for understanding structure formation in nature. Markus Oberthaler emphasises: “The impressive result of both experiments is that they found two different universality classes. This suggests that we are on the track of a fundamental structure.” The work in Heidelberg and Vienna was conducted under the auspices of the “Isolated quantum systems and universality in extreme conditions” Collaborative Research Centre at Heidelberg University.
________________________________________________________________________________
Original publications:
M. Prüfer, P. Kunkel, H. Strobel, S. Lannig, D. Linnemann, C.M. Schmied, J. Berges, T. Gasenzer, and M.K.
Oberthaler: Observation of universal dynamics in a spinor Bose gas far from equilibrium, Nature 563, 217-220
(2018)
DOI:10.1038/s41586-018-0659-0
S. Erne, R. Bücker, T. Gasenzer, J. Berges, and J. Schmiedmayer: Universal dynamics in an isolated onedimensional
Bose gas far from equilibrium, Nature 563, 225-229 (2018) DOI: 10.1038/s41586-018-0667-0
________________________________________________________________________________
Prof. Dr. Markus Oberthaler
Kirchhoff Institute for Physics
Phone +49 6221 54-5170
markus.oberthaler@kip.uni-heidelberg.de
Communications and Marketing
Press Office
Phone +49 6221 54-2311
presse@rektorat.uni-heidelberg.de
Pressemitteilung Nr. 132/2018, 9. November 2018
Unterschiedliche physikalische Systeme – in sich abgeschlossen und fern des Gleichgewichts – können sich vergleichbar verhalten. Das ist in der Quantenmechanik dann der Fall, wenn die Dynamik dieser Vielteilchensysteme universell wird. Physikern der Universität Heidelberg und der Technischen Universität Wien (Österreich) ist es jetzt gelungen, in zwei grundverschiedenen Experimenten eine solche Universalität in ultrakalten Wolken von Rubidium-Atomen nachzuweisen. Dies erlaubt es, das bessere Verständnis des untersuchten Systems zu nutzen, um Vorhersagen über ganz andere Systeme zu treffen. Die Forschungsergebnisse wurden in „Nature“ veröffentlicht.
Die Expansion des Universums unmittelbar nach dem Urknall, die Folgen einer Kollision schwerer Ionen im Teilchenbeschleuniger des Forschungszentrums CERN oder Wolken von Atomen bei extrem niedrigen Temperaturen – viele abgeschlossene physikalische Systeme sind natürlicherweise nicht im Gleichgewicht, jedoch sind die relevanten Größen oft nur schwer messbar. Das ist im Fall ultrakalter Atomgase anders. „Die hohe Kontrolle über unser System erlaubt es uns, gezielt interessante Anfangszustände zu erzeugen und im Laufe der Dynamik die universellen Messgrößen, zum Beispiel den Zustand der Atome, an jedem Ort präzise auszulesen“, erläutert Prof. Dr. Markus Oberthaler, Gründer der Forschungsgruppe Synthetische Quantensysteme am Kirchhoff-Institut für Physik der Universität Heidelberg.
In ihrem Experiment präparierten die Heidelberger Forscher rund 70.000 ultrakalte Rubidium-Atome. Diese wurden durch das schnelle Ändern eines von außen angelegten Magnetfeldes aus dem Gleichgewicht gebracht. Bei ultrakalten Temperaturen von etwa zehn Nanokelvin und perfekter Isolation von der Umgebung verhalten sich die Atome wie Magnete, die miteinander in Wechselwirkung treten. Dabei lassen sich die Charakteristiken der universellen Dynamik erst nach – für Experimente mit ultrakalten Atomen – langen Wartezeiten beobachten. „Dies erfordert eine hohe Stabilität des experimentellen Aufbaus, erlaubt es uns jedoch, die Dynamik genau zu untersuchen“, so der Erstautor der Studie, Maximilian Prüfer.
Einen weiteren Typ universeller Dynamik hat die Forschungsgruppe von Prof. Dr. Jörg Schmiedmayer an der Technischen Universität Wien beobachtet. „Universalität bedeutet, dass man nun eine neue Methode zur Verfügung hat, um wichtige Informationen über normalerweise im Labor unzugängliche Quantensysteme zu erhalten“, so Jörg Schmiedmayer. Beteiligt an den Untersuchungen waren Prof. Dr. Thomas Gasenzer vom Kirchhoff-Institut für Physik und Prof. Dr. Jürgen Berges vom Institut für Theoretische Physik der Ruperto Carola, die auch Co-Autoren der Heidelberger Studie unter der Leitung von Prof. Oberthaler sind.
Die Charakterisierung von quantenmechanischen Vielteilchensystemen fern des Gleichgewichts ist unter anderem für das Verständnis der Strukturbildung in der Natur von besonderer Bedeutung. Markus Oberthaler betont: „Das beeindruckende Ergebnis der beiden Experimente ist, dass wir zwei verschiedene Universalitätsklassen gefunden haben. Das legt nahe, dass wir einer fundamentalen Struktur auf der Spur sind.“ Die Arbeiten in Heidelberg und Wien wurden im Rahmen des Sonderforschungsbereichs „Quantensysteme unter extremen Bedingungen im Fokus von Experiment und Theorie“ der Universität Heidelberg durchgeführt.
________________________________________________________________________________
Originalpublikationen:
M. Prüfer, P. Kunkel, H. Strobel, S. Lannig, D. Linnemann, C.M. Schmied, J. Berges, T. Gasenzer, and M.K.
Oberthaler: Observation of universal dynamics in a spinor Bose gas far from equilibrium, Nature 563, 217-220
(2018)
DOI:10.1038/s41586-018-0659-0
S. Erne, R. Bücker, T. Gasenzer, J. Berges, and J. Schmiedmayer: Universal dynamics in an isolated onedimensional
Bose gas far from equilibrium, Nature 563, 225-229 (2018) DOI: 10.1038/s41586-018-0667-0
________________________________________________________________________________
Prof. Dr. Markus Oberthaler
Kirchhoff-Institut für Physik
Tel. +49 6221 54-5170
markus.oberthaler@kip.uni-heidelberg.de
Kommunikation und Marketing
Pressestelle
Tel. +49 6221 54-2311
presse@rektorat.uni-heidelberg.de
Pressemitteilung Nr. 132/2018, 9. November 2018
Unterschiedliche physikalische Systeme – in sich abgeschlossen und fern des Gleichgewichts – können sich vergleichbar verhalten. Das ist in der Quantenmechanik dann der Fall, wenn die Dynamik dieser Vielteilchensysteme universell wird. Physikern der Universität Heidelberg und der Technischen Universität Wien (Österreich) ist es jetzt gelungen, in zwei grundverschiedenen Experimenten eine solche Universalität in ultrakalten Wolken von Rubidium-Atomen nachzuweisen. Dies erlaubt es, das bessere Verständnis des untersuchten Systems zu nutzen, um Vorhersagen über ganz andere Systeme zu treffen. Die Forschungsergebnisse wurden in „Nature“ veröffentlicht.
Die Expansion des Universums unmittelbar nach dem Urknall, die Folgen einer Kollision schwerer Ionen im Teilchenbeschleuniger des Forschungszentrums CERN oder Wolken von Atomen bei extrem niedrigen Temperaturen – viele abgeschlossene physikalische Systeme sind natürlicherweise nicht im Gleichgewicht, jedoch sind die relevanten Größen oft nur schwer messbar. Das ist im Fall ultrakalter Atomgase anders. „Die hohe Kontrolle über unser System erlaubt es uns, gezielt interessante Anfangszustände zu erzeugen und im Laufe der Dynamik die universellen Messgrößen, zum Beispiel den Zustand der Atome, an jedem Ort präzise auszulesen“, erläutert Prof. Dr. Markus Oberthaler, Gründer der Forschungsgruppe Synthetische Quantensysteme am Kirchhoff-Institut für Physik der Universität Heidelberg.
In ihrem Experiment präparierten die Heidelberger Forscher rund 70.000 ultrakalte Rubidium-Atome. Diese wurden durch das schnelle Ändern eines von außen angelegten Magnetfeldes aus dem Gleichgewicht gebracht. Bei ultrakalten Temperaturen von etwa zehn Nanokelvin und perfekter Isolation von der Umgebung verhalten sich die Atome wie Magnete, die miteinander in Wechselwirkung treten. Dabei lassen sich die Charakteristiken der universellen Dynamik erst nach – für Experimente mit ultrakalten Atomen – langen Wartezeiten beobachten. „Dies erfordert eine hohe Stabilität des experimentellen Aufbaus, erlaubt es uns jedoch, die Dynamik genau zu untersuchen“, so der Erstautor der Studie, Maximilian Prüfer.
Einen weiteren Typ universeller Dynamik hat die Forschungsgruppe von Prof. Dr. Jörg Schmiedmayer an der Technischen Universität Wien beobachtet. „Universalität bedeutet, dass man nun eine neue Methode zur Verfügung hat, um wichtige Informationen über normalerweise im Labor unzugängliche Quantensysteme zu erhalten“, so Jörg Schmiedmayer. Beteiligt an den Untersuchungen waren Prof. Dr. Thomas Gasenzer vom Kirchhoff-Institut für Physik und Prof. Dr. Jürgen Berges vom Institut für Theoretische Physik der Ruperto Carola, die auch Co-Autoren der Heidelberger Studie unter der Leitung von Prof. Oberthaler sind.
Die Charakterisierung von quantenmechanischen Vielteilchensystemen fern des Gleichgewichts ist unter anderem für das Verständnis der Strukturbildung in der Natur von besonderer Bedeutung. Markus Oberthaler betont: „Das beeindruckende Ergebnis der beiden Experimente ist, dass wir zwei verschiedene Universalitätsklassen gefunden haben. Das legt nahe, dass wir einer fundamentalen Struktur auf der Spur sind.“ Die Arbeiten in Heidelberg und Wien wurden im Rahmen des Sonderforschungsbereichs „Quantensysteme unter extremen Bedingungen im Fokus von Experiment und Theorie“ der Universität Heidelberg durchgeführt.
________________________________________________________________________________
Originalpublikationen:
M. Prüfer, P. Kunkel, H. Strobel, S. Lannig, D. Linnemann, C.M. Schmied, J. Berges, T. Gasenzer, and M.K.
Oberthaler: Observation of universal dynamics in a spinor Bose gas far from equilibrium, Nature 563, 217-220
(2018)
DOI:10.1038/s41586-018-0659-0
S. Erne, R. Bücker, T. Gasenzer, J. Berges, and J. Schmiedmayer: Universal dynamics in an isolated onedimensional
Bose gas far from equilibrium, Nature 563, 225-229 (2018) DOI: 10.1038/s41586-018-0667-0
________________________________________________________________________________
Prof. Dr. Markus Oberthaler
Kirchhoff-Institut für Physik
Tel. +49 6221 54-5170
markus.oberthaler@kip.uni-heidelberg.de
Kommunikation und Marketing
Pressestelle
Tel. +49 6221 54-2311
presse@rektorat.uni-heidelberg.de
Press release of TU Wien, 08 November 2018
When quantum particles swirl about, they still obey universal laws. Different quantum systems can show the same behaviour – this has been demonstrated by two different experiments at TU Wien and Heidelberg University.
Some things are so complicated that it is completely impossible to precisely calculate them. This includes large quantum systems, which consist of many particles, particularly when they are not in an equilibrium state, but changing rapidly. Such examples include the wild particle inferno that occurs in particle accelerators when large atoms collide, or in the early universe, just after the Big Bang, when particles rapidly expanded and subsequently cooled.
At TU Wien and Heidelberg University, remarkable rules have been detected in the apparent chaos of disequilibrium processes. This indicates that such processes can be divided into universality classes. Systems belonging to the same class behave identically in many ways. This means that experiments can be carried out with quantum systems that are easy to handle, in order to obtain precise information about other systems that cannot be directly studied in the experiment. These findings have since been published in the journal ‘Nature’.
Universal rules
“Universality classes are known from other areas of physics,” says Prof. Jörg Schmiedmayer from the Institute of Atomic and Subatomic Physics at TU Wien. “When you study phase transitions, for example materials very close to the melting point, you can describe certain properties using formulas that are very universal, such as the relationship between the specific heat and the temperature.” The microscopic details of the melting process do not matter. Very different materials can obey the same simple equations.
“It is however entirely astounding that universality of this kind can also be found in quantum systems that are far removed from an equilibrium state,” says Jörg Schmiedmayer. “At first glance, you wouldn’t expect this: why should a quantum system made up of many particles that are changing extremely rapidly obey any universal laws?” Nevertheless, theoretical work from Jürgen Berges and Thomas Gasenzer’s groups from Heidelberg University predicted exactly that. These notable predictions have now been verified twice at the same time – at TU Wien and in Heidelberg.
The quick and the slow direction
The experiment in Prof. Schmiedmayer’s group at the Vienna Center for Quantum Science and Technology (VCQ) at the Institute of Atomic and Subatomic Physics (TU Wien) is using a very special atom trap. On an atom chip, thousands of rubidium atoms can be trapped and cooled using electromagnetic fields. “In this process, we generate an atom cloud with a short and a long direction, similar to a cigar,” explains Sebastian Erne, the lead author of the study.
Initially, the atoms move in all directions at the same speed. The atom trap can however be opened in the short (transverse) directions, meaning that those atoms that are moving particularly fast in this direction fly away. This leaves behind only atoms that have a relatively low speed in the transverse directions.
“The speed distribution in one direction is changed so quickly that during this time, the speed distribution in the other direction, along the longer axis of the cigar, virtually does not change at all,” says Sebastian Erne. “As a result, we produce a state that is far from the thermal equilibrium.” Collisions and interactions then lead to energy exchange between the atoms, which is referred to as ‘thermalisation’.
“Our experiment demonstrates that the course of this thermalisation follows a universal law and is not dependent on any details,” says Jörg Schmiedmayer. “Regardless of how we started the thermalisation, the transition can always be described with the same formula.”
It was a similar story for the research team from Heidelberg. There too, they started out with an elongated atom cloud. However, the Heidelberg team did not study the speed but the spin (the intrinsic angular momentum) of the particles. They first controlled the spin directions of the atoms and then observed how these directions change over time due to interactions between the atoms.
This change can be described using the same formulas as the one from the other experiment: “In our case, the physical situation is quite different from that of the TU Wien experiment, but the dynamics also obey universal scaling laws,” explains Maximilian Prüfer (Heidelberg), first author of Heidelberg publication. “We have found a process that also obeys the universality but belongs to a different universality class. This is great because it confirms our theories very convincingly and suggests that we really are on to something – a new, fundamental law, ” says Markus Oberthaler (also Heidelberg).
Learning from one system about others
Universality upens up the possibility to obtain important information on quantum systems that are usually inaccessible in a laboratory. “Nobody can recreate the Big Bang in a laboratory, but if we know the universality class to which it belongs, we can look at other quantum systems in the same class and indirectly investigate universal properties during the Big Bang,” explains Schmiedmayer. “Better understanding the behaviour of many-particle quantum systems that are far from equilibrium is one of the most pressing issues in physics today. Even with the best supercomputers, there’s no chance of precisely calculating these events, and so our universality classes are a major opportunity to learn something new.”
________________________________________________________________________________
Original publication
S. Erne, R. Bücker, T. Gasenzer, J. Berges, and J. Schmiedmayer: Universal dynamics in an isolated onedimensional
Bose gas far from equilibrium, Nature 563, 225-229 (2018) DOI: 10.1038/s41586-018-0667-0
________________________________________________________________________________
Prof. Jörg Schmiedmayer
Vienna Center for Quantum Science and Technology (VCQ)
TU Wien
Stadionallee 2, 1020 Vienna
T: +43-1-58801-141801
hannes-joerg.schmiedmayer@tuwien.ac.at
Dr. Florian Aigner
TU Wien
Public Relations and Marketing
Resselgasse 3, 1040 Wien
T: +43-1-58801-41027
florian.aigner@tuwien.ac.at
Press release of TU Wien, 08 November 2018
When quantum particles swirl about, they still obey universal laws. Different quantum systems can show the same behaviour – this has been demonstrated by two different experiments at TU Wien and Heidelberg University.
Some things are so complicated that it is completely impossible to precisely calculate them. This includes large quantum systems, which consist of many particles, particularly when they are not in an equilibrium state, but changing rapidly. Such examples include the wild particle inferno that occurs in particle accelerators when large atoms collide, or in the early universe, just after the Big Bang, when particles rapidly expanded and subsequently cooled.
At TU Wien and Heidelberg University, remarkable rules have been detected in the apparent chaos of disequilibrium processes. This indicates that such processes can be divided into universality classes. Systems belonging to the same class behave identically in many ways. This means that experiments can be carried out with quantum systems that are easy to handle, in order to obtain precise information about other systems that cannot be directly studied in the experiment. These findings have since been published in the journal ‘Nature’.
Universal rules
“Universality classes are known from other areas of physics,” says Prof. Jörg Schmiedmayer from the Institute of Atomic and Subatomic Physics at TU Wien. “When you study phase transitions, for example materials very close to the melting point, you can describe certain properties using formulas that are very universal, such as the relationship between the specific heat and the temperature.” The microscopic details of the melting process do not matter. Very different materials can obey the same simple equations.
“It is however entirely astounding that universality of this kind can also be found in quantum systems that are far removed from an equilibrium state,” says Jörg Schmiedmayer. “At first glance, you wouldn’t expect this: why should a quantum system made up of many particles that are changing extremely rapidly obey any universal laws?” Nevertheless, theoretical work from Jürgen Berges and Thomas Gasenzer’s groups from Heidelberg University predicted exactly that. These notable predictions have now been verified twice at the same time – at TU Wien and in Heidelberg.
The quick and the slow direction
The experiment in Prof. Schmiedmayer’s group at the Vienna Center for Quantum Science and Technology (VCQ) at the Institute of Atomic and Subatomic Physics (TU Wien) is using a very special atom trap. On an atom chip, thousands of rubidium atoms can be trapped and cooled using electromagnetic fields. “In this process, we generate an atom cloud with a short and a long direction, similar to a cigar,” explains Sebastian Erne, the lead author of the study.
Initially, the atoms move in all directions at the same speed. The atom trap can however be opened in the short (transverse) directions, meaning that those atoms that are moving particularly fast in this direction fly away. This leaves behind only atoms that have a relatively low speed in the transverse directions.
“The speed distribution in one direction is changed so quickly that during this time, the speed distribution in the other direction, along the longer axis of the cigar, virtually does not change at all,” says Sebastian Erne. “As a result, we produce a state that is far from the thermal equilibrium.” Collisions and interactions then lead to energy exchange between the atoms, which is referred to as ‘thermalisation’.
“Our experiment demonstrates that the course of this thermalisation follows a universal law and is not dependent on any details,” says Jörg Schmiedmayer. “Regardless of how we started the thermalisation, the transition can always be described with the same formula.”
It was a similar story for the research team from Heidelberg. There too, they started out with an elongated atom cloud. However, the Heidelberg team did not study the speed but the spin (the intrinsic angular momentum) of the particles. They first controlled the spin directions of the atoms and then observed how these directions change over time due to interactions between the atoms.
This change can be described using the same formulas as the one from the other experiment: “In our case, the physical situation is quite different from that of the TU Wien experiment, but the dynamics also obey universal scaling laws,” explains Maximilian Prüfer (Heidelberg), first author of Heidelberg publication. “We have found a process that also obeys the universality but belongs to a different universality class. This is great because it confirms our theories very convincingly and suggests that we really are on to something – a new, fundamental law, ” says Markus Oberthaler (also Heidelberg).
Learning from one system about others
Universality upens up the possibility to obtain important information on quantum systems that are usually inaccessible in a laboratory. “Nobody can recreate the Big Bang in a laboratory, but if we know the universality class to which it belongs, we can look at other quantum systems in the same class and indirectly investigate universal properties during the Big Bang,” explains Schmiedmayer. “Better understanding the behaviour of many-particle quantum systems that are far from equilibrium is one of the most pressing issues in physics today. Even with the best supercomputers, there’s no chance of precisely calculating these events, and so our universality classes are a major opportunity to learn something new.”
________________________________________________________________________________
Original publication
S. Erne, R. Bücker, T. Gasenzer, J. Berges, and J. Schmiedmayer: Universal dynamics in an isolated onedimensional
Bose gas far from equilibrium, Nature 563, 225-229 (2018) DOI: 10.1038/s41586-018-0667-0
________________________________________________________________________________
Prof. Jörg Schmiedmayer
Vienna Center for Quantum Science and Technology (VCQ)
TU Wien
Stadionallee 2, 1020 Vienna
T: +43-1-58801-141801
hannes-joerg.schmiedmayer@tuwien.ac.at
Dr. Florian Aigner
TU Wien
Public Relations and Marketing
Resselgasse 3, 1040 Wien
T: +43-1-58801-41027
florian.aigner@tuwien.ac.at
Pressemitteilung TU Wien, 08. November 2018
Wenn Quantenteilchen durcheinanderwirbeln, halten sie sich trotzdem an universelle Gesetze. Was für ein Quantensystem gilt, gilt auch für andere – das zeigen zwei verschiedene Experimente an der TU Wien und der Universität Heidelberg.
Manche Dinge sind so kompliziert, dass es völlig unmöglich ist, sie exakt zu berechnen. Dazu gehören große Quantensysteme, die aus vielen Teilchen bestehen – insbesondere dann, wenn sie sich nicht in einem Gleichgewichtszustand befinden, sondern sich rasch verändern. Das trifft zum Beispiel auf das wilde Teilcheninferno zu, das in Teilchenbeschleunigern bei der Kollision großer Atome entsteht, oder auch auf das frühe Universum, kurz nach dem Urknall, das sich rasch ausdehnte und dabei abkühlte.
An der TU Wien und der Universität Heidelberg konnte man nun zeigen, dass sich im scheinbaren Chaos solcher Ungleichgewichtsprozesse doch bemerkenswerte Regeln entdecken lassen. Das weist darauf hin, dass man solche Vorgänge in universelle Klassen einteilen kann. Systeme, die zur selben Klasse gehören, verhalten sich in vielerlei Hinsicht völlig gleich. Das bedeutet, dass man Experimente mit experimentell leichter handhabbaren Quantensystemen durchführen kann, um exakte Information über andere Systeme zu erhalten, die im Experiment nicht direkt studiert werden können. Diese Ergebnisse wurden nun im Fachjournal „Nature“ publiziert.
Universelle Regeln
„Universalitätsklassen kennt man aus anderen Bereichen der Physik“, sagt Prof. Jörg Schmiedmayer vom Atominstitut der TU Wien. „Wenn man Phasenübergänge studiert, zum Beispiel Materialien ganz knapp am Schmelzpunkt, dann kann man bestimmte Eigenschaften mit Formeln beschreiben, die sehr universell sind. Zum Beispiel den Zusammenhang zwischen der spezifischen Wärme mit der Temperatur.“ Für solche Zusammenhänge spielen die mikroskopischen Details des Schmelzvorgangs keine Rolle. Sehr unterschiedliche Materialien können denselben einfachen Gleichungen gehorchen.
„Höchst erstaunlich ist allerdings, dass man eine solche Universalität auch bei Quantensystemen findet, die von einem Gleichgewichtszustand weit entfernt sind“, sagt Jörg Schmiedmayer. „Das würde man auf den ersten Blick nicht erwarten: Warum soll sich ein Quantensystem aus vielen Teilchen, das sich gerade rasend schnell verändert, an irgendwelche universellen Gesetze halten? Trotzdem sagten theoretische Arbeiten aus den Gruppen von Jürgen Berges und Thomas Gasenzer von der Universität Heidelberg genau das voraus. Diese bemerkenswerten Vorhersagen wurden nun zur gleichen Zeit gleich doppelt nachgewiesen – an der TU Wien und in Heidelberg.
Die schnelle und die langsame Richtung
Das Experiment in der Gruppe von Prof. Schmiedmayer am Vienna Center for Quantum Science and Technology (VCQ), Atominstitut, der TU Wien verwendet dafür eine ganz besondere Atom-Falle: Auf einem speziellen Atomchip können tausende Rubidium-Atome mit elektromagnetischen Feldern gefangen und abgekühlt werden. „Dabei erzeugen wir eine Atom-Wolke mit einer kurzen und einer langen Richtung – ähnlich wie eine Zigarre“, erklärt Sebastian Erne, der Erstautor der Studie.
Zunächst bewegen sich die Atome in beiden Richtungen mit gleicher Geschwindigkeit. Man kann allerdings die Atomfalle in der kurzen (transversalen) Richtung so öffnen, sodass jene Atome davonfliegen, die sich besonders schnell in diese Richtung bewegen. Somit bleiben nur noch Atome übrig, die transversal eine relativ geringe Geschwindigkeit aufweisen.
„Die Geschwindigkeitsverteilung entlang der einen Richtung wird so rasch verändert, dass sich in dieser Zeit die Geschwindigkeitsverteilung in der anderen Richtung – entlang der längeren Achse der Zigarre – praktisch nicht verändert“, sagt Sebastian Erne. „Dadurch erzeugen wir einen Zustand fernab des thermischen Gleichgewichts.“ Durch Stöße und Wechselwirkungen kommt es dann zum Austausch von Energie zwischen den Atomen, man spricht von „Thermalisierung“.
„Unser Experiment zeigt, dass der Verlauf dieser Thermalisierung einem ganz universellen Gesetz folgt und nicht von irgendwelchen Details abhängt“, sagt Jörg Schmiedmayer. „Egal, wie wir die Thermalisierung starten: Der Übergang lässt sich immer mit derselben Formel beschreiben.“
Ähnlich ging das Forschungsteam aus Heidelberg vor: Auch dort begann man mit einer in die Länge gezogenen Atomwolke in einem instabilen Anfangszustand. Allerdings untersuchte man nicht die Geschwindigkeit, sondern den Spin (den Eigendrehimpuls) der Teilchen. Zunächst wurden die Spins der Atome zur Anregung gebracht, und man beobachtete dann, wie sich deren Ausrichtung durch Wechselwirkungen zwischen den Atomen mit der Zeit veränderte.
Diese zeitliche Veränderung lässt sich anhand derselben Formeln beschreiben wie die aus dem anderen Experiment: „Die physikalische Situation ist in unserem Fall ganz anders als beim Wiener Experiment, die Dynamik gehorcht aber ebenso universellen Skalierungsgesetzen“, erklärt Maximilian Prüfer (Heidelberg), Erstautor der Heidelberger Publikation. „Wir haben einen Prozess gefunden, der denselben universellen Regeln gehorcht, aber zu einer anderen Universalitätsklasse gehört. Das ist sehr erfreulich, weil es die Theorien noch überzeugender bestätigt und nahelegt, dass wir einem fundamentalen Gesetz auf der Spur sind”, betont Markus Oberthaler (ebenfalls Heidelberg).
Von einem System über andere lernen
Diese Universalität bedeutet, dass man nun eine neue Methode zur Verfügung hat, wichtige Informationen über normalerweise im Labor nicht unzugängliche Quantensysteme zu erhalten. „Niemand kann den Urknall im Labor nachbauen. Aber wenn wir wissen, zu welcher Universalitätsklasse er gehört, können wir uns andere Quantensysteme derselben Klasse ansehen, und so indirekt untersuchen, was beim Urknall passiert ist“, erklärt Schmiedmayer. „Das Verhalten von Vielteilchen-Quantensystemen weit entfernt vom Gleichgewicht besser zu verstehen, gilt heute als eine der drängendsten Fragen in der Physik. Selbst mit den besten Supercomputern hat man keine Chance, diese Vorgänge exakt zu berechnen, daher sind unsere Universalitätsklassen eine wichtige Möglichkeit, Neues zu lernen.“
________________________________________________________________________________
Originalpublikation
S. Erne, R. Bücker, T. Gasenzer, J. Berges, and J. Schmiedmayer: Universal dynamics in an isolated onedimensional
Bose gas far from equilibrium, Nature 563, 225-229 (2018) DOI: 10.1038/s41586-018-0667-0
________________________________________________________________________________
Prof. Jörg Schmiedmayer
Atominstitut, Vienna Center for Quantum Science and Technology (VCQ)
Technische Universität Wien
Stadionallee 2, 1020 Wien
T: +43-1-58801-141801
hannes-joerg.schmiedmayer@tuwien.ac.at
Dr. Florian Aigner
Technische Universität Wien
PR und Marketing
Resselgasse 3, 1040 Wien
T: +43-1-58801-41027
florian.aigner@tuwien.ac.at
Pressemitteilung TU Wien, 08. November 2018
Wenn Quantenteilchen durcheinanderwirbeln, halten sie sich trotzdem an universelle Gesetze. Was für ein Quantensystem gilt, gilt auch für andere – das zeigen zwei verschiedene Experimente an der TU Wien und der Universität Heidelberg.
Manche Dinge sind so kompliziert, dass es völlig unmöglich ist, sie exakt zu berechnen. Dazu gehören große Quantensysteme, die aus vielen Teilchen bestehen – insbesondere dann, wenn sie sich nicht in einem Gleichgewichtszustand befinden, sondern sich rasch verändern. Das trifft zum Beispiel auf das wilde Teilcheninferno zu, das in Teilchenbeschleunigern bei der Kollision großer Atome entsteht, oder auch auf das frühe Universum, kurz nach dem Urknall, das sich rasch ausdehnte und dabei abkühlte.
An der TU Wien und der Universität Heidelberg konnte man nun zeigen, dass sich im scheinbaren Chaos solcher Ungleichgewichtsprozesse doch bemerkenswerte Regeln entdecken lassen. Das weist darauf hin, dass man solche Vorgänge in universelle Klassen einteilen kann. Systeme, die zur selben Klasse gehören, verhalten sich in vielerlei Hinsicht völlig gleich. Das bedeutet, dass man Experimente mit experimentell leichter handhabbaren Quantensystemen durchführen kann, um exakte Information über andere Systeme zu erhalten, die im Experiment nicht direkt studiert werden können. Diese Ergebnisse wurden nun im Fachjournal „Nature“ publiziert.
Universelle Regeln
„Universalitätsklassen kennt man aus anderen Bereichen der Physik“, sagt Prof. Jörg Schmiedmayer vom Atominstitut der TU Wien. „Wenn man Phasenübergänge studiert, zum Beispiel Materialien ganz knapp am Schmelzpunkt, dann kann man bestimmte Eigenschaften mit Formeln beschreiben, die sehr universell sind. Zum Beispiel den Zusammenhang zwischen der spezifischen Wärme mit der Temperatur.“ Für solche Zusammenhänge spielen die mikroskopischen Details des Schmelzvorgangs keine Rolle. Sehr unterschiedliche Materialien können denselben einfachen Gleichungen gehorchen.
„Höchst erstaunlich ist allerdings, dass man eine solche Universalität auch bei Quantensystemen findet, die von einem Gleichgewichtszustand weit entfernt sind“, sagt Jörg Schmiedmayer. „Das würde man auf den ersten Blick nicht erwarten: Warum soll sich ein Quantensystem aus vielen Teilchen, das sich gerade rasend schnell verändert, an irgendwelche universellen Gesetze halten? Trotzdem sagten theoretische Arbeiten aus den Gruppen von Jürgen Berges und Thomas Gasenzer von der Universität Heidelberg genau das voraus. Diese bemerkenswerten Vorhersagen wurden nun zur gleichen Zeit gleich doppelt nachgewiesen – an der TU Wien und in Heidelberg.
Die schnelle und die langsame Richtung
Das Experiment in der Gruppe von Prof. Schmiedmayer am Vienna Center for Quantum Science and Technology (VCQ), Atominstitut, der TU Wien verwendet dafür eine ganz besondere Atom-Falle: Auf einem speziellen Atomchip können tausende Rubidium-Atome mit elektromagnetischen Feldern gefangen und abgekühlt werden. „Dabei erzeugen wir eine Atom-Wolke mit einer kurzen und einer langen Richtung – ähnlich wie eine Zigarre“, erklärt Sebastian Erne, der Erstautor der Studie.
Zunächst bewegen sich die Atome in beiden Richtungen mit gleicher Geschwindigkeit. Man kann allerdings die Atomfalle in der kurzen (transversalen) Richtung so öffnen, sodass jene Atome davonfliegen, die sich besonders schnell in diese Richtung bewegen. Somit bleiben nur noch Atome übrig, die transversal eine relativ geringe Geschwindigkeit aufweisen.
„Die Geschwindigkeitsverteilung entlang der einen Richtung wird so rasch verändert, dass sich in dieser Zeit die Geschwindigkeitsverteilung in der anderen Richtung – entlang der längeren Achse der Zigarre – praktisch nicht verändert“, sagt Sebastian Erne. „Dadurch erzeugen wir einen Zustand fernab des thermischen Gleichgewichts.“ Durch Stöße und Wechselwirkungen kommt es dann zum Austausch von Energie zwischen den Atomen, man spricht von „Thermalisierung“.
„Unser Experiment zeigt, dass der Verlauf dieser Thermalisierung einem ganz universellen Gesetz folgt und nicht von irgendwelchen Details abhängt“, sagt Jörg Schmiedmayer. „Egal, wie wir die Thermalisierung starten: Der Übergang lässt sich immer mit derselben Formel beschreiben.“
Ähnlich ging das Forschungsteam aus Heidelberg vor: Auch dort begann man mit einer in die Länge gezogenen Atomwolke in einem instabilen Anfangszustand. Allerdings untersuchte man nicht die Geschwindigkeit, sondern den Spin (den Eigendrehimpuls) der Teilchen. Zunächst wurden die Spins der Atome zur Anregung gebracht, und man beobachtete dann, wie sich deren Ausrichtung durch Wechselwirkungen zwischen den Atomen mit der Zeit veränderte.
Diese zeitliche Veränderung lässt sich anhand derselben Formeln beschreiben wie die aus dem anderen Experiment: „Die physikalische Situation ist in unserem Fall ganz anders als beim Wiener Experiment, die Dynamik gehorcht aber ebenso universellen Skalierungsgesetzen“, erklärt Maximilian Prüfer (Heidelberg), Erstautor der Heidelberger Publikation. „Wir haben einen Prozess gefunden, der denselben universellen Regeln gehorcht, aber zu einer anderen Universalitätsklasse gehört. Das ist sehr erfreulich, weil es die Theorien noch überzeugender bestätigt und nahelegt, dass wir einem fundamentalen Gesetz auf der Spur sind”, betont Markus Oberthaler (ebenfalls Heidelberg).
Von einem System über andere lernen
Diese Universalität bedeutet, dass man nun eine neue Methode zur Verfügung hat, wichtige Informationen über normalerweise im Labor nicht unzugängliche Quantensysteme zu erhalten. „Niemand kann den Urknall im Labor nachbauen. Aber wenn wir wissen, zu welcher Universalitätsklasse er gehört, können wir uns andere Quantensysteme derselben Klasse ansehen, und so indirekt untersuchen, was beim Urknall passiert ist“, erklärt Schmiedmayer. „Das Verhalten von Vielteilchen-Quantensystemen weit entfernt vom Gleichgewicht besser zu verstehen, gilt heute als eine der drängendsten Fragen in der Physik. Selbst mit den besten Supercomputern hat man keine Chance, diese Vorgänge exakt zu berechnen, daher sind unsere Universalitätsklassen eine wichtige Möglichkeit, Neues zu lernen.“
________________________________________________________________________________
Originalpublikation
S. Erne, R. Bücker, T. Gasenzer, J. Berges, and J. Schmiedmayer: Universal dynamics in an isolated onedimensional
Bose gas far from equilibrium, Nature 563, 225-229 (2018) DOI: 10.1038/s41586-018-0667-0
________________________________________________________________________________
Prof. Jörg Schmiedmayer
Atominstitut, Vienna Center for Quantum Science and Technology (VCQ)
Technische Universität Wien
Stadionallee 2, 1020 Wien
T: +43-1-58801-141801
hannes-joerg.schmiedmayer@tuwien.ac.at
Dr. Florian Aigner
Technische Universität Wien
PR und Marketing
Resselgasse 3, 1040 Wien
T: +43-1-58801-41027
florian.aigner@tuwien.ac.at
joint press release of Universität Heidelberg, WWU Münster and GSI Darmstadt,
20 September 2018
New findings published in ”Nature“ on the formation of matter / Experiments provide information on the beginnings of the universe
The building blocks of matter in our universe were formed in the first 10 microseconds of its existence, according to the currently accepted scientific picture. After the Big Bang about 13.7 billion years ago, matter consisted mainly of quarks and gluons, two types of elementary particles whose interactions are governed by quantum chromodynamics (QCD), the theory of strong interaction. In the early universe, these particles moved (nearly) freely in a quark-gluon plasma. Then, in a phase transition, they combined and formed hadrons, among them the building blocks of atomic nuclei, protons and neutrons. In the current issue of the science journal “Nature”, an international team of scientists presents an analysis of a series of experiments at major particle accelerators which sheds light on the nature of this transition. The scientists determined with precision the transition temperature and obtained new insights into the mechanism of cooling and freeze-out of the quark-gluon plasma into the current constituents of matter such as protons, neutrons, and atomic nuclei. The team of researchers consists of scientists from the GSI Helmholtzzentrum für Schwerionenforschung in Darmstadt, and from the universities of Heidelberg, Münster, and Wroclaw (Poland).
Analysis of experimental results confirm the predicted value of the transition temperature / Onehundred and twenty thousend times hotter than the interior of the sun
A central result: The experiments at world-wide highest energy with the ALICE detector at the Large Hadron Collider (LHC) at the research center CERN produce matter where particles and anti-particles coexist, with very high accuracy, in equal amounts, similar to the conditions in the early universe. The team confirms, with analysis of the experimental data, theoretical predictions that the phase transition between quark-gluon plasma and hadronic matter takes place at the temperature of 156 MeV. This temperature is 120,000 times higher than that in the interior of the sun.
“Snowballs in hell”
The physicists analyzed more precisely the yields of a number of particles and anti-particles. “Our investigations revealed a number of surprizing discoveries. One of them is that light nuclei and their anti-particles are produced at the same temperature as protons and anti-protons, although their binding energies are about 100 times smaller than the energy corresponding to the transition temperature”, explains Prof. Dr. Anton Andronic who recently joined the University of Münster from the GSI Helmholtzzentrum für Schwerionenforschung. The scientists presume that such “loosely bound objects” are formed at high temperature first as compact multi-quark objects which only later develop into the observed light nuclei and anti-nuclei. The existence of such multi-quark states was proposed a long time ago but no convincing evidence was found.
“Confinement”: Charm quarks travel freely in the fireball
Another remarkable observation concerns a phenomenon long known but poorly understood: Normally, quarks are confined into the interior of protons and neutrons; isolated quarks have never been observed, a property which scientists describe as “confinement”. In the interior of the fireball formed in nuclear collisions at high energy this confinement is lifted (deconfinement). The new study shows that charmonium states such as J/psi mesons, consisting of a pair of charm and anti-charm quarks, are produced far more often at LHC energies compared to observations at lower energies, such as at the “Relativistic Heavy Ion Collider” in the USA. Because of the higher energy density at LHC the opposite, namely a reduction of J/psi mesons through dissociation was expected. In contradistinction, enhancement was predicted 18 years ago by two of the team members (Prof. Dr. Peter Braun-Munzinger, GSI, and Prof. Dr. Johanna Stachel, Universität Heidelberg) because of deconfinement of the charm quarks. The consequences of the prediction were worked out in detail in a series of publications by the whole team. The now observed enhanced production of J/psi particles confirms the prediction: J/psi mesons can only be produced in the observed large quantities if their constituents, the charm- and anticharm quarks, can travel freely in the fireball over distances of a trillionth of a centimeter – corresponding to about ten times the size of a proton. “These observations are a first step towards understanding the phenomenon of confinement in more detail”, underlines Prof. Dr. Krzysztof Redlich of the University of Wroclaw (Poland).
Experiments at CERN and at Brookhaven National Laboratory
The data were obtained during several years of investigations in the framework of the experiment “ALICE” at the Large Hadron Collider accelerator at the research center CERN near Geneva. In “ALICE”, scientists from 41 countries investigated in collisions between two lead nuclei the state of the universe within microseconds after the Big Bang. The highest ever man-made energy densities are produced in such collisions. These result in the formation of matter (quarks and gluons) as it existed at that time in the early universe. In each head-on collision more than 30,000 particles (hadrons) are produced which are then detected in the ALICE experiment. The actual study also used data from experiments at lower energy accelerators, the “Super Proton Synchrotron” at CERN and the “Relativistic Heavy Ion Collider” at the US-Brookhaven National Laboratory on Long Island, New York.
The investigations were supported in the framework of the “Collaborative Research Center” 1225 “Isolated quantum systems and universality under extreme conditions (ISOQUANT)” by the German Research Foundation (DFG). Furthermore, they were supported by the Polish National Science Center (NCN) (Maestro grant DEC-2013/10/A/ST2/00106).
Nuclear physics at the University of Münster
Nuclear physicists at the University of Münster not only participated in the ALICE experiment from the start but were also part of the predecessor experiments at CERN and the Relativistic Heavy Ion Collider. The group at the Institute for Nuclear Physics, first under the direction of Prof. Dr. Rainer Santo, then Prof. Dr. Johannes Wessels and now Prof. Dr. Anton Andronic, played a leading role in the design, construction and operation of the ALICE Transition Radiation Detector TRD which is primarily used to identify electrons and positrons. In addition, the Münster group played a significant role in the physics analyses for the ALICE experiment.
________________________________________________________________________________
Original publication:
A. Andronic, P. Braun-Munzinger, K. Redlich und J. Stachel: Decoding the phase structure of QCD via particle
production at high energy, Nature 561, 321–330 (2018)
DOI:10.1038/s41586-018-0491-6
________________________________________________________________________________
Communication and Public Relations
Schlossplatz 2
48149 Münster
Tel: +49 251 83-22232
Fax: +49 251 83-22258
joint press release of Universität Heidelberg, WWU Münster and GSI Darmstadt,
20 September 2018
New findings published in ”Nature“ on the formation of matter / Experiments provide information on the beginnings of the universe
The building blocks of matter in our universe were formed in the first 10 microseconds of its existence, according to the currently accepted scientific picture. After the Big Bang about 13.7 billion years ago, matter consisted mainly of quarks and gluons, two types of elementary particles whose interactions are governed by quantum chromodynamics (QCD), the theory of strong interaction. In the early universe, these particles moved (nearly) freely in a quark-gluon plasma. Then, in a phase transition, they combined and formed hadrons, among them the building blocks of atomic nuclei, protons and neutrons. In the current issue of the science journal “Nature”, an international team of scientists presents an analysis of a series of experiments at major particle accelerators which sheds light on the nature of this transition. The scientists determined with precision the transition temperature and obtained new insights into the mechanism of cooling and freeze-out of the quark-gluon plasma into the current constituents of matter such as protons, neutrons, and atomic nuclei. The team of researchers consists of scientists from the GSI Helmholtzzentrum für Schwerionenforschung in Darmstadt, and from the universities of Heidelberg, Münster, and Wroclaw (Poland).
Analysis of experimental results confirm the predicted value of the transition temperature / Onehundred and twenty thousend times hotter than the interior of the sun
A central result: The experiments at world-wide highest energy with the ALICE detector at the Large Hadron Collider (LHC) at the research center CERN produce matter where particles and anti-particles coexist, with very high accuracy, in equal amounts, similar to the conditions in the early universe. The team confirms, with analysis of the experimental data, theoretical predictions that the phase transition between quark-gluon plasma and hadronic matter takes place at the temperature of 156 MeV. This temperature is 120,000 times higher than that in the interior of the sun.
“Snowballs in hell”
The physicists analyzed more precisely the yields of a number of particles and anti-particles. “Our investigations revealed a number of surprizing discoveries. One of them is that light nuclei and their anti-particles are produced at the same temperature as protons and anti-protons, although their binding energies are about 100 times smaller than the energy corresponding to the transition temperature”, explains Prof. Dr. Anton Andronic who recently joined the University of Münster from the GSI Helmholtzzentrum für Schwerionenforschung. The scientists presume that such “loosely bound objects” are formed at high temperature first as compact multi-quark objects which only later develop into the observed light nuclei and anti-nuclei. The existence of such multi-quark states was proposed a long time ago but no convincing evidence was found.
“Confinement”: Charm quarks travel freely in the fireball
Another remarkable observation concerns a phenomenon long known but poorly understood: Normally, quarks are confined into the interior of protons and neutrons; isolated quarks have never been observed, a property which scientists describe as “confinement”. In the interior of the fireball formed in nuclear collisions at high energy this confinement is lifted (deconfinement). The new study shows that charmonium states such as J/psi mesons, consisting of a pair of charm and anti-charm quarks, are produced far more often at LHC energies compared to observations at lower energies, such as at the “Relativistic Heavy Ion Collider” in the USA. Because of the higher energy density at LHC the opposite, namely a reduction of J/psi mesons through dissociation was expected. In contradistinction, enhancement was predicted 18 years ago by two of the team members (Prof. Dr. Peter Braun-Munzinger, GSI, and Prof. Dr. Johanna Stachel, Universität Heidelberg) because of deconfinement of the charm quarks. The consequences of the prediction were worked out in detail in a series of publications by the whole team. The now observed enhanced production of J/psi particles confirms the prediction: J/psi mesons can only be produced in the observed large quantities if their constituents, the charm- and anticharm quarks, can travel freely in the fireball over distances of a trillionth of a centimeter – corresponding to about ten times the size of a proton. “These observations are a first step towards understanding the phenomenon of confinement in more detail”, underlines Prof. Dr. Krzysztof Redlich of the University of Wroclaw (Poland).
Experiments at CERN and at Brookhaven National Laboratory
The data were obtained during several years of investigations in the framework of the experiment “ALICE” at the Large Hadron Collider accelerator at the research center CERN near Geneva. In “ALICE”, scientists from 41 countries investigated in collisions between two lead nuclei the state of the universe within microseconds after the Big Bang. The highest ever man-made energy densities are produced in such collisions. These result in the formation of matter (quarks and gluons) as it existed at that time in the early universe. In each head-on collision more than 30,000 particles (hadrons) are produced which are then detected in the ALICE experiment. The actual study also used data from experiments at lower energy accelerators, the “Super Proton Synchrotron” at CERN and the “Relativistic Heavy Ion Collider” at the US-Brookhaven National Laboratory on Long Island, New York.
The investigations were supported in the framework of the “Collaborative Research Center” 1225 “Isolated quantum systems and universality under extreme conditions (ISOQUANT)” by the German Research Foundation (DFG). Furthermore, they were supported by the Polish National Science Center (NCN) (Maestro grant DEC-2013/10/A/ST2/00106).
Nuclear physics at the University of Münster
Nuclear physicists at the University of Münster not only participated in the ALICE experiment from the start but were also part of the predecessor experiments at CERN and the Relativistic Heavy Ion Collider. The group at the Institute for Nuclear Physics, first under the direction of Prof. Dr. Rainer Santo, then Prof. Dr. Johannes Wessels and now Prof. Dr. Anton Andronic, played a leading role in the design, construction and operation of the ALICE Transition Radiation Detector TRD which is primarily used to identify electrons and positrons. In addition, the Münster group played a significant role in the physics analyses for the ALICE experiment.
________________________________________________________________________________
Original publication:
A. Andronic, P. Braun-Munzinger, K. Redlich und J. Stachel: Decoding the phase structure of QCD via particle
production at high energy, Nature 561, 321–330 (2018)
DOI:10.1038/s41586-018-0491-6
________________________________________________________________________________
Communication and Public Relations
Schlossplatz 2
48149 Münster
Tel: +49 251 83-22232
Fax: +49 251 83-22258
gemeinsame Pressemitteilung der Universität Heidelberg, WWU Münster und GSI Darmstadt,
20. September 2018
Messungen bestätigen vorhergesagte Übergangstemperatur / Einhundertzwanzigtausend Mal heißer als das Innere der Sonne
Ein zentraler Befund: Die Experimente bei weltweit höchster Energie mit dem ALICE-Detektor am Kernforschungszentrum CERN (Large Hadron Collider, LHC) produzieren Materie, in der Teilchen und Antiteilchen mit sehr genau gleicher Häufigkeit vorkommen, identisch mit der Urknallmaterie. Das Team bestätigt durch die Analyse der in den Experimenten gewonnenen Daten theoretische Vorhersagen, nach denen der Phasenübergang zwischen Quark-Gluon-Plasma und hadronischer Materie bei einer Temperatur von 156 Megaelektronenvolt geschieht. Das entspricht einer Temperatur, die Einhundertzwanzigtausend Mal heißer ist als das Innere der Sonne.
Teilchen trotzen hohen Temperaturen im Verbund
Die Physiker analysierten eine Vielzahl an Teilchen und Antiteilchen genauer. „Unsere Untersuchungen bringen mehrere überraschende Erkenntnisse mit sich. Eine davon ist, dass leichte Atomkerne und ihre Antiteilchen bei der gleichen Temperatur wie Protonen und Antiprotonen erzeugt werden, obwohl ihre Bindungsenergien etwa einhundert Mal kleiner sind als die der Übergangstemperatur entsprechende Energie“, sagt Prof. Dr. Anton Andronic von der Westfälischen Wilhelms-Universität Münster, vormals GSI Helmholtzzentrum für Schwerionenforschung. Die Wissenschaftler vermuten, dass solche „schwach gebundenen“ Teilchen den hohen Temperaturen zunächst als kompakte Multi-Quark Zustände trotzen, die sich erst bei viel niedrigerer Temperatur in die beobachteten leichten Atomkerne oder Antikerne entwickeln. Die Existenz solcher Multi-Quark Zustände wurde seit langer Zeit vermutet, ohne dass konkrete Hinweise darauf gefunden werden konnten.
„Confinement“: Charm-Quarks wandern im Feuerball
Eine andere besonders interessante Beobachtung betrifft ein zwar seit langer Zeit bekanntes, aber bisher nicht verstandenes Phänomen: Normalerweise sind Quarks im Innern von Protonen und Neutronen eingeschlossen; isolierte Quarks kommen nicht vor – eine Eigenschaft, die fachsprachlich mit „confinement“ bezeichnet wird. Im Inneren des Feuerballs, der nach einer Kernkollision im Teilchenbeschleuniger entsteht, ist dieses „confinement“ aufgehoben („deconfinement“). Die neue Studie zeigt: Charmonium-Zustände – sogenannte J/psi-Mesonen, bestehend aus einem schweren Charm-Quark und seinem Antiteilchen –, werden bei LHC-Energie weit häufiger erzeugt als bei niedrigerer Energie, zum Beispiel am „Relativistic Heavy Ion Collider“ in den USA. Wegen der höheren Energiedichte am LHC war das Gegenteil, nämlich eine Reduktion durch Dissoziation von J/psi-Mesonen, erwartet worden. Andererseits war vor 18 Jahren von zwei Team-Mitgliedern (Prof. Dr. Peter Braun-Munzinger, GSI, und Prof. Dr. Johanna Stachel, Universität Heidelberg) eine erhöhte Produktion vorhergesagt worden aufgrund des „deconfinements“ der Charm-Quarks. Dieser Vorschlag wurde in einer Serie von Veröffentlichungen vom gesamten Team detailliert ausgearbeitet. Die nun beobachtete verstärkte Produktion der J/psi-Teilchen bestätigt die Vorhersage: J/psi-Mesonen werden nur dann in der beobachteten großen Anzahl produziert, wenn ihre Bestandteile, die Charm- und Anticharm-Quarks, sich in diesem Zustand bis zu einem Billiardstel Zentimeter weit frei bewegen können – das entspricht ungefähr zehn Mal der Größe eines Protons. „Diese Beobachtungen sind ein erster Schritt, um das Phänomen des ‚confinements‘ im Detail zu verstehen“, unterstreicht Prof. Dr. Krzysztof Redlich von der Universität Breslau.
Experimente am Kernforschungszentrum CERN und am Brookhaven National Laboratory
Die Daten stammen aus mehrjährigen Untersuchungen im Rahmen des Experiments „ALICE“ am Teilchenbeschleuniger „Large Hadron Collider“ (LHC) des Kernforschungszentrums CERN bei Genf. Mit „ALICE“ untersuchen Wissenschaftler aus 41 Ländern anhand von aufeinanderprallenden Strahlen aus Bleiatomkernen den Zustand des Universums unmittelbar nach dem Urknall: Bei den Kollisionen dieser Atomkerne entstehen die höchsten jemals durch Menschen erzeugten Energiedichten. Als Folge dieser Zusammenstöße bildet sich Materie (Quarks), wie sie unmittelbar zu Beginn des Universums existierte. Pro Kollision entstehen mehr als 30.000 Teilchen, die mit den „ALICE“-Detektoren vermessen werden. In die aktuelle Studie flossen außerdem Daten aus Experimenten an zwei Teilchenbeschleunigern mit niedrigerer Energie ein: dem „Super Proton Synchroton“ am CERN sowie dem „Relativistic Heavy Ion Collider“ am US-amerikanischen Brookhaven National Laboratory auf Long Island, New York.
Die Arbeiten wurden im Rahmen des Sonderforschungsbereichs (SFB) 1225 „Isolierte Quantensysteme und Universalität unter extremen Bedingungen (ISOQUANT)“ von der Deutschen Forschungsgemeinschaft unterstützt. Sie wurden außerdem durch das polnische National Science Center (NCN) gefördert (Maestro grant DEC-2013/10/A/ST2/00106).
Kernphysik an der Westfälischen Wilhelms-Universität Münster (WWU)
Die Kernphysiker der WWU sind nicht nur von Beginn an Teil des ALICE-Teams, sondern sie waren auch bereits an den Vorgänger-Experimenten am CERN sowie an Experimenten am Brookhaven National Laboratory beteiligt. Die Gruppe am Institut für Kernphysik – zunächst unter der Leitung von Prof. Dr. Rainer Santo, danach Prof. Dr. Johannes Wessels und nun Prof. Dr. Anton Andronic – war beispielsweise federführend an der Konzeption, am Aufbau und am Betrieb des sogenannten Übergangsstrahlungsdetektors beteiligt, der im ALICE-Experiment Elektronen und Positronen nachweist. Außerdem spielen die Münsteraner eine führende Rolle bei vielen physikalischen Analysen des ALICE-Experiments.
________________________________________________________________________________
Originalpublikation:
A. Andronic, P. Braun-Munzinger, K. Redlich und J. Stachel: Decoding the phase structure of QCD via particle
production at high energy, Nature 561, 321–330 (2018)
DOI:10.1038/s41586-018-0491-6
________________________________________________________________________________
Kommunikation und Öffentlichkeitsarbeit
Schlossplatz 2
48149 Münster
Tel: +49 251 83-22232
Fax: +49 251 83-22258
gemeinsame Pressemitteilung der Universität Heidelberg, WWU Münster und GSI Darmstadt,
20. September 2018
Messungen bestätigen vorhergesagte Übergangstemperatur / Einhundertzwanzigtausend Mal heißer als das Innere der Sonne
Ein zentraler Befund: Die Experimente bei weltweit höchster Energie mit dem ALICE-Detektor am Kernforschungszentrum CERN (Large Hadron Collider, LHC) produzieren Materie, in der Teilchen und Antiteilchen mit sehr genau gleicher Häufigkeit vorkommen, identisch mit der Urknallmaterie. Das Team bestätigt durch die Analyse der in den Experimenten gewonnenen Daten theoretische Vorhersagen, nach denen der Phasenübergang zwischen Quark-Gluon-Plasma und hadronischer Materie bei einer Temperatur von 156 Megaelektronenvolt geschieht. Das entspricht einer Temperatur, die Einhundertzwanzigtausend Mal heißer ist als das Innere der Sonne.
Teilchen trotzen hohen Temperaturen im Verbund
Die Physiker analysierten eine Vielzahl an Teilchen und Antiteilchen genauer. „Unsere Untersuchungen bringen mehrere überraschende Erkenntnisse mit sich. Eine davon ist, dass leichte Atomkerne und ihre Antiteilchen bei der gleichen Temperatur wie Protonen und Antiprotonen erzeugt werden, obwohl ihre Bindungsenergien etwa einhundert Mal kleiner sind als die der Übergangstemperatur entsprechende Energie“, sagt Prof. Dr. Anton Andronic von der Westfälischen Wilhelms-Universität Münster, vormals GSI Helmholtzzentrum für Schwerionenforschung. Die Wissenschaftler vermuten, dass solche „schwach gebundenen“ Teilchen den hohen Temperaturen zunächst als kompakte Multi-Quark Zustände trotzen, die sich erst bei viel niedrigerer Temperatur in die beobachteten leichten Atomkerne oder Antikerne entwickeln. Die Existenz solcher Multi-Quark Zustände wurde seit langer Zeit vermutet, ohne dass konkrete Hinweise darauf gefunden werden konnten.
„Confinement“: Charm-Quarks wandern im Feuerball
Eine andere besonders interessante Beobachtung betrifft ein zwar seit langer Zeit bekanntes, aber bisher nicht verstandenes Phänomen: Normalerweise sind Quarks im Innern von Protonen und Neutronen eingeschlossen; isolierte Quarks kommen nicht vor – eine Eigenschaft, die fachsprachlich mit „confinement“ bezeichnet wird. Im Inneren des Feuerballs, der nach einer Kernkollision im Teilchenbeschleuniger entsteht, ist dieses „confinement“ aufgehoben („deconfinement“). Die neue Studie zeigt: Charmonium-Zustände – sogenannte J/psi-Mesonen, bestehend aus einem schweren Charm-Quark und seinem Antiteilchen –, werden bei LHC-Energie weit häufiger erzeugt als bei niedrigerer Energie, zum Beispiel am „Relativistic Heavy Ion Collider“ in den USA. Wegen der höheren Energiedichte am LHC war das Gegenteil, nämlich eine Reduktion durch Dissoziation von J/psi-Mesonen, erwartet worden. Andererseits war vor 18 Jahren von zwei Team-Mitgliedern (Prof. Dr. Peter Braun-Munzinger, GSI, und Prof. Dr. Johanna Stachel, Universität Heidelberg) eine erhöhte Produktion vorhergesagt worden aufgrund des „deconfinements“ der Charm-Quarks. Dieser Vorschlag wurde in einer Serie von Veröffentlichungen vom gesamten Team detailliert ausgearbeitet. Die nun beobachtete verstärkte Produktion der J/psi-Teilchen bestätigt die Vorhersage: J/psi-Mesonen werden nur dann in der beobachteten großen Anzahl produziert, wenn ihre Bestandteile, die Charm- und Anticharm-Quarks, sich in diesem Zustand bis zu einem Billiardstel Zentimeter weit frei bewegen können – das entspricht ungefähr zehn Mal der Größe eines Protons. „Diese Beobachtungen sind ein erster Schritt, um das Phänomen des ‚confinements‘ im Detail zu verstehen“, unterstreicht Prof. Dr. Krzysztof Redlich von der Universität Breslau.
Experimente am Kernforschungszentrum CERN und am Brookhaven National Laboratory
Die Daten stammen aus mehrjährigen Untersuchungen im Rahmen des Experiments „ALICE“ am Teilchenbeschleuniger „Large Hadron Collider“ (LHC) des Kernforschungszentrums CERN bei Genf. Mit „ALICE“ untersuchen Wissenschaftler aus 41 Ländern anhand von aufeinanderprallenden Strahlen aus Bleiatomkernen den Zustand des Universums unmittelbar nach dem Urknall: Bei den Kollisionen dieser Atomkerne entstehen die höchsten jemals durch Menschen erzeugten Energiedichten. Als Folge dieser Zusammenstöße bildet sich Materie (Quarks), wie sie unmittelbar zu Beginn des Universums existierte. Pro Kollision entstehen mehr als 30.000 Teilchen, die mit den „ALICE“-Detektoren vermessen werden. In die aktuelle Studie flossen außerdem Daten aus Experimenten an zwei Teilchenbeschleunigern mit niedrigerer Energie ein: dem „Super Proton Synchroton“ am CERN sowie dem „Relativistic Heavy Ion Collider“ am US-amerikanischen Brookhaven National Laboratory auf Long Island, New York.
Die Arbeiten wurden im Rahmen des Sonderforschungsbereichs (SFB) 1225 „Isolierte Quantensysteme und Universalität unter extremen Bedingungen (ISOQUANT)“ von der Deutschen Forschungsgemeinschaft unterstützt. Sie wurden außerdem durch das polnische National Science Center (NCN) gefördert (Maestro grant DEC-2013/10/A/ST2/00106).
Kernphysik an der Westfälischen Wilhelms-Universität Münster (WWU)
Die Kernphysiker der WWU sind nicht nur von Beginn an Teil des ALICE-Teams, sondern sie waren auch bereits an den Vorgänger-Experimenten am CERN sowie an Experimenten am Brookhaven National Laboratory beteiligt. Die Gruppe am Institut für Kernphysik – zunächst unter der Leitung von Prof. Dr. Rainer Santo, danach Prof. Dr. Johannes Wessels und nun Prof. Dr. Anton Andronic – war beispielsweise federführend an der Konzeption, am Aufbau und am Betrieb des sogenannten Übergangsstrahlungsdetektors beteiligt, der im ALICE-Experiment Elektronen und Positronen nachweist. Außerdem spielen die Münsteraner eine führende Rolle bei vielen physikalischen Analysen des ALICE-Experiments.
________________________________________________________________________________
Originalpublikation:
A. Andronic, P. Braun-Munzinger, K. Redlich und J. Stachel: Decoding the phase structure of QCD via particle
production at high energy, Nature 561, 321–330 (2018)
DOI:10.1038/s41586-018-0491-6
________________________________________________________________________________
Kommunikation und Öffentlichkeitsarbeit
Schlossplatz 2
48149 Münster
Tel: +49 251 83-22232
Fax: +49 251 83-22258
Pressemitteilung Nr. 113/2018, 20. September 2018
Etwa zehn Millionstel Sekunden dauerte es, bis die Bausteine der Materie unserer heutigen Alltagswelt entstanden, so die gängige Annahme von Experten: Nach dem Urknall vor 13,7 Milliarden Jahren bewegten sich Quarks und Gluonen, zwei Arten von Elementarteilchen, in den allerersten Augenblicken des Universums frei in einem Quark-Gluon-Plasma. Dann, in einem Phasenübergang, vereinigten sich diese Teilchen und bildeten Hadronen, darunter die Bausteine von Atomkernen – Protonen und Neutronen. Ein internationales Forscherteam präsentiert nun eine Analyse der Ergebnisse langjähriger Experimente an Teilchenbeschleunigern, die Licht auf die Natur dieses Phasenübergangs wirft.
Sie wurden unter anderem mit dem ALICE-Detektor am Large Hadron Collider des Europäischen Kernforschungszentrums CERN bei den weltweit höchsten Energien durchgeführt. Unter anderem bestimmten die Forscher mit Präzision die Übergangstemperatur und erhielten neue Erkenntnisse zu dem Mechanismus, wie ein Quark-Gluon-Plasma beim Abkühlen in die normalen Materiebausteine wie Protonen, Neutronen oder Atomkerne ausfriert. Das Forscherteam besteht aus Wissenschaftlern des GSI Helmholtzzentrums für Schwerionenforschung in Darmstadt sowie der Universitäten Heidelberg, Münster und Breslau (Polen). Ihre Ergebnisse veröffentlichten sie in „Nature“.
Die Heidelberger Forschungsgruppe am Physikalischen Institut der Universität Heidelberg unter Leitung von Prof. Dr. Johanna Stachel ist seit 1996 Mitglied von ALICE und hat sowohl im Aufbau des Experiments als auch im Betrieb und in der Datenanalyse eine Schlüsselfunktion inne. Prof. Stachel ist Leiterin einer der Hauptkomponenten von ALICE, des TRD-Projekts (Übergangsstrahlungsdetektor), und war stellvertretende Leiterin des TPC-Projekts (Spurendriftkammer). Mit ihrer Gruppe hat sie entscheidenden Anteil am erfolgreichen ALICE-Experiment. In Deutschland ist die Förderung für die LHC-Experimente in vier Forschungsschwerpunkten (FSP) des Bundesministeriums für Bildung und Forschung strukturiert. Die Wissenschaftlerin ist seit Beginn der FSPs Sprecherin des FSP202-ALICE. Die phänomenologischen Untersuchungen zur Interpretation der ALICE-Daten, die im Zentrum der aktuellen „Nature“-Veröffentlichung stehen, wurden im Rahmen des Sonderforschungsbereichs „Isolierte Quantensysteme und Universalität unter extremen Bedingungen“ (SFB1225/ISOQUANT) der Ruperto Carola durchgeführt. Prof. Stachel ist stellvertretende Sprecherin des von der Deutschen Forschungsgemeinschaft geförderten SFB 1225.
________________________________________________________________________________
Originalpublikation:
A. Andronic, P. Braun-Munzinger, K. Redlich und J. Stachel: Decoding the phase structure of QCD via particle
production at high energy, Nature 561, 321–330 (2018)
DOI:10.1038/s41586-018-0491-6
________________________________________________________________________________
Prof. Dr. Johanna Stachel
Physikalisches Institut
Tel. +49 06221 54 19501
stachel@physi.uni-heidelberg.de
Kommunikation und Marketing
Pressestelle
Tel. +49 6221 54-2311
presse@rektorat.uni-heidelberg.de
Pressemitteilung Nr. 113/2018, 20. September 2018
Etwa zehn Millionstel Sekunden dauerte es, bis die Bausteine der Materie unserer heutigen Alltagswelt entstanden, so die gängige Annahme von Experten: Nach dem Urknall vor 13,7 Milliarden Jahren bewegten sich Quarks und Gluonen, zwei Arten von Elementarteilchen, in den allerersten Augenblicken des Universums frei in einem Quark-Gluon-Plasma. Dann, in einem Phasenübergang, vereinigten sich diese Teilchen und bildeten Hadronen, darunter die Bausteine von Atomkernen – Protonen und Neutronen. Ein internationales Forscherteam präsentiert nun eine Analyse der Ergebnisse langjähriger Experimente an Teilchenbeschleunigern, die Licht auf die Natur dieses Phasenübergangs wirft.
Sie wurden unter anderem mit dem ALICE-Detektor am Large Hadron Collider des Europäischen Kernforschungszentrums CERN bei den weltweit höchsten Energien durchgeführt. Unter anderem bestimmten die Forscher mit Präzision die Übergangstemperatur und erhielten neue Erkenntnisse zu dem Mechanismus, wie ein Quark-Gluon-Plasma beim Abkühlen in die normalen Materiebausteine wie Protonen, Neutronen oder Atomkerne ausfriert. Das Forscherteam besteht aus Wissenschaftlern des GSI Helmholtzzentrums für Schwerionenforschung in Darmstadt sowie der Universitäten Heidelberg, Münster und Breslau (Polen). Ihre Ergebnisse veröffentlichten sie in „Nature“.
Die Heidelberger Forschungsgruppe am Physikalischen Institut der Universität Heidelberg unter Leitung von Prof. Dr. Johanna Stachel ist seit 1996 Mitglied von ALICE und hat sowohl im Aufbau des Experiments als auch im Betrieb und in der Datenanalyse eine Schlüsselfunktion inne. Prof. Stachel ist Leiterin einer der Hauptkomponenten von ALICE, des TRD-Projekts (Übergangsstrahlungsdetektor), und war stellvertretende Leiterin des TPC-Projekts (Spurendriftkammer). Mit ihrer Gruppe hat sie entscheidenden Anteil am erfolgreichen ALICE-Experiment. In Deutschland ist die Förderung für die LHC-Experimente in vier Forschungsschwerpunkten (FSP) des Bundesministeriums für Bildung und Forschung strukturiert. Die Wissenschaftlerin ist seit Beginn der FSPs Sprecherin des FSP202-ALICE. Die phänomenologischen Untersuchungen zur Interpretation der ALICE-Daten, die im Zentrum der aktuellen „Nature“-Veröffentlichung stehen, wurden im Rahmen des Sonderforschungsbereichs „Isolierte Quantensysteme und Universalität unter extremen Bedingungen“ (SFB1225/ISOQUANT) der Ruperto Carola durchgeführt. Prof. Stachel ist stellvertretende Sprecherin des von der Deutschen Forschungsgemeinschaft geförderten SFB 1225.
________________________________________________________________________________
Originalpublikation:
A. Andronic, P. Braun-Munzinger, K. Redlich und J. Stachel: Decoding the phase structure of QCD via particle
production at high energy, Nature 561, 321–330 (2018)
DOI:10.1038/s41586-018-0491-6
________________________________________________________________________________
Prof. Dr. Johanna Stachel
Physikalisches Institut
Tel. +49 06221 54 19501
stachel@physi.uni-heidelberg.de
Kommunikation und Marketing
Pressestelle
Tel. +49 6221 54-2311
presse@rektorat.uni-heidelberg.de
Press Release No. 80/2018, 27 June 2018
A system’s state is characterised as entangled or quantum correlated if two or more particles cannot be described as a combination of separate, independent states but only as a whole. Researchers at the Kirchhoff Institute for Physics of Heidelberg University recently succeeded in verifying so-called non-local quantum correlations between ultracold clouds of rubidium atoms. Under the direction of Prof. Dr Markus Oberthaler und Prof. Dr Thomas Gasenzer, the researchers were able to gain important new insights into the character of quantum mechanical many-body systems.
The correlations that the theory of quantum mechanics predicts are counter-intuitive. These quantum correlations seem to contradict the Heisenberg uncertainty principle, which states that two properties of an object, such as position and speed, can never be precisely determined at the same time. In quantum mechanical systems, however, two particles can be prepared so as to accurately predict the position of particle two by localising the position of particle one. Similarly, measuring the speed of one particle allows predicting the speed of the other. “In this case, the position and speed of particle two do need to be precisely determined prior to measurement,” explains Prof. Oberthaler. “The measurement result for particle one cannot be immediately present at particle two’s position if the two are spatially separate.”
The uncertainty principle actually does not support this simultaneous determination of position and speed. But in quantum mechanics, two objects are not considered separate if they are correlated, i.e., entangled, hence resolving the apparent contradiction. “If we can prove that measurement results of different observables in one system can actually be predicted by measuring a second, remote system, then we can use this evidence to substantiate entanglement as well – and that’s exactly what we did in our experiment,” states Philipp Kunkel, the study’s primary author.
In their experiment, the researchers used a cloud of approximately 11,000 rubidium atoms, which they cooled to extremely low temperatures. Using laser light, they kept the atoms suspended in a vacuum chamber, which allowed them to exclude any disturbing effects, such as collisions with air molecules. Because quantum effects are detectable only at very low temperatures, working with ultracold atoms is required. Like when measuring position and speed, these extreme conditions allow the internal state of the particles, often called spin, to be measured as well. “By measuring the spin in one half of the cloud, we were able to predict the spin in the other more accurately than the local uncertainty principle would allow,” explains Philipp Kunkel.
The characterisation of quantum mechanical many-body systems is important for future applications such as quantum computers and quantum communication, among others. The most recent Heidelberg research results were published in “Science”.
________________________________________________________________________________
Original publication:
P. Kunkel, M. Prüfer, H. Strobel, D. Linnemann, A. Frölian, T. Gasenzer, M. Gärttner, M.K. Oberthaler: Spatially
distributed multipartite entanglement enables EPR steering of atomic clouds, Science (published online 27
April 2018)
DOI:10.1126/science.aao2254
________________________________________________________________________________
Prof. Dr. Markus Oberthaler
Kirchhoff Institute for Physics
Phone +49 6221 54-5170
markus.oberthaler@kip.uni-heidelberg.de
Communications and Marketing
Press Office
Phone +49 6221 54-2311
presse@rektorat.uni-heidelberg.de
Press Release No. 80/2018, 27 June 2018
A system’s state is characterised as entangled or quantum correlated if two or more particles cannot be described as a combination of separate, independent states but only as a whole. Researchers at the Kirchhoff Institute for Physics of Heidelberg University recently succeeded in verifying so-called non-local quantum correlations between ultracold clouds of rubidium atoms. Under the direction of Prof. Dr Markus Oberthaler und Prof. Dr Thomas Gasenzer, the researchers were able to gain important new insights into the character of quantum mechanical many-body systems.
The correlations that the theory of quantum mechanics predicts are counter-intuitive. These quantum correlations seem to contradict the Heisenberg uncertainty principle, which states that two properties of an object, such as position and speed, can never be precisely determined at the same time. In quantum mechanical systems, however, two particles can be prepared so as to accurately predict the position of particle two by localising the position of particle one. Similarly, measuring the speed of one particle allows predicting the speed of the other. “In this case, the position and speed of particle two do need to be precisely determined prior to measurement,” explains Prof. Oberthaler. “The measurement result for particle one cannot be immediately present at particle two’s position if the two are spatially separate.”
The uncertainty principle actually does not support this simultaneous determination of position and speed. But in quantum mechanics, two objects are not considered separate if they are correlated, i.e., entangled, hence resolving the apparent contradiction. “If we can prove that measurement results of different observables in one system can actually be predicted by measuring a second, remote system, then we can use this evidence to substantiate entanglement as well – and that’s exactly what we did in our experiment,” states Philipp Kunkel, the study’s primary author.
In their experiment, the researchers used a cloud of approximately 11,000 rubidium atoms, which they cooled to extremely low temperatures. Using laser light, they kept the atoms suspended in a vacuum chamber, which allowed them to exclude any disturbing effects, such as collisions with air molecules. Because quantum effects are detectable only at very low temperatures, working with ultracold atoms is required. Like when measuring position and speed, these extreme conditions allow the internal state of the particles, often called spin, to be measured as well. “By measuring the spin in one half of the cloud, we were able to predict the spin in the other more accurately than the local uncertainty principle would allow,” explains Philipp Kunkel.
The characterisation of quantum mechanical many-body systems is important for future applications such as quantum computers and quantum communication, among others. The most recent Heidelberg research results were published in “Science”.
________________________________________________________________________________
Original publication:
P. Kunkel, M. Prüfer, H. Strobel, D. Linnemann, A. Frölian, T. Gasenzer, M. Gärttner, M.K. Oberthaler: Spatially
distributed multipartite entanglement enables EPR steering of atomic clouds, Science (published online 27
April 2018)
DOI:10.1126/science.aao2254
________________________________________________________________________________
Prof. Dr. Markus Oberthaler
Kirchhoff Institute for Physics
Phone +49 6221 54-5170
markus.oberthaler@kip.uni-heidelberg.de
Communications and Marketing
Press Office
Phone +49 6221 54-2311
presse@rektorat.uni-heidelberg.de
Pressemitteilung Nr. 80/2018, 27. Juni 2018
Als verschränkt oder auch quantenkorreliert wird der Zustand eines Systems bezeichnet, bei dem sich zwei oder mehr Teilchen nicht mehr als Kombination jeweils unabhängiger Zustände beschreiben lassen, sondern nur durch einen gemeinsamen Zustand. Wissenschaftlern am Kirchhoff-Institut für Physik der Universität Heidelberg ist es nun gelungen, sogenannte nichtlokale Quantenkorrelationen zwischen ultrakalten Wolken von Rubidium-Atomen nachzuweisen. Damit konnten die Forscher um Prof. Dr. Markus Oberthaler und Prof. Dr. Thomas Gasenzer wichtige neue Erkenntnisse zur Charakterisierung von quantenmechanischen Vielteilchensystemen gewinnen.
Die Theorie der Quantenmechanik sagt Korrelationen vorher, die der Intuition zuwiderlaufen. Solche Quantenkorrelationen scheinen im Widerspruch zu stehen zur Heisenbergschen Unschärferelation: Sie besagt, dass zwei Eigenschaften eines Objekts wie Ort und Geschwindigkeit nie gleichzeitig exakt bestimmt sein können. In quantenmechanischen Systemen lassen sich jedoch zwei Teilchen so präparieren, dass es die Ortsbestimmung von Teilchen eins möglich macht, die Position des zweiten exakt vorherzusagen. Genauso erlaubt die Geschwindigkeitsmessung des einen Teilchens die Vorhersage der Geschwindigkeit des anderen. „In diesem Fall müssen sowohl Ort als auch Geschwindigkeit von Teilchen zwei schon vor der Messung exakt bestimmt sein“, erläutert Prof. Oberthaler. „Das Messergebnis an Teilchen eins kann nicht sofort am Ort von Teilchen zwei vorliegen, wenn beide räumlich voneinander getrennt sind.“
Diese gleichzeitige Bestimmtheit von Ort und Geschwindigkeit ist aber durch die Unschärferelation eigentlich nicht möglich. Der scheinbare Widerspruch löst sich dadurch auf, dass in der quantenmechanischen Beschreibung nicht von zwei getrennten Objekten gesprochen werden kann, wenn diese korreliert, also verschränkt sind. „Wenn wir nachweisen können, dass sich Messergebnisse verschiedener Beobachtungsgrößen in einem System durch Messungen an einem zweiten, entfernten System tatsächlich vorhersagen lassen, dann können wir diesen Nachweis verwenden, um auch eine Verschränkung zu belegen – und genau das haben wir in unserem Experiment gezeigt“, so der Erstautor der Studie, Philipp Kunkel.
In ihrem Experiment benutzten die Forscher eine Wolke von rund 11.000 Rubidium-Atomen, die sie auf extrem niedrige Temperaturen kühlten und mit Laserlicht in einer Vakuumkammer in der Schwebe hielten. Damit sollten jegliche Störeffekte wie zum Beispiel Stöße mit Luft-Molekülen ausgeschlossen werden. Die Arbeit mit ultrakalten Atomen ist erforderlich, da Quanteneffekte erst bei sehr niedrigen Temperaturen nachweisbar werden. Unter diesen extremen Bedingungen ist es zudem möglich, vergleichbar zu Ort und Geschwindigkeit den internen Zustand der Teilchen, oftmals Spin genannt, zu messen. So konnten wir den Spin in der einen Hälfte der Wolke durch eine Messung an der anderen Hälfte genauer vorhersagen, als es die lokale Unschärferelation erlauben würde“, erklärt Philipp Kunkel.
Die Charakterisierung von quantenmechanischen Vielteilchensystemen ist unter anderem von Bedeutung für künftige Anwendungen wie Quantencomputer oder die Quantenkommunikation. Die aktuellen Heidelberger Forschungsergebnisse wurden in „Science“ veröffentlicht.
________________________________________________________________________________
Originalpublikation:
P. Kunkel, M. Prüfer, H. Strobel, D. Linnemann, A. Frölian, T. Gasenzer, M. Gärttner, M.K. Oberthaler: Spatially
distributed multipartite entanglement enables EPR steering of atomic clouds, Science (published online 27
April 2018)
DOI:10.1126/science.aao2254
________________________________________________________________________________
Prof. Dr. Markus Oberthaler
Kirchhoff-Institut für Physik
Tel. +49 6221 54-5170
markus.oberthaler@kip.uni-heidelberg.de
Kommunikation und Marketing
Pressestelle
Tel. +49 6221 54-2311
presse@rektorat.uni-heidelberg.de
Pressemitteilung Nr. 80/2018, 27. Juni 2018
Als verschränkt oder auch quantenkorreliert wird der Zustand eines Systems bezeichnet, bei dem sich zwei oder mehr Teilchen nicht mehr als Kombination jeweils unabhängiger Zustände beschreiben lassen, sondern nur durch einen gemeinsamen Zustand. Wissenschaftlern am Kirchhoff-Institut für Physik der Universität Heidelberg ist es nun gelungen, sogenannte nichtlokale Quantenkorrelationen zwischen ultrakalten Wolken von Rubidium-Atomen nachzuweisen. Damit konnten die Forscher um Prof. Dr. Markus Oberthaler und Prof. Dr. Thomas Gasenzer wichtige neue Erkenntnisse zur Charakterisierung von quantenmechanischen Vielteilchensystemen gewinnen.
Die Theorie der Quantenmechanik sagt Korrelationen vorher, die der Intuition zuwiderlaufen. Solche Quantenkorrelationen scheinen im Widerspruch zu stehen zur Heisenbergschen Unschärferelation: Sie besagt, dass zwei Eigenschaften eines Objekts wie Ort und Geschwindigkeit nie gleichzeitig exakt bestimmt sein können. In quantenmechanischen Systemen lassen sich jedoch zwei Teilchen so präparieren, dass es die Ortsbestimmung von Teilchen eins möglich macht, die Position des zweiten exakt vorherzusagen. Genauso erlaubt die Geschwindigkeitsmessung des einen Teilchens die Vorhersage der Geschwindigkeit des anderen. „In diesem Fall müssen sowohl Ort als auch Geschwindigkeit von Teilchen zwei schon vor der Messung exakt bestimmt sein“, erläutert Prof. Oberthaler. „Das Messergebnis an Teilchen eins kann nicht sofort am Ort von Teilchen zwei vorliegen, wenn beide räumlich voneinander getrennt sind.“
Diese gleichzeitige Bestimmtheit von Ort und Geschwindigkeit ist aber durch die Unschärferelation eigentlich nicht möglich. Der scheinbare Widerspruch löst sich dadurch auf, dass in der quantenmechanischen Beschreibung nicht von zwei getrennten Objekten gesprochen werden kann, wenn diese korreliert, also verschränkt sind. „Wenn wir nachweisen können, dass sich Messergebnisse verschiedener Beobachtungsgrößen in einem System durch Messungen an einem zweiten, entfernten System tatsächlich vorhersagen lassen, dann können wir diesen Nachweis verwenden, um auch eine Verschränkung zu belegen – und genau das haben wir in unserem Experiment gezeigt“, so der Erstautor der Studie, Philipp Kunkel.
In ihrem Experiment benutzten die Forscher eine Wolke von rund 11.000 Rubidium-Atomen, die sie auf extrem niedrige Temperaturen kühlten und mit Laserlicht in einer Vakuumkammer in der Schwebe hielten. Damit sollten jegliche Störeffekte wie zum Beispiel Stöße mit Luft-Molekülen ausgeschlossen werden. Die Arbeit mit ultrakalten Atomen ist erforderlich, da Quanteneffekte erst bei sehr niedrigen Temperaturen nachweisbar werden. Unter diesen extremen Bedingungen ist es zudem möglich, vergleichbar zu Ort und Geschwindigkeit den internen Zustand der Teilchen, oftmals Spin genannt, zu messen. So konnten wir den Spin in der einen Hälfte der Wolke durch eine Messung an der anderen Hälfte genauer vorhersagen, als es die lokale Unschärferelation erlauben würde“, erklärt Philipp Kunkel.
Die Charakterisierung von quantenmechanischen Vielteilchensystemen ist unter anderem von Bedeutung für künftige Anwendungen wie Quantencomputer oder die Quantenkommunikation. Die aktuellen Heidelberger Forschungsergebnisse wurden in „Science“ veröffentlicht.
________________________________________________________________________________
Originalpublikation:
P. Kunkel, M. Prüfer, H. Strobel, D. Linnemann, A. Frölian, T. Gasenzer, M. Gärttner, M.K. Oberthaler: Spatially
distributed multipartite entanglement enables EPR steering of atomic clouds, Science (published online 27
April 2018)
DOI:10.1126/science.aao2254
________________________________________________________________________________
Prof. Dr. Markus Oberthaler
Kirchhoff-Institut für Physik
Tel. +49 6221 54-5170
markus.oberthaler@kip.uni-heidelberg.de
Kommunikation und Marketing
Pressestelle
Tel. +49 6221 54-2311
presse@rektorat.uni-heidelberg.de
Pressemitteilung Nr. 148/2017, 21. November 2017
Mit der Erforschung von Modellsystemen für magnetische Materialien beschäftigt sich eine neue Emmy Noether-Nachwuchsgruppe, die am Kirchhoff-Institut für Physik der Universität Heidelberg ihre Arbeit aufgenommen hat. Das Team unter der Leitung von Juniorprofessor Dr. Fred Jendrzejewski nutzt dazu Experimente mit ultrakalten Gasen. Die Deutsche Forschungsgemeinschaft (DFG) fördert die Gruppe des Quantenphysikers über einen Zeitraum von fünf Jahren mit rund 1,5 Millionen Euro. Sie erweitert Prof. Jendrzejewskis bereits bestehende Forschungsgruppe „Complex Quantum Matter“.
„Mit unseren Arbeiten konzentrieren wir uns auf die Frage, wie die Eigenschaften von Quantensystemen durch ihre mikroskopischen Bestandteile erklärt und manipuliert werden können. Wir beschäftigen uns zum Beispiel mit Magnetismus, welcher ursprünglich in der Festkörperphysik untersucht wurde“, erläutert Prof. Jendrzejewski. „Dabei wollen wir insbesondere herausfinden, welche Rolle die Quantenmechanik in dieser komplexen Fragestellung spielt.“ Für ihre Untersuchungen nutzen die Forscher Experimente mit ultrakalten, atomaren Gasen, da sie vielseitig einsetzbar sind und eine hohe Genauigkeit ermöglichen. Von ihrer Arbeit erhofft sich die Gruppe ein grundlegend verbessertes Verständnis magnetischer Materialien.
Fred Jendrzejewski (Jahrgang 1986) studierte Physik an der Technischen Universität Dresden und der Universität Hamburg. Weitere Studienaufenthalte führten ihn an die École Polytechnique in Paris sowie an die Universität Paris-Süd, an der er im Jahr 2012 promoviert wurde. Als Postdoktorand wirkte Fred Jendrzejewski für drei Jahre in den USA am Joint Quantum Institute, einer gemeinsamen Forschungseinrichtung der University of Maryland und des National Institute of Standards and Technology. Seit 2015 ist der Wissenschaftler als Juniorprofessor am Kirchhoff-Institut für Physik der Universität Heidelberg tätig.
Juniorprofessor Dr. Fred Jendrzejewski
Kirchhoff-Institut für Physik
Telefon (06221) 54-5182
fnj@kip.uni-heidelberg.de
Heidelberg University
Communications and Marketing
Press Office, phone +49 6221 54-2311
presse@rektorat.uni-heidelberg.de
Pressemitteilung Nr. 148/2017, 21. November 2017
Mit der Erforschung von Modellsystemen für magnetische Materialien beschäftigt sich eine neue Emmy Noether-Nachwuchsgruppe, die am Kirchhoff-Institut für Physik der Universität Heidelberg ihre Arbeit aufgenommen hat. Das Team unter der Leitung von Juniorprofessor Dr. Fred Jendrzejewski nutzt dazu Experimente mit ultrakalten Gasen. Die Deutsche Forschungsgemeinschaft (DFG) fördert die Gruppe des Quantenphysikers über einen Zeitraum von fünf Jahren mit rund 1,5 Millionen Euro. Sie erweitert Prof. Jendrzejewskis bereits bestehende Forschungsgruppe „Complex Quantum Matter“.
„Mit unseren Arbeiten konzentrieren wir uns auf die Frage, wie die Eigenschaften von Quantensystemen durch ihre mikroskopischen Bestandteile erklärt und manipuliert werden können. Wir beschäftigen uns zum Beispiel mit Magnetismus, welcher ursprünglich in der Festkörperphysik untersucht wurde“, erläutert Prof. Jendrzejewski. „Dabei wollen wir insbesondere herausfinden, welche Rolle die Quantenmechanik in dieser komplexen Fragestellung spielt.“ Für ihre Untersuchungen nutzen die Forscher Experimente mit ultrakalten, atomaren Gasen, da sie vielseitig einsetzbar sind und eine hohe Genauigkeit ermöglichen. Von ihrer Arbeit erhofft sich die Gruppe ein grundlegend verbessertes Verständnis magnetischer Materialien.
Fred Jendrzejewski (Jahrgang 1986) studierte Physik an der Technischen Universität Dresden und der Universität Hamburg. Weitere Studienaufenthalte führten ihn an die École Polytechnique in Paris sowie an die Universität Paris-Süd, an der er im Jahr 2012 promoviert wurde. Als Postdoktorand wirkte Fred Jendrzejewski für drei Jahre in den USA am Joint Quantum Institute, einer gemeinsamen Forschungseinrichtung der University of Maryland und des National Institute of Standards and Technology. Seit 2015 ist der Wissenschaftler als Juniorprofessor am Kirchhoff-Institut für Physik der Universität Heidelberg tätig.
Juniorprofessor Dr. Fred Jendrzejewski
Kirchhoff-Institut für Physik
Telefon (06221) 54-5182
fnj@kip.uni-heidelberg.de
Heidelberg University
Communications and Marketing
Press Office, phone +49 6221 54-2311
presse@rektorat.uni-heidelberg.de
Press Release No. 148/2017, 21 November 2017
A new Emmy Noether junior research group has taken up its work investigating model systems for magnetic materials at the Kirchhoff Institute for Physics at Heidelberg University. The team under the direction of Junior Professor Dr Fred Jendrzejewski is using experiments with ultracold gases. Over the next five years, the group of quantum physicists will receive funding from the German Research Foundation (DFG) totalling approximately 1.5 million euros. The group is an extension of Prof. Jendrzejewski’s existing “Complex Quantum Matter” research group.
“We are concentrating our work on how the properties of quantum systems can be explained and manipulated through their microscopic components. For example, we are working on magnetism, which was originally investigated in solid state physics,” states Prof. Jendrzejewski. “In particular, we want to explore the role quantum mechanics plays in this complex enquiry.” In their experiments, the researchers are working with ultracold atomic gases, which are known for their extreme versatility and accuracy. The group hopes their work will result in a fundamentally better understanding of magnetic materials.
Fred Jendrzejewski (b. 1986) studied physics at the Dresden University of Technology and the University of Hamburg. Study abroad took him to the École Polytechnique in Paris and the University of Paris-Sud, where he earned his doctorate in 2012. As a postdoc, Fred Jendrzejewski worked for three years in the USA at the Joint Quantum Institute, a research facility of the University of Maryland and the National Institute of Standards and Technology. The researcher has been a Junior Professor at the Kirchhoff Institute for Physics at Heidelberg University since 2015.
Junior Professor Dr Fred Jendrzejewski
Kirchhoff Institute for Physics
Phone: +49 6221 54-5182
fnj@kip.uni-heidelberg.de
Heidelberg University
Communications and Marketing
Press Office, phone +49 6221 54-2311
presse@rektorat.uni-heidelberg.de
Press Release No. 148/2017, 21 November 2017
A new Emmy Noether junior research group has taken up its work investigating model systems for magnetic materials at the Kirchhoff Institute for Physics at Heidelberg University. The team under the direction of Junior Professor Dr Fred Jendrzejewski is using experiments with ultracold gases. Over the next five years, the group of quantum physicists will receive funding from the German Research Foundation (DFG) totalling approximately 1.5 million euros. The group is an extension of Prof. Jendrzejewski’s existing “Complex Quantum Matter” research group.
“We are concentrating our work on how the properties of quantum systems can be explained and manipulated through their microscopic components. For example, we are working on magnetism, which was originally investigated in solid state physics,” states Prof. Jendrzejewski. “In particular, we want to explore the role quantum mechanics plays in this complex enquiry.” In their experiments, the researchers are working with ultracold atomic gases, which are known for their extreme versatility and accuracy. The group hopes their work will result in a fundamentally better understanding of magnetic materials.
Fred Jendrzejewski (b. 1986) studied physics at the Dresden University of Technology and the University of Hamburg. Study abroad took him to the École Polytechnique in Paris and the University of Paris-Sud, where he earned his doctorate in 2012. As a postdoc, Fred Jendrzejewski worked for three years in the USA at the Joint Quantum Institute, a research facility of the University of Maryland and the National Institute of Standards and Technology. The researcher has been a Junior Professor at the Kirchhoff Institute for Physics at Heidelberg University since 2015.
Junior Professor Dr Fred Jendrzejewski
Kirchhoff Institute for Physics
Phone: +49 6221 54-5182
fnj@kip.uni-heidelberg.de
Heidelberg University
Communications and Marketing
Press Office, phone +49 6221 54-2311
presse@rektorat.uni-heidelberg.de
Pressemitteilung Nr. 66/2016, 27. Mai 2016
Mit vier Anträgen für die Förderung großer Forschungsverbünde ist die Universität Heidelberg in der aktuellen Bewilligungsrunde der Deutschen Forschungsgemeinschaft (DFG) erfolgreich: So fördert die DFG einen neuen Sonderforschungsbereich/Transregio (SFB/TRR), der an der Medizinischen Fakultät Heidelberg angesiedelt ist: Hier werden sich Wissenschaftler aus Heidelberg, Freiburg und München mit Infektionen durch Hepatitis-Viren befassen. Hinzu kommt ein weiterer neu bewilligter SFB/TRR auf dem Gebiet der molekularen Lebenswissenschaften; Heidelberger und Berliner Wissenschaftler untersuchen in diesem Verbund die Koordination der Signalübermittlung in lebenden Zellen. Neu eingerichtet wird außerdem ein SFB in der Physik, an dem isolierte Quantensysteme unter extremen Bedingungen in Experiment und Theorie erforscht werden. In eine zweite Förderperiode geht der molekularbiologische SFB 1036 „Zelluläre Qualitätskontrolle und Schadensbegrenzung“ der Universität Heidelberg. Für alle vier Verbünde, die jeweils über vier Jahre gefördert werden, stellt die DFG Fördermittel in Höhe von insgesamt mehr als 46 Millionen Euro zur Verfügung.
SFB/TRR 179 „Ursachen der Ausheilung oder Chronifizierung von Infektionen mit Hepatitis-Viren“
Die Wissenschaftler im Sonderforschungsbereich/Transregio 179 gehen am Beispiel der Infektion mit verschiedenen Hepatitis-Viren der Frage nach, welche Faktoren den Ausschlag dafür geben, dass Infektionen ausheilen oder einen chronischen Verlauf nehmen. Wie Prof. Dr. Ralf Bartenschlager erläutert, unterscheiden sich die Vermehrungsstrategien beispielsweise der Hepatitis-B-Viren und der Hepatitis-C-Viren grundsätzlich. „Darüber ist inzwischen viel bekannt. Wir wissen allerdings nicht, wie es die Viren jeweils schaffen, die körpereigene Abwehr zu überlisten“, sagt der Sprecher des neuen SFB/TRR „Ursachen der Ausheilung oder Chronifizierung von Infektionen mit Hepatitis-Viren“. Nach den Worten von Prof. Bartenschlager hat sich die Forschung bislang hauptsächlich darauf konzentriert, einzelne Signalwege zu entschlüsseln oder wichtige molekulare Faktoren zu identifizieren. „Wenn wir allerdings die generellen Mechanismen verstehen wollen, die darüber entscheiden, ob eine Virus-Infektion chronisch wird oder spontan ausheilt, brauchen wir einen integrativen Ansatz, der die komplexen Wechselwirkungen zwischen Viren und Wirtsorganismus von verschiedenen Seiten beleuchtet“, so der Virologe, der Direktor der Abteilung für Molekulare Virologie am Zentrum für Infektiologie des Universitätsklinikums Heidelberg ist und den Forschungsschwerpunkt „Infektionen, Entzündung und Krebs“ am Deutschen Krebsforschungszentrum (DKFZ) leitet. Neben weiteren Wissenschaftlern der Universität Heidelberg sind Teams des Universitätsklinikums Freiburg, der Technischen Universität München, der Ludwig-Maximilians-Universität München sowie des DKFZ an den insgesamt 21 Teilprojekten beteiligt. Der SFB/TRR 179 erhält Fördermittel in Höhe von rund 12,1 Millionen Euro.
SFB/TRR 186 „Molekulare Schalter in der Raum-Zeit-Kontrolle der zellulären Signaltransmission“
Zentrales Forschungsthema des SFB/TRR 186 „Molekulare Schalter in der Raum-Zeit-Kontrolle der zellulären Signaltransmission“ sind die Koordinierungsprozesse bei der Übermittlung von Signalen in lebenden Zellen, die in der Funktionalität von biologischen Systemen eine zentrale Rolle spielen. Weitgehend unbekannt ist dabei aber, wie die Signale von aktivierten molekularen Schaltern räumlich und zeitlich koordiniert werden, wie Prof. Dr. Walter Nickel vom Biochemie-Zentrum der Universität Heidelberg erläutert. Der neue Forschungsverbund basiert auf der Entwicklung einer großen Zahl von chemisch-biologischen Werkzeugen, die auf breiter Basis für eine direkte „Manipulation“ von molekularen Schaltern angewendet werden können. Mit Hilfe der extrem hochauflösenden Lichtmikroskopie sollen diese Techniken für systematische Untersuchungen in lebenden Zellen eingesetzt werden. Aufbauend darauf wollen die Wissenschaftler theoretische Modelle zur Aufklärung der räumlichen und zeitlichen Regulation zellulärer Signalübertragungsprozesse entwickeln, so Prof. Nickel, der die Sprecherfunktion des Konsortiums übernimmt. Der SFB/TRR wird 18 wissenschaftliche Teilprojekte und zwei technische Plattformen umfassen. Beteiligt sind daran neben der Universität Heidelberg, der Freien Universität Berlin und der Humboldt-Universität zu Berlin auch Forscher des Universitätsklinikums Heidelberg und der Charité – Universitätsmedizin Berlin. Als außeruniversitäre Einrichtungen werden das Deutsche Krebsforschungszentrum (DKFZ) und das Europäische Laboratorium für Molekularbiologie (EMBL) in Heidelberg sowie das Leibniz-Institut für Molekulare Pharmakologie in Berlin in diesem Verbund mitwirken. Der SFB/TRR 186 erhält DFG-Fördermittel in Höhe von rund 11,8 Millionen Euro.
SFB 1225 „Isolierte Quantensysteme und Universalität unter extremen Bedingungen“ (ISOQUANT)
Im Mittelpunkt des SFB 1225 „Isolierte Quantensysteme und Universalität unter extremen Bedingungen“ (ISOQUANT) steht ein Forschungsthema, das für eine Vielzahl von Anwendungen – von der Teilchenphysik über die Kernphysik bis zur Atom- und Festkörperphysik – von großer Bedeutung ist. Viele dieser Systeme zeigen ähnliche Eigenschaften, obwohl wesentliche Parameter wie Temperatur, Dichte oder Feldstärke sehr verschieden sind. Es existieren sogar universelle Bereiche, in denen quantitative Übereinstimmungen zwischen scheinbar grundverschiedenen physikalischen Systemen beobachtet werden können. Die am Sonderforschungsbereich beteiligten Wissenschaftler werden dabei sowohl zeitabhängige Phänomene als auch Gleichgewichtseigenschaften mit gemeinsamen Herangehensweisen untersuchen, um mit diesem neuen Ansatz aktuelle Forschungsfragen fachübergreifend bearbeiten zu können. Neben dem sogenannten Thermalisierungsprozess isolierter Quantensysteme geht es dabei um das Wechselspiel zwischen starken elektromagnetischen oder stark wechselwirkenden Feldern und dem Vakuum oder Materie sowie um die Phasenstruktur von Systemen unter extremen Bedingungen. Im Sonderforschungsbereich ISOQUANT haben sich Arbeitsgruppen des Instituts für Theoretische Physik, des Kirchhoff-Instituts für Physik und des Physikalischen Instituts der Universität Heidelberg sowie Wissenschaftler des Max-Planck-Instituts für Kernphysik in Heidelberg und der Technischen Universität Wien (Österreich) zusammengeschlossen. Die Funktion des Sprechers übernimmt Prof. Dr. Jürgen Berges, der an der Ruperto Carola auf dem Gebiet der Theoretischen Physik forscht. Die DFG fördert den SFB, der 14 Teilprojekte umfasst, mit rund 10,5 Millionen Euro.
SFB 1036 „Zelluläre Qualitätskontrolle und Schadensbegrenzung“
Im Mittelpunkt des SFB 1036 stehen die Überwachungs- und Qualitätskontrollsysteme, die Zellen vor Schäden oder einer Störung des zellulären Gleichgewichts bewahren. Diese Systeme registrieren Defekte und lösen schützende Stressantworten aus, die das Überleben der Zellen ermöglichen sollen. Die Wissenschaftler des Sonderforschungsbereichs, der am Zentrum für Molekulare Biologie der Universität Heidelberg (ZMBH) angesiedelt ist, untersuchen diese Prozesse auf molekularer und systemischer Ebene. Im Mittelpunkt stehen dabei die Überwachungssysteme der DNA, RNA und Proteine, die anhand verschiedener Modellorganismen erforscht werden. In den kommenden vier Jahren sollen die Arbeiten durch eine strukturbiologische Analyse der Kontrollmechanismen von Proteinen auf atomarer Ebene erweitert werden. Weitere zentrale Aspekte sind die Qualitätskontrolle an der Schnittstelle von Proteinüberwachung und Proteinsynthese sowie die Sicherung der Genomstabilität. Darüber hinaus wird es um Modelle für Stressantworten in multizellulären Organismen gehen. „Unser langfristiges Ziel ist es, ein übergreifendes, mechanistisches Verständnis der zellulären Überwachungssysteme und Schadensantworten für alle Organismen zu entwickeln“, betont ZMBH-Direktor Prof. Dr. Bernd Bukau, der Sprecher des SFB 1036 „Zelluläre Qualitätskontrolle und Schadensbegrenzung“ ist. An den 20 Teilprojekten des Sonderforschungsbereichs wirken neben Biowissenschaftlern und Medizinern der Ruperto Carola auch Wissenschaftler des Deutschen Krebsforschungszentrums (DKFZ) sowie des Europäischen Laboratoriums für Molekularbiologie (EMBL) in Heidelberg mit. Die zentrale Koordination liegt am ZMBH. Prof. Bukau leitet eine Brückenabteilung am DKFZ und ist Ko‐Direktor der DKFZ‐ZMBH‐Allianz. In der zweiten Förderperiode wird der SFB 1036 von der DFG mit rund 12,1 Millionen Euro gefördert.
________________________________________________________________________________
Kommunikation und Marketing
Pressestelle
Tel. +49 6221 54-2311
presse@rektorat.uni-heidelberg.de
Press Release No. 66/2016, 27 May 2016
Heidelberg University succeeded in gaining support from the German Research Foundation (DFG) in the latest approval round, recieving funding for four research networks. The DFG granted funds to the new Transregional Collaborative Research Centre (CRC/TRR) of the Heidelberg Medical Faculty, where researchers from Heidelberg, Freiburg and Munich will investigate viral hepatitis infections. In the molecular life sciences, Heidelberg and Berlin scientists in another new CRC/TRR will study the coordination of signal transmission in living cells. A new CRC, this one in the field of physics, will explore in experiments and theory isolated quantum systems under extreme conditions. In molecular biology, the “Cellular Surveillance and Damage Response” CRC 1036 has been approved for a second funding period. Total DFG funding for all four consortia is over 46 million euros for a period of four years.
CRC/TRR 179, “Determinants and Dynamics of Elimination versus Persistence of Hepatitis Virus Infection”
Researchers in Transregional Collaborative Research Centre 179 will study infections caused by various hepatitis viruses to determine the critical factors in healing or a chronic disease course. As Prof. Dr. Ralf Bartenschlager explains, the reproduction strategies of the hepatitis B and hepatitis C viruses, for example, fundamentally differ. “Although we do know a lot, what we don’t know is how the viruses manage to outsmart the body’s defences,” says the spokesperson for the new CRC/TRR, “Determinants and Dynamics of Elimination versus Persistence of Hepatitis Virus Infection”. Until now, research concentrated mainly on decoding individual signal pathways or identifying critical molecular factors. “But if we want to understand the general mechanisms that determine whether a viral infection becomes chronic or spontaneously heals, we need an integrative approach that examines the complex interactions between viruses and the host organism from different sides,” says the virologist, who is the Director of the Molecular Virology Department at the Centre for Infectious Diseases of Heidelberg University Hospital and heads the “Infection, Inflammation and Cancer” Department at the German Cancer Research Center (DKFZ). In addition to researchers from Heidelberg University, teams from the Freiburg University Hospital, the Technical University of Munich, the Ludwig Maximilians University of Munich and the DKFZ will be involved in the 21 total subprojects. CRC/TRR 179 will receive funding in the amount of approx. 12.1 million euros.
CRC/TRR 186, “Molecular Switches in the Spacio-temporal Control of Cellular Signal Transmission”
The research of CRC/TRR 186, “Molecular Switches in the Spacio-temporal Control of Cellular Signal Transmission”, will focus on the coordination processes in the transmission of signals in living cells that play a pivotal role in the functionality of biological systems. According to Prof. Dr. Walter Nickel of the Heidelberg University Biochemistry Center, how activated molecular switches coordinate the signals in time and space remains largely unknown. The new research network is based on the development of a large number of chemical-biological tools that can be used for broad-based, direct manipulation of molecular switches. Using extremely high-resolution light microscopy, these technologies are to be used for systematic studies in living cells. On that basis, the researchers plan on developing theoretical models to explain the spatial and temporal regulation of cellular signal transmission processes, according to Prof. Nickel, spokesperson for the consortium. The CRC/TRR will incorporate 18 scientific subprojects and two technical platforms. In addition to Heidelberg University, other participating universities include Berlin’s Free University and Humboldt University along with researchers from Heidelberg University Hospital and Charité – University Hospital Berlin. Collaborating non-university institutions include the German Cancer Research Center (DKFZ), the European Laboratory for Molecular Biology (EMBL) in Heidelberg and the Leibniz Institute for Molecular Pharmacology in Berlin. CRC/TRR 186 will receive DFG funding in the amount of approx. 11.8 million euros.
CRC 1225, “Isolated Quantum Systems and Universality under Extreme Conditions” (ISOQUANT)
The “Isolated Quantum Systems and Universality under Extreme Conditions” (ISOQUANT) CRC is focused on a research area of major importance for numerous applications – from particle physics and nuclear physics to atomic and solid state physics. Many of these systems evidence similar properties even though essential parameters such as temperature, density and field strength are markedly different. There are even universal areas in which quantitative equivalents between seemingly completely different physical systems can be observed. CRC 1225 researchers will study time-dependent phenomena as well as equilibrium properties using common approaches to support cross-discipline investigation of current questions in research. Areas under study include the thermalisation process of isolated quantum systems, the interaction of highly electromagnetic or interactive fields with a vacuum or matter, and the phase structure of systems under extreme conditions. Members of the ISOQUANT CRC include working groups from the Institute for Theoretical Physics, the Kirchhoff Institute for Physics and the Institute for Physics of Heidelberg University as well as researchers from the Max Planck Institute for Nuclear Physics in Heidelberg and the Vienna University of Technology in Austria. Theoretical physicist Prof. Dr. Jürgen Berges of Ruperto Carola will serve as the CRC spokesperson. The CRC, which comprises 14 subprojects, will receive DFG funding in the amount of approx. 10.5 million euros.
CRC 1036, “Cellular Surveillance and Damage Response”
CRC 1036 is devoted to the study of surveillance and quality control systems that safeguard cells from damage or disruption of cellular equilibrium. These systems register defects and release protective stress responses intended to support the survival of the cell. The investigators of the Collaborative Research Centre, which is located at the Centre for Molecular Biology of Heidelberg University (ZMBH), are examining these processes at the molecular and systemic level. Of particular interest are the surveillance systems of DNA, RNA and proteins, which are being investigated based on different model organisms. Over the next four years, the CRC will expand its research to include the structural biological analysis of the control mechanisms of proteins at the atomic level. Other central topics include quality control at the interface of protein surveillance and protein synthesis and safeguarding genome stability. Models for stress responses in multicellular organisms are also being studied. “Our long-term goal is to develop a comprehensive mechanistic understanding of the cellular surveillance systems and damage responses for all organisms,” emphasises ZMBH Director Prof. Dr. Bernd Bukau, spokesperson for CRC 1036, “Cellular Surveillance and Damage Response”. In addition to bioscientists and medical scientists at Ruperto Carola, researchers from the German Cancer Research Center (DKFZ) and the European Laboratory for Molecular Biology (EMBL) in Heidelberg are also involved in the CRC’s 20 subprojects. The ZMBH is providing central coordination. Prof. Bukau heads a cross-departmental group at the DKFZ and Co‐director of the DKFZ‐ZMBH Alliance. In the second funding period, CRC 1036 will receive approx. 12.1 million euros in funding from the DFG.
________________________________________________________________________________
Communications and Marketing
Press Office
Phone +49 6221 54-2311
presse@rektorat.uni-heidelberg.de
Pressemitteilung vom 18. Mai 2017
Eine neue Art der Vermessung von Vielteilchen-Quantensystemen haben Wissenschaftler der Technischen Universität Wien in Kooperation mit Physikern der Universität Heidelberg entwickelt. Dabei geht es um sogenannte Quantenfeldtheorien: Diese beschreiben Teilchen nicht getrennt voneinander, sondern als zusammenhängende Felder, die unser ganzes Universum erfüllen. Am Vienna Center for Quantum Science and Technology konnte nun gezeigt werden, wie sich diese Theorien in Experimenten gezielt testen lassen, obwohl sie sonst zum Teil nur sehr schwer experimentell zugänglich sind. Dazu haben die Forscher ein Quantensystem aus tausenden von ultrakalten Atomen hergestellt. Diese Atomwolke wird – festgehalten in einer magnetischen Falle auf einem Atomchip – zu einem Quanten-Simulator, mit dem sich grundlegende physikalische Quantenprozesse im Labor nachbauen und untersuchen lassen. Die aktuellen Forschungsergebnisse wurden in „Nature“ veröffentlicht.
Wird die Atomwolke mit hoher Präzision vermessen, lässt sich feststellen: Nicht an jedem Punkt ist die Wahrscheinlichkeit, ein Atom zu finden, gleich groß. In der Quantenphysik stehen diese Wahrscheinlichkeiten an unterschiedlichen Orten miteinander in Verbindung. Sie geben damit Auskunft über die grundlegenden Naturgesetze, die das Verhalten einer solchen Wolke von Atomen auf dem Level von Quanten bestimmen. Die Korrelationsfunktionen, mit denen diese Zusammenhänge mathematisch beschrieben werden, gelten in der theoretischen Physik als ein äußerst wichtiges Instrument zur Charakterisierung von Quantensystemen, wie der Heidelberger Physiker Prof. Dr. Jürgen Berges erläutert. Allerdings waren wichtige Korrelationsfunktionen hoher Ordnung, bei denen die Zusammenhänge zwischen den Ergebnissen von Messungen an vielen verschiedenen Punkten im Raum bestimmt werden, im Experiment bisher kaum zugänglich. Der Quanten-Simulator macht es nun möglich, Korrelationen bis hin zur zehnten Ordnung präzise zu vermessen.
Anhand dieser hochkorrelierten Quantensysteme mit ultrakalten Atomen, deren Bedingungen mit der Atomwolke in einer magnetischen Falle nachgestellt werden, können die Wissenschaftler unterschiedliche Quantentheorien im Experiment testen. Damit wollen sie unter anderem die gemeinsamen physikalischen Grundlagen sehr unterschiedlicher Systeme ermitteln. Das ist auch das Ziel des von der Deutschen Forschungsgemeinschaft geförderten Sonderforschungsbereichs „Isolierte Quantensysteme und Universalität unter extremen Bedingungen“, der zum Juli 2016 an der Universität Heidelberg seine Arbeit aufgenommen hat. Im SFB ISOQUANT haben sich verschiedene physikalische Arbeitsgruppen der Ruperto Carola sowie Wissenschaftler des Max-Planck-Instituts für Kernphysik in Heidelberg und der Technischen Universität Wien zusammengeschlossen. Sprecher ist Prof. Berges vom Institut für Theoretische Physik der Universität Heidelberg.
________________________________________________________________________________
Originalpublikation:
T. Schweigler, V. Kasper, S. Erne, I. Mazets, B. Rauer, F. Cataldini, T. Langen, T. Gasenzer, J. Berges, and J.
Schmiedmayer: Experimental characterization of a quantum many-body system via higher-order correlations,
Nature 545 (2017).
https://doi.org/10.1038/nature22310
________________________________________________________________________________
Prof. Dr. Jürgen Berges
Institut für Theoretische Physik
Telefon (06221) 54-9422
berges@thphys.uni-heidelberg.de
Kommunikation und Marketing
Pressestelle
Tel. +49 6221 54-2311
presse@rektorat.uni-heidelberg.de
Pressemitteilung vom 18. Mai 2017
Eine neue Art der Vermessung von Vielteilchen-Quantensystemen haben Wissenschaftler der Technischen Universität Wien in Kooperation mit Physikern der Universität Heidelberg entwickelt. Dabei geht es um sogenannte Quantenfeldtheorien: Diese beschreiben Teilchen nicht getrennt voneinander, sondern als zusammenhängende Felder, die unser ganzes Universum erfüllen. Am Vienna Center for Quantum Science and Technology konnte nun gezeigt werden, wie sich diese Theorien in Experimenten gezielt testen lassen, obwohl sie sonst zum Teil nur sehr schwer experimentell zugänglich sind. Dazu haben die Forscher ein Quantensystem aus tausenden von ultrakalten Atomen hergestellt. Diese Atomwolke wird – festgehalten in einer magnetischen Falle auf einem Atomchip – zu einem Quanten-Simulator, mit dem sich grundlegende physikalische Quantenprozesse im Labor nachbauen und untersuchen lassen. Die aktuellen Forschungsergebnisse wurden in „Nature“ veröffentlicht.
Wird die Atomwolke mit hoher Präzision vermessen, lässt sich feststellen: Nicht an jedem Punkt ist die Wahrscheinlichkeit, ein Atom zu finden, gleich groß. In der Quantenphysik stehen diese Wahrscheinlichkeiten an unterschiedlichen Orten miteinander in Verbindung. Sie geben damit Auskunft über die grundlegenden Naturgesetze, die das Verhalten einer solchen Wolke von Atomen auf dem Level von Quanten bestimmen. Die Korrelationsfunktionen, mit denen diese Zusammenhänge mathematisch beschrieben werden, gelten in der theoretischen Physik als ein äußerst wichtiges Instrument zur Charakterisierung von Quantensystemen, wie der Heidelberger Physiker Prof. Dr. Jürgen Berges erläutert. Allerdings waren wichtige Korrelationsfunktionen hoher Ordnung, bei denen die Zusammenhänge zwischen den Ergebnissen von Messungen an vielen verschiedenen Punkten im Raum bestimmt werden, im Experiment bisher kaum zugänglich. Der Quanten-Simulator macht es nun möglich, Korrelationen bis hin zur zehnten Ordnung präzise zu vermessen.
Anhand dieser hochkorrelierten Quantensysteme mit ultrakalten Atomen, deren Bedingungen mit der Atomwolke in einer magnetischen Falle nachgestellt werden, können die Wissenschaftler unterschiedliche Quantentheorien im Experiment testen. Damit wollen sie unter anderem die gemeinsamen physikalischen Grundlagen sehr unterschiedlicher Systeme ermitteln. Das ist auch das Ziel des von der Deutschen Forschungsgemeinschaft geförderten Sonderforschungsbereichs „Isolierte Quantensysteme und Universalität unter extremen Bedingungen“, der zum Juli 2016 an der Universität Heidelberg seine Arbeit aufgenommen hat. Im SFB ISOQUANT haben sich verschiedene physikalische Arbeitsgruppen der Ruperto Carola sowie Wissenschaftler des Max-Planck-Instituts für Kernphysik in Heidelberg und der Technischen Universität Wien zusammengeschlossen. Sprecher ist Prof. Berges vom Institut für Theoretische Physik der Universität Heidelberg.
________________________________________________________________________________
Originalpublikation:
T. Schweigler, V. Kasper, S. Erne, I. Mazets, B. Rauer, F. Cataldini, T. Langen, T. Gasenzer, J. Berges, and J.
Schmiedmayer: Experimental characterization of a quantum many-body system via higher-order correlations,
Nature 545 (2017).
https://doi.org/10.1038/nature22310
________________________________________________________________________________
Prof. Dr. Jürgen Berges
Institut für Theoretische Physik
Telefon (06221) 54-9422
berges@thphys.uni-heidelberg.de
Kommunikation und Marketing
Pressestelle
Tel. +49 6221 54-2311
presse@rektorat.uni-heidelberg.de
Pressemitteilung Nr. 36/2017, 7. März 2017
Der Heidelberger Wissenschaftler Prof. Dr. Selim Jochim erhält eine hochdotierte Förderung des Europäischen Forschungsrats (ERC), einen ERC Consolidator Grant für exzellente junge Forscher. Unterstützt wird damit ein Forschungsprojekt im Bereich der Quantenphysik. Ziel ist es, aus wohlbekannten Bausteinen Vielteilchensysteme zusammenzusetzen und so die Eigenschaften von komplexen Quantensystemen zu simulieren. Dafür stellt der ERC für einen Zeitraum von fünf Jahren Fördermittel in Höhe von rund zwei Millionen Euro zur Verfügung. Prof. Jochim ist am Physikalischen Institut der Universität Heidelberg tätig. Der Physiker hatte bereits im Jahr 2011 einen ERC Starting Grant erhalten.
Nach den Worten von Prof. Jochim gehört es zu den zentralen Forschungsthemen in der Physik, das komplexe Zusammenspiel vieler Teilchen zu verstehen. Dabei geht es insbesondere um die Frage, wie sich daraus konkrete Eigenschaften der Materie ergeben. Ein besonders eingängiges Beispiel dafür ist die längst gelöste Frage, wie aus der mikroskopischen Bewegung der Gasmoleküle beobachtete Größen wie Druck oder Temperatur resultieren. In quantenmechanischen Vielteilchensystemen zielt diese Frage häufig auf die Bewegung von Elektronen in einem Festkörper ab, die hier mithilfe von neutralen Atomen simuliert werden. In ihren Experimenten arbeiten die Forscher um Selim Jochim mit ultrakalten Lithiumatomen. Dabei ist es ihnen bereits gelungen, die fundamentalen Bausteine zu erzeugen, die für die Simulation derartiger Vielteilchensysteme benötigt werden.
In dem vom ERC geförderten Projekt „Quantum State Assembler“ wollen die Heidelberger Wissenschaftler aus diesen Bausteinen Einheiten zusammensetzen, deren Eigenschaften kontrollierbar und steuerbar sind. Mithilfe dieser „Quantensimulation“ sollen schließlich physikalische Systeme untersucht werden, deren Eigenschaften sich ansonsten nur schwer vorhersagen lassen. „Damit erhoffen wir uns“, so Selim Jochim, „zum Verständnis noch ungeklärter Effekte, wie beispielsweise der Hochtemperatursupraleitung, beitragen zu können. Vielleicht lassen sich auf diesem Weg verwandte, aber dennoch ganz neue Phänomene entdecken.“
Selim Jochim (Jahrgang 1975) absolvierte ein Studium der Physik an der Universität Heidelberg, der University of California in Berkeley und der San Francisco State University. Mit einer Arbeit zur Bose-Einstein-Kondensation von Molekülen wurde er 2004 an der Universität Innsbruck (Österreich) promoviert. Als Postdoktorand forschte er am IBM Research Laboratory in Zürich (Schweiz) und an der University of Chicago (USA). Von 2006 bis 2009 war der Wissenschaftler in Kooperation mit der Universität Heidelberg als Juniorprofessor am Max-Planck-Institut für Kernphysik tätig. Seit 2009 hat Selim Jochim eine Professur für experimentelle Physik am Physikalischen Institut der Ruperto Carola inne.
Der Consolidator Grant wendet sich an vielversprechende Forscherinnen und Forscher, deren eigene unabhängige Arbeitsgruppe sich in der Festigungs- und Vertiefungsphase befindet. Zentrales Förderkriterium ist die wissenschaftliche Exzellenz.
________________________________________________________________________________
Prof. Dr. Selim Jochim
Physikalisches Institut / Zentrum für Quantendynamik
Telefon (06221) 54-19472
jochim@uni-heidelberg.de
Kommunikation und Marketing
Pressestelle
Tel. +49 6221 54-2311
presse@rektorat.uni-heidelberg.de
Pressemitteilung Nr. 36/2017, 7. März 2017
Der Heidelberger Wissenschaftler Prof. Dr. Selim Jochim erhält eine hochdotierte Förderung des Europäischen Forschungsrats (ERC), einen ERC Consolidator Grant für exzellente junge Forscher. Unterstützt wird damit ein Forschungsprojekt im Bereich der Quantenphysik. Ziel ist es, aus wohlbekannten Bausteinen Vielteilchensysteme zusammenzusetzen und so die Eigenschaften von komplexen Quantensystemen zu simulieren. Dafür stellt der ERC für einen Zeitraum von fünf Jahren Fördermittel in Höhe von rund zwei Millionen Euro zur Verfügung. Prof. Jochim ist am Physikalischen Institut der Universität Heidelberg tätig. Der Physiker hatte bereits im Jahr 2011 einen ERC Starting Grant erhalten.
Nach den Worten von Prof. Jochim gehört es zu den zentralen Forschungsthemen in der Physik, das komplexe Zusammenspiel vieler Teilchen zu verstehen. Dabei geht es insbesondere um die Frage, wie sich daraus konkrete Eigenschaften der Materie ergeben. Ein besonders eingängiges Beispiel dafür ist die längst gelöste Frage, wie aus der mikroskopischen Bewegung der Gasmoleküle beobachtete Größen wie Druck oder Temperatur resultieren. In quantenmechanischen Vielteilchensystemen zielt diese Frage häufig auf die Bewegung von Elektronen in einem Festkörper ab, die hier mithilfe von neutralen Atomen simuliert werden. In ihren Experimenten arbeiten die Forscher um Selim Jochim mit ultrakalten Lithiumatomen. Dabei ist es ihnen bereits gelungen, die fundamentalen Bausteine zu erzeugen, die für die Simulation derartiger Vielteilchensysteme benötigt werden.
In dem vom ERC geförderten Projekt „Quantum State Assembler“ wollen die Heidelberger Wissenschaftler aus diesen Bausteinen Einheiten zusammensetzen, deren Eigenschaften kontrollierbar und steuerbar sind. Mithilfe dieser „Quantensimulation“ sollen schließlich physikalische Systeme untersucht werden, deren Eigenschaften sich ansonsten nur schwer vorhersagen lassen. „Damit erhoffen wir uns“, so Selim Jochim, „zum Verständnis noch ungeklärter Effekte, wie beispielsweise der Hochtemperatursupraleitung, beitragen zu können. Vielleicht lassen sich auf diesem Weg verwandte, aber dennoch ganz neue Phänomene entdecken.“
Selim Jochim (Jahrgang 1975) absolvierte ein Studium der Physik an der Universität Heidelberg, der University of California in Berkeley und der San Francisco State University. Mit einer Arbeit zur Bose-Einstein-Kondensation von Molekülen wurde er 2004 an der Universität Innsbruck (Österreich) promoviert. Als Postdoktorand forschte er am IBM Research Laboratory in Zürich (Schweiz) und an der University of Chicago (USA). Von 2006 bis 2009 war der Wissenschaftler in Kooperation mit der Universität Heidelberg als Juniorprofessor am Max-Planck-Institut für Kernphysik tätig. Seit 2009 hat Selim Jochim eine Professur für experimentelle Physik am Physikalischen Institut der Ruperto Carola inne.
Der Consolidator Grant wendet sich an vielversprechende Forscherinnen und Forscher, deren eigene unabhängige Arbeitsgruppe sich in der Festigungs- und Vertiefungsphase befindet. Zentrales Förderkriterium ist die wissenschaftliche Exzellenz.
________________________________________________________________________________
Prof. Dr. Selim Jochim
Physikalisches Institut / Zentrum für Quantendynamik
Telefon (06221) 54-19472
jochim@uni-heidelberg.de
Kommunikation und Marketing
Pressestelle
Tel. +49 6221 54-2311
presse@rektorat.uni-heidelberg.de
Press Release No. 36/2017, 7 March 2017
Heidelberg researcher Prof. Dr Selim Jochim has received a prestigious ERC Consolidator Grant for excellent young researchers from the European Research Council (ERC). The funds will be used to support a research project in the field of quantum physics. The project’s aim is to assemble many-body systems from familiar components and thus simulate the properties of complex quantum systems. The ERC will provide approximately two million euros in funding for a five-year period. Prof. Jochim teaches at the Institute for Physics at Heidelberg University. In 2011, the physicist was the recipient of an ERC Starting Grant.
According to Prof. Jochim, one of the main areas of research in physics is understanding the complex interplay of many particles. Of particular interest is how microscopic interactions produce the specific properties of matter. A particularly simple example for this is the long-resolved question on how the motion of gas molecules leads to observed quantities like pressure and temperature. In quantum mechanical many body systems, this question is usually related to the motion of electrons in a solid, which is simulated here using neutral atoms. In their experiments, Jochim and his team of researchers are working with ultracold lithium atoms. They have already succeeded in creating the fundamental building blocks that are needed to simulate such many-body systems.
In the ERC-funded “Quantum State Assembler” project, the Heidelberg researchers intend to use these building blocks to assemble systems with widely tunable properties. Using this “quantum simulation”, they will investigate physical systems whose properties are otherwise very difficult to predict. “We hope to contribute to the understanding of effects that have yet to be explained, such as high-temperature superconductivity,” states Prof. Jochim. “We may also be able to discover related but completely new phenomena in this way.”
Selim Jochim (b. 1975) studied physics at Heidelberg University, the University of California in Berkeley and San Francisco State University. He earned his doctorate in 2004 at the University of Innsbruck, Austria for his dissertation on the Bose-Einstein condensate. He pursued postdoctoral research at the IBM Research Laboratory in Zürich, Switzerland and the University of Chicago in the US. In cooperation with Heidelberg University, the researcher was a Junior Professor at the Max Planck Institute for Nuclear Physics from 2006 to 2009. He has been a professor of experimental physics at the Institute for Physics at Ruperto Carola since 2009.
The Consolidator Grant is given to promising researchers whose own independent working group is in the consolidation phase. Scientific excellence is the main criterion.
________________________________________________________________________________
Prof. Dr. Selim Jochim
Institute for Physics/Center for Quantum Dynamics
Tel: (06221) 54-19472
jochim@uni-heidelberg.de
Communications and Marketing
Press Office
Phone +49 6221 54-2311
presse@rektorat.uni-heidelberg.de
Press Release No. 36/2017, 7 March 2017
Heidelberg researcher Prof. Dr Selim Jochim has received a prestigious ERC Consolidator Grant for excellent young researchers from the European Research Council (ERC). The funds will be used to support a research project in the field of quantum physics. The project’s aim is to assemble many-body systems from familiar components and thus simulate the properties of complex quantum systems. The ERC will provide approximately two million euros in funding for a five-year period. Prof. Jochim teaches at the Institute for Physics at Heidelberg University. In 2011, the physicist was the recipient of an ERC Starting Grant.
According to Prof. Jochim, one of the main areas of research in physics is understanding the complex interplay of many particles. Of particular interest is how microscopic interactions produce the specific properties of matter. A particularly simple example for this is the long-resolved question on how the motion of gas molecules leads to observed quantities like pressure and temperature. In quantum mechanical many body systems, this question is usually related to the motion of electrons in a solid, which is simulated here using neutral atoms. In their experiments, Jochim and his team of researchers are working with ultracold lithium atoms. They have already succeeded in creating the fundamental building blocks that are needed to simulate such many-body systems.
In the ERC-funded “Quantum State Assembler” project, the Heidelberg researchers intend to use these building blocks to assemble systems with widely tunable properties. Using this “quantum simulation”, they will investigate physical systems whose properties are otherwise very difficult to predict. “We hope to contribute to the understanding of effects that have yet to be explained, such as high-temperature superconductivity,” states Prof. Jochim. “We may also be able to discover related but completely new phenomena in this way.”
Selim Jochim (b. 1975) studied physics at Heidelberg University, the University of California in Berkeley and San Francisco State University. He earned his doctorate in 2004 at the University of Innsbruck, Austria for his dissertation on the Bose-Einstein condensate. He pursued postdoctoral research at the IBM Research Laboratory in Zürich, Switzerland and the University of Chicago in the US. In cooperation with Heidelberg University, the researcher was a Junior Professor at the Max Planck Institute for Nuclear Physics from 2006 to 2009. He has been a professor of experimental physics at the Institute for Physics at Ruperto Carola since 2009.
The Consolidator Grant is given to promising researchers whose own independent working group is in the consolidation phase. Scientific excellence is the main criterion.
________________________________________________________________________________
Prof. Dr. Selim Jochim
Institute for Physics/Center for Quantum Dynamics
Tel: (06221) 54-19472
jochim@uni-heidelberg.de
Communications and Marketing
Press Office
Phone +49 6221 54-2311
presse@rektorat.uni-heidelberg.de
Pressemitteilung Nr. 77/2016, 13. Juni 2016
Der Europäische Forschungsrat (ERC) vergibt eine hochdotierte Förderung – einen ERC Advanced Grant für Spitzenforscher in Europa – an den Heidelberger Physiker Prof. Dr. Markus Oberthaler. Über fünf Jahre wird damit ein Forschungsvorhaben an der Universität Heidelberg gefördert, in dem sich der Wissenschaftler mit der Erzeugung von quantenmechanischen Eigenschaften in komplexen Systemen beschäftigen wird. Im Mittelpunkt steht die Frage, ob es ein universelles Verhalten zur Erzeugung von quantenmechanischer Verschränkung in Vielteilchensystemen gibt. „Dies ist nicht nur von fundamentalem wissenschaftlichen Interesse, sondern hat direkte Auswirkungen auf die Sensorik der Zukunft, da auf diese Weise die Messung von physikalischen Größen erheblich besser durchgeführt werden könnte als mit den besten klassischen Messapparaturen“, sagt Prof. Oberthaler, der am Kirchhoff-Institut für Physik forscht und dem Zentrum für Quantendynamik angehört. Für die Forschungsarbeiten, die im Oktober 2016 beginnen, stehen rund 2,4 Millionen Euro an Fördermitteln zur Verfügung.
In dem vom ERC geförderten Projekt „Entanglement Generation in Universal Time Dynamics“ (EntangleGen) gehen Prof. Oberthaler und sein Team der grundlegenden Frage nach, wie sich in dynamischen Vielteilchensystemen quantenmechanische Korrelationen oder Verschränkungen aufbauen. Dieser zentrale Aspekt soll experimentell beleuchtet werden, indem mit modernen Methoden der Atomphysik derartige Systeme mit hoher Präzision sowohl produziert als auch analysiert werden. Wie der Wissenschaftler erläutert, ist eine Quantenverschränkung in Vielteilchensystemen, bei der sich mehrere Teilchen nicht mehr unabhängig voneinander, sondern nur noch in einem gemeinsamen Zustand beschreiben lassen, grundsätzlich schwer nachzuweisen. Im EntangleGen-Projekt wird eine neue Nachweismethode zum Einsatz kommen, die die Arbeitsgruppe unter der Leitung von Prof. Oberthaler vor zwei Jahren der Fachwelt vorgestellt hat. Der erfolgreiche Nachweis einer quantenmechanischen Verschränkung könnte die sogenannte Quantenmetrologie einen großen Schritt voranbringen, so der Heidelberger Physiker. In dem aktuellen Vorhaben sollen daher Fragestellungen der Grundlagenforschung und Fragen der Anwendung für eine bessere Sensorik miteinander verknüpft werden.
Markus Oberthaler (Jahrgang 1968) studierte Physik an der Universität Innsbruck (Österreich), an der er 1997 auch promoviert wurde. Nach einem zweijährigen Forschungsaufenthalt an der University of Oxford (Großbritannien) übernahm der Wissenschaftler im Jahr 2000 an der Universität Konstanz die Leitung einer eigenen Nachwuchsgruppe, die im Rahmen des Emmy-Noether-Programms von der Deutschen Forschungsgemeinschaft gefördert wurde. 2003 wechselte Markus Oberthaler als Professor für Experimentalphysik an die Universität Heidelberg. Am Kirchhoff-Institut für Physik leitet er die Arbeitsgruppe „Synthetische Quantensysteme“.
Der Europäische Forschungsrat vergibt den Advanced Grant an etablierte Spitzenforscher, die mit risikoreichen Forschungsvorhaben in ihren jeweiligen Bereichen neue Wege beschreiten.
________________________________________________________________________________
Prof. Dr. Markus Oberthaler
Kirchhoff-Institut für Physik
Tel. +49 6221 54-5170
markus.oberthaler@kip.uni-heidelberg.de
Kommunikation und Marketing
Pressestelle
Tel. +49 6221 54-2311
presse@rektorat.uni-heidelberg.de
Pressemitteilung Nr. 77/2016, 13. Juni 2016
Der Europäische Forschungsrat (ERC) vergibt eine hochdotierte Förderung – einen ERC Advanced Grant für Spitzenforscher in Europa – an den Heidelberger Physiker Prof. Dr. Markus Oberthaler. Über fünf Jahre wird damit ein Forschungsvorhaben an der Universität Heidelberg gefördert, in dem sich der Wissenschaftler mit der Erzeugung von quantenmechanischen Eigenschaften in komplexen Systemen beschäftigen wird. Im Mittelpunkt steht die Frage, ob es ein universelles Verhalten zur Erzeugung von quantenmechanischer Verschränkung in Vielteilchensystemen gibt. „Dies ist nicht nur von fundamentalem wissenschaftlichen Interesse, sondern hat direkte Auswirkungen auf die Sensorik der Zukunft, da auf diese Weise die Messung von physikalischen Größen erheblich besser durchgeführt werden könnte als mit den besten klassischen Messapparaturen“, sagt Prof. Oberthaler, der am Kirchhoff-Institut für Physik forscht und dem Zentrum für Quantendynamik angehört. Für die Forschungsarbeiten, die im Oktober 2016 beginnen, stehen rund 2,4 Millionen Euro an Fördermitteln zur Verfügung.
In dem vom ERC geförderten Projekt „Entanglement Generation in Universal Time Dynamics“ (EntangleGen) gehen Prof. Oberthaler und sein Team der grundlegenden Frage nach, wie sich in dynamischen Vielteilchensystemen quantenmechanische Korrelationen oder Verschränkungen aufbauen. Dieser zentrale Aspekt soll experimentell beleuchtet werden, indem mit modernen Methoden der Atomphysik derartige Systeme mit hoher Präzision sowohl produziert als auch analysiert werden. Wie der Wissenschaftler erläutert, ist eine Quantenverschränkung in Vielteilchensystemen, bei der sich mehrere Teilchen nicht mehr unabhängig voneinander, sondern nur noch in einem gemeinsamen Zustand beschreiben lassen, grundsätzlich schwer nachzuweisen. Im EntangleGen-Projekt wird eine neue Nachweismethode zum Einsatz kommen, die die Arbeitsgruppe unter der Leitung von Prof. Oberthaler vor zwei Jahren der Fachwelt vorgestellt hat. Der erfolgreiche Nachweis einer quantenmechanischen Verschränkung könnte die sogenannte Quantenmetrologie einen großen Schritt voranbringen, so der Heidelberger Physiker. In dem aktuellen Vorhaben sollen daher Fragestellungen der Grundlagenforschung und Fragen der Anwendung für eine bessere Sensorik miteinander verknüpft werden.
Markus Oberthaler (Jahrgang 1968) studierte Physik an der Universität Innsbruck (Österreich), an der er 1997 auch promoviert wurde. Nach einem zweijährigen Forschungsaufenthalt an der University of Oxford (Großbritannien) übernahm der Wissenschaftler im Jahr 2000 an der Universität Konstanz die Leitung einer eigenen Nachwuchsgruppe, die im Rahmen des Emmy-Noether-Programms von der Deutschen Forschungsgemeinschaft gefördert wurde. 2003 wechselte Markus Oberthaler als Professor für Experimentalphysik an die Universität Heidelberg. Am Kirchhoff-Institut für Physik leitet er die Arbeitsgruppe „Synthetische Quantensysteme“.
Der Europäische Forschungsrat vergibt den Advanced Grant an etablierte Spitzenforscher, die mit risikoreichen Forschungsvorhaben in ihren jeweiligen Bereichen neue Wege beschreiten.
________________________________________________________________________________
Prof. Dr. Markus Oberthaler
Kirchhoff-Institut für Physik
Tel. +49 6221 54-5170
markus.oberthaler@kip.uni-heidelberg.de
Kommunikation und Marketing
Pressestelle
Tel. +49 6221 54-2311
presse@rektorat.uni-heidelberg.de
Press Release No. 77/2016, 13 June 2016
The European Research Council (ERC) has awarded a highly endowed grant – an ERC Advanced Grant for leading researchers in Europe – to Heidelberg physicist Prof. Dr Markus Oberthaler. The five-year endowment will fund a Heidelberg University research project to explore the generation of quantum mechanical properties in complex systems. Of particular interest is whether there is universal behaviour to generate quantum mechanical entanglement in many-body systems. “This is not only of fundamental scientific interest but also has direct impact on the sensor technology of the future because this will allow physical quantities to be measured with far more accuracy than with the best traditional measuring devices,” says Prof. Oberthaler, a researcher at the Kirchhoff Institute for Physics and a member of the Center for Quantum Dynamics. 2.4 million euros have been allocated to the project, set to begin in October 2016.
In the ERC-funded project, entitled “Entanglement Generation in Universal Time Dynamics” (EntangleGen), Prof. Oberthaler and his team will investigate the fundamental question of how quantum mechanical correlations known as entanglement form in dynamical evolving many-body systems. In order to clarify this question experimentally, the researchers will apply modern methods of atomic physics to produce and analyse these types of systems with unprecedented precision. Prof. Oberthaler points out that it is extremely difficult to detect quantum entanglement in many-body systems in which multiple particles can only be described as a quantum mechanical unit in their aggregate state rather than independently of one another. The EntangleGen project builds on a new detection method that the research group led by Prof. Oberthaler introduced to the research community two years ago. According to the Heidelberg physicist, successfully detecting a quantum mechanical entanglement could greatly advance the field of quantum metrology. In the current project, issues of basic research will therefore be combined with application aspects for tomorrow’s sensor technology.
Markus Oberthaler (born 1968) studied physics at the University of Innsbruck (Austria), earning his doctorate in 1997. After a two-year research stay at the University of Oxford (Great Britain), in 2000 he became head of an independent junior research group at the University of Konstanz funded through the prestigious Emmy Noether Programme of the German Research Foundation. In 2003 Markus Oberthaler joined the faculty of Heidelberg University as a professor of experimental physics. He directs the Synthetic Quantum Systems Research Group at the Kirchhoff Institute for Physics.
The European Research Council awards the Advanced Grant to senior researchers pursuing high-risk, ground-breaking research in their respective fields.
________________________________________________________________________________
Prof. Dr. Markus Oberthaler
Kirchhoff Institute for Physics
Phone + 49 6221 54-5170
markus.oberthaler@kip.uni-heidelberg.de
Communications and Marketing
Press Office
Phone +49 6221 54-2311
presse@rektorat.uni-heidelberg.de
Press Release No. 77/2016, 13 June 2016
The European Research Council (ERC) has awarded a highly endowed grant – an ERC Advanced Grant for leading researchers in Europe – to Heidelberg physicist Prof. Dr Markus Oberthaler. The five-year endowment will fund a Heidelberg University research project to explore the generation of quantum mechanical properties in complex systems. Of particular interest is whether there is universal behaviour to generate quantum mechanical entanglement in many-body systems. “This is not only of fundamental scientific interest but also has direct impact on the sensor technology of the future because this will allow physical quantities to be measured with far more accuracy than with the best traditional measuring devices,” says Prof. Oberthaler, a researcher at the Kirchhoff Institute for Physics and a member of the Center for Quantum Dynamics. 2.4 million euros have been allocated to the project, set to begin in October 2016.
In the ERC-funded project, entitled “Entanglement Generation in Universal Time Dynamics” (EntangleGen), Prof. Oberthaler and his team will investigate the fundamental question of how quantum mechanical correlations known as entanglement form in dynamical evolving many-body systems. In order to clarify this question experimentally, the researchers will apply modern methods of atomic physics to produce and analyse these types of systems with unprecedented precision. Prof. Oberthaler points out that it is extremely difficult to detect quantum entanglement in many-body systems in which multiple particles can only be described as a quantum mechanical unit in their aggregate state rather than independently of one another. The EntangleGen project builds on a new detection method that the research group led by Prof. Oberthaler introduced to the research community two years ago. According to the Heidelberg physicist, successfully detecting a quantum mechanical entanglement could greatly advance the field of quantum metrology. In the current project, issues of basic research will therefore be combined with application aspects for tomorrow’s sensor technology.
Markus Oberthaler (born 1968) studied physics at the University of Innsbruck (Austria), earning his doctorate in 1997. After a two-year research stay at the University of Oxford (Great Britain), in 2000 he became head of an independent junior research group at the University of Konstanz funded through the prestigious Emmy Noether Programme of the German Research Foundation. In 2003 Markus Oberthaler joined the faculty of Heidelberg University as a professor of experimental physics. He directs the Synthetic Quantum Systems Research Group at the Kirchhoff Institute for Physics.
The European Research Council awards the Advanced Grant to senior researchers pursuing high-risk, ground-breaking research in their respective fields.
________________________________________________________________________________
Prof. Dr. Markus Oberthaler
Kirchhoff Institute for Physics
Phone + 49 6221 54-5170
markus.oberthaler@kip.uni-heidelberg.de
Communications and Marketing
Press Office
Phone +49 6221 54-2311
presse@rektorat.uni-heidelberg.de
Pressemitteilung Nr. 66/2016, 27. Mai 2016
Mit vier Anträgen für die Förderung großer Forschungsverbünde ist die Universität Heidelberg in der aktuellen Bewilligungsrunde der Deutschen Forschungsgemeinschaft (DFG) erfolgreich: So fördert die DFG einen neuen Sonderforschungsbereich/Transregio (SFB/TRR), der an der Medizinischen Fakultät Heidelberg angesiedelt ist: Hier werden sich Wissenschaftler aus Heidelberg, Freiburg und München mit Infektionen durch Hepatitis-Viren befassen. Hinzu kommt ein weiterer neu bewilligter SFB/TRR auf dem Gebiet der molekularen Lebenswissenschaften; Heidelberger und Berliner Wissenschaftler untersuchen in diesem Verbund die Koordination der Signalübermittlung in lebenden Zellen. Neu eingerichtet wird außerdem ein SFB in der Physik, an dem isolierte Quantensysteme unter extremen Bedingungen in Experiment und Theorie erforscht werden. In eine zweite Förderperiode geht der molekularbiologische SFB 1036 „Zelluläre Qualitätskontrolle und Schadensbegrenzung“ der Universität Heidelberg. Für alle vier Verbünde, die jeweils über vier Jahre gefördert werden, stellt die DFG Fördermittel in Höhe von insgesamt mehr als 46 Millionen Euro zur Verfügung.
SFB/TRR 179 „Ursachen der Ausheilung oder Chronifizierung von Infektionen mit Hepatitis-Viren“
Die Wissenschaftler im Sonderforschungsbereich/Transregio 179 gehen am Beispiel der Infektion mit verschiedenen Hepatitis-Viren der Frage nach, welche Faktoren den Ausschlag dafür geben, dass Infektionen ausheilen oder einen chronischen Verlauf nehmen. Wie Prof. Dr. Ralf Bartenschlager erläutert, unterscheiden sich die Vermehrungsstrategien beispielsweise der Hepatitis-B-Viren und der Hepatitis-C-Viren grundsätzlich. „Darüber ist inzwischen viel bekannt. Wir wissen allerdings nicht, wie es die Viren jeweils schaffen, die körpereigene Abwehr zu überlisten“, sagt der Sprecher des neuen SFB/TRR „Ursachen der Ausheilung oder Chronifizierung von Infektionen mit Hepatitis-Viren“. Nach den Worten von Prof. Bartenschlager hat sich die Forschung bislang hauptsächlich darauf konzentriert, einzelne Signalwege zu entschlüsseln oder wichtige molekulare Faktoren zu identifizieren. „Wenn wir allerdings die generellen Mechanismen verstehen wollen, die darüber entscheiden, ob eine Virus-Infektion chronisch wird oder spontan ausheilt, brauchen wir einen integrativen Ansatz, der die komplexen Wechselwirkungen zwischen Viren und Wirtsorganismus von verschiedenen Seiten beleuchtet“, so der Virologe, der Direktor der Abteilung für Molekulare Virologie am Zentrum für Infektiologie des Universitätsklinikums Heidelberg ist und den Forschungsschwerpunkt „Infektionen, Entzündung und Krebs“ am Deutschen Krebsforschungszentrum (DKFZ) leitet. Neben weiteren Wissenschaftlern der Universität Heidelberg sind Teams des Universitätsklinikums Freiburg, der Technischen Universität München, der Ludwig-Maximilians-Universität München sowie des DKFZ an den insgesamt 21 Teilprojekten beteiligt. Der SFB/TRR 179 erhält Fördermittel in Höhe von rund 12,1 Millionen Euro.
SFB/TRR 186 „Molekulare Schalter in der Raum-Zeit-Kontrolle der zellulären Signaltransmission“
Zentrales Forschungsthema des SFB/TRR 186 „Molekulare Schalter in der Raum-Zeit-Kontrolle der zellulären Signaltransmission“ sind die Koordinierungsprozesse bei der Übermittlung von Signalen in lebenden Zellen, die in der Funktionalität von biologischen Systemen eine zentrale Rolle spielen. Weitgehend unbekannt ist dabei aber, wie die Signale von aktivierten molekularen Schaltern räumlich und zeitlich koordiniert werden, wie Prof. Dr. Walter Nickel vom Biochemie-Zentrum der Universität Heidelberg erläutert. Der neue Forschungsverbund basiert auf der Entwicklung einer großen Zahl von chemisch-biologischen Werkzeugen, die auf breiter Basis für eine direkte „Manipulation“ von molekularen Schaltern angewendet werden können. Mit Hilfe der extrem hochauflösenden Lichtmikroskopie sollen diese Techniken für systematische Untersuchungen in lebenden Zellen eingesetzt werden. Aufbauend darauf wollen die Wissenschaftler theoretische Modelle zur Aufklärung der räumlichen und zeitlichen Regulation zellulärer Signalübertragungsprozesse entwickeln, so Prof. Nickel, der die Sprecherfunktion des Konsortiums übernimmt. Der SFB/TRR wird 18 wissenschaftliche Teilprojekte und zwei technische Plattformen umfassen. Beteiligt sind daran neben der Universität Heidelberg, der Freien Universität Berlin und der Humboldt-Universität zu Berlin auch Forscher des Universitätsklinikums Heidelberg und der Charité – Universitätsmedizin Berlin. Als außeruniversitäre Einrichtungen werden das Deutsche Krebsforschungszentrum (DKFZ) und das Europäische Laboratorium für Molekularbiologie (EMBL) in Heidelberg sowie das Leibniz-Institut für Molekulare Pharmakologie in Berlin in diesem Verbund mitwirken. Der SFB/TRR 186 erhält DFG-Fördermittel in Höhe von rund 11,8 Millionen Euro.
SFB 1225 „Isolierte Quantensysteme und Universalität unter extremen Bedingungen“ (ISOQUANT)
Im Mittelpunkt des SFB 1225 „Isolierte Quantensysteme und Universalität unter extremen Bedingungen“ (ISOQUANT) steht ein Forschungsthema, das für eine Vielzahl von Anwendungen – von der Teilchenphysik über die Kernphysik bis zur Atom- und Festkörperphysik – von großer Bedeutung ist. Viele dieser Systeme zeigen ähnliche Eigenschaften, obwohl wesentliche Parameter wie Temperatur, Dichte oder Feldstärke sehr verschieden sind. Es existieren sogar universelle Bereiche, in denen quantitative Übereinstimmungen zwischen scheinbar grundverschiedenen physikalischen Systemen beobachtet werden können. Die am Sonderforschungsbereich beteiligten Wissenschaftler werden dabei sowohl zeitabhängige Phänomene als auch Gleichgewichtseigenschaften mit gemeinsamen Herangehensweisen untersuchen, um mit diesem neuen Ansatz aktuelle Forschungsfragen fachübergreifend bearbeiten zu können. Neben dem sogenannten Thermalisierungsprozess isolierter Quantensysteme geht es dabei um das Wechselspiel zwischen starken elektromagnetischen oder stark wechselwirkenden Feldern und dem Vakuum oder Materie sowie um die Phasenstruktur von Systemen unter extremen Bedingungen. Im Sonderforschungsbereich ISOQUANT haben sich Arbeitsgruppen des Instituts für Theoretische Physik, des Kirchhoff-Instituts für Physik und des Physikalischen Instituts der Universität Heidelberg sowie Wissenschaftler des Max-Planck-Instituts für Kernphysik in Heidelberg und der Technischen Universität Wien (Österreich) zusammengeschlossen. Die Funktion des Sprechers übernimmt Prof. Dr. Jürgen Berges, der an der Ruperto Carola auf dem Gebiet der Theoretischen Physik forscht. Die DFG fördert den SFB, der 14 Teilprojekte umfasst, mit rund 10,5 Millionen Euro.
SFB 1036 „Zelluläre Qualitätskontrolle und Schadensbegrenzung“
Im Mittelpunkt des SFB 1036 stehen die Überwachungs- und Qualitätskontrollsysteme, die Zellen vor Schäden oder einer Störung des zellulären Gleichgewichts bewahren. Diese Systeme registrieren Defekte und lösen schützende Stressantworten aus, die das Überleben der Zellen ermöglichen sollen. Die Wissenschaftler des Sonderforschungsbereichs, der am Zentrum für Molekulare Biologie der Universität Heidelberg (ZMBH) angesiedelt ist, untersuchen diese Prozesse auf molekularer und systemischer Ebene. Im Mittelpunkt stehen dabei die Überwachungssysteme der DNA, RNA und Proteine, die anhand verschiedener Modellorganismen erforscht werden. In den kommenden vier Jahren sollen die Arbeiten durch eine strukturbiologische Analyse der Kontrollmechanismen von Proteinen auf atomarer Ebene erweitert werden. Weitere zentrale Aspekte sind die Qualitätskontrolle an der Schnittstelle von Proteinüberwachung und Proteinsynthese sowie die Sicherung der Genomstabilität. Darüber hinaus wird es um Modelle für Stressantworten in multizellulären Organismen gehen. „Unser langfristiges Ziel ist es, ein übergreifendes, mechanistisches Verständnis der zellulären Überwachungssysteme und Schadensantworten für alle Organismen zu entwickeln“, betont ZMBH-Direktor Prof. Dr. Bernd Bukau, der Sprecher des SFB 1036 „Zelluläre Qualitätskontrolle und Schadensbegrenzung“ ist. An den 20 Teilprojekten des Sonderforschungsbereichs wirken neben Biowissenschaftlern und Medizinern der Ruperto Carola auch Wissenschaftler des Deutschen Krebsforschungszentrums (DKFZ) sowie des Europäischen Laboratoriums für Molekularbiologie (EMBL) in Heidelberg mit. Die zentrale Koordination liegt am ZMBH. Prof. Bukau leitet eine Brückenabteilung am DKFZ und ist Ko‐Direktor der DKFZ‐ZMBH‐Allianz. In der zweiten Förderperiode wird der SFB 1036 von der DFG mit rund 12,1 Millionen Euro gefördert.
________________________________________________________________________________
Kommunikation und Marketing
Pressestelle
Tel. +49 6221 54-2311
presse@rektorat.uni-heidelberg.de
Press Release No. 66/2016, 27 May 2016
Heidelberg University succeeded in gaining support from the German Research Foundation (DFG) in the latest approval round, recieving funding for four research networks. The DFG granted funds to the new Transregional Collaborative Research Centre (CRC/TRR) of the Heidelberg Medical Faculty, where researchers from Heidelberg, Freiburg and Munich will investigate viral hepatitis infections. In the molecular life sciences, Heidelberg and Berlin scientists in another new CRC/TRR will study the coordination of signal transmission in living cells. A new CRC, this one in the field of physics, will explore in experiments and theory isolated quantum systems under extreme conditions. In molecular biology, the “Cellular Surveillance and Damage Response” CRC 1036 has been approved for a second funding period. Total DFG funding for all four consortia is over 46 million euros for a period of four years.
CRC/TRR 179, “Determinants and Dynamics of Elimination versus Persistence of Hepatitis Virus Infection”
Researchers in Transregional Collaborative Research Centre 179 will study infections caused by various hepatitis viruses to determine the critical factors in healing or a chronic disease course. As Prof. Dr. Ralf Bartenschlager explains, the reproduction strategies of the hepatitis B and hepatitis C viruses, for example, fundamentally differ. “Although we do know a lot, what we don’t know is how the viruses manage to outsmart the body’s defences,” says the spokesperson for the new CRC/TRR, “Determinants and Dynamics of Elimination versus Persistence of Hepatitis Virus Infection”. Until now, research concentrated mainly on decoding individual signal pathways or identifying critical molecular factors. “But if we want to understand the general mechanisms that determine whether a viral infection becomes chronic or spontaneously heals, we need an integrative approach that examines the complex interactions between viruses and the host organism from different sides,” says the virologist, who is the Director of the Molecular Virology Department at the Centre for Infectious Diseases of Heidelberg University Hospital and heads the “Infection, Inflammation and Cancer” Department at the German Cancer Research Center (DKFZ). In addition to researchers from Heidelberg University, teams from the Freiburg University Hospital, the Technical University of Munich, the Ludwig Maximilians University of Munich and the DKFZ will be involved in the 21 total subprojects. CRC/TRR 179 will receive funding in the amount of approx. 12.1 million euros.
CRC/TRR 186, “Molecular Switches in the Spacio-temporal Control of Cellular Signal Transmission”
The research of CRC/TRR 186, “Molecular Switches in the Spacio-temporal Control of Cellular Signal Transmission”, will focus on the coordination processes in the transmission of signals in living cells that play a pivotal role in the functionality of biological systems. According to Prof. Dr. Walter Nickel of the Heidelberg University Biochemistry Center, how activated molecular switches coordinate the signals in time and space remains largely unknown. The new research network is based on the development of a large number of chemical-biological tools that can be used for broad-based, direct manipulation of molecular switches. Using extremely high-resolution light microscopy, these technologies are to be used for systematic studies in living cells. On that basis, the researchers plan on developing theoretical models to explain the spatial and temporal regulation of cellular signal transmission processes, according to Prof. Nickel, spokesperson for the consortium. The CRC/TRR will incorporate 18 scientific subprojects and two technical platforms. In addition to Heidelberg University, other participating universities include Berlin’s Free University and Humboldt University along with researchers from Heidelberg University Hospital and Charité – University Hospital Berlin. Collaborating non-university institutions include the German Cancer Research Center (DKFZ), the European Laboratory for Molecular Biology (EMBL) in Heidelberg and the Leibniz Institute for Molecular Pharmacology in Berlin. CRC/TRR 186 will receive DFG funding in the amount of approx. 11.8 million euros.
CRC 1225, “Isolated Quantum Systems and Universality under Extreme Conditions” (ISOQUANT)
The “Isolated Quantum Systems and Universality under Extreme Conditions” (ISOQUANT) CRC is focused on a research area of major importance for numerous applications – from particle physics and nuclear physics to atomic and solid state physics. Many of these systems evidence similar properties even though essential parameters such as temperature, density and field strength are markedly different. There are even universal areas in which quantitative equivalents between seemingly completely different physical systems can be observed. CRC 1225 researchers will study time-dependent phenomena as well as equilibrium properties using common approaches to support cross-discipline investigation of current questions in research. Areas under study include the thermalisation process of isolated quantum systems, the interaction of highly electromagnetic or interactive fields with a vacuum or matter, and the phase structure of systems under extreme conditions. Members of the ISOQUANT CRC include working groups from the Institute for Theoretical Physics, the Kirchhoff Institute for Physics and the Institute for Physics of Heidelberg University as well as researchers from the Max Planck Institute for Nuclear Physics in Heidelberg and the Vienna University of Technology in Austria. Theoretical physicist Prof. Dr. Jürgen Berges of Ruperto Carola will serve as the CRC spokesperson. The CRC, which comprises 14 subprojects, will receive DFG funding in the amount of approx. 10.5 million euros.
CRC 1036, “Cellular Surveillance and Damage Response”
CRC 1036 is devoted to the study of surveillance and quality control systems that safeguard cells from damage or disruption of cellular equilibrium. These systems register defects and release protective stress responses intended to support the survival of the cell. The investigators of the Collaborative Research Centre, which is located at the Centre for Molecular Biology of Heidelberg University (ZMBH), are examining these processes at the molecular and systemic level. Of particular interest are the surveillance systems of DNA, RNA and proteins, which are being investigated based on different model organisms. Over the next four years, the CRC will expand its research to include the structural biological analysis of the control mechanisms of proteins at the atomic level. Other central topics include quality control at the interface of protein surveillance and protein synthesis and safeguarding genome stability. Models for stress responses in multicellular organisms are also being studied. “Our long-term goal is to develop a comprehensive mechanistic understanding of the cellular surveillance systems and damage responses for all organisms,” emphasises ZMBH Director Prof. Dr. Bernd Bukau, spokesperson for CRC 1036, “Cellular Surveillance and Damage Response”. In addition to bioscientists and medical scientists at Ruperto Carola, researchers from the German Cancer Research Center (DKFZ) and the European Laboratory for Molecular Biology (EMBL) in Heidelberg are also involved in the CRC’s 20 subprojects. The ZMBH is providing central coordination. Prof. Bukau heads a cross-departmental group at the DKFZ and Co‐director of the DKFZ‐ZMBH Alliance. In the second funding period, CRC 1036 will receive approx. 12.1 million euros in funding from the DFG.
________________________________________________________________________________
Communications and Marketing
Press Office
Phone +49 6221 54-2311
presse@rektorat.uni-heidelberg.de